Stimulation of Na+-Ca2+ exchange by purified antibody against alpha-2 repeat of Na+-Ca2+ exchanger in rat cardiomyocytes
Introduction
The process of Na+-Ca2+ exchange was first identified in guinea pig atria by Reuter and Seitz in 1968[1], and in the squid giant axon by Baker et al shortly after in 1969[2]. The entity, the so-called Na+-Ca2+ exchanger (NCX), is an ion transport protein that catalyzes electrogenetic antitransport of Na+ and Ca2+ across the plasma membrane in a coupling ratio of 3 Na+:1 Ca2+ and exists in the plasma membrane of almost all animal cells[3]. It is in cardiomyocytes, however, that the exchanger is highly expressed and plays an important role in Ca2+ homeostasis. The NCX system is the primary mechanism responsible for transarcolemmal Ca2+ extrusion. There is a general agreement that the majority of Ca2+ entry through voltage-dependent Ca2+ channel is transported out of the cell by NCX[4,5]. Moreover, it has also been suggested that Ca2+ entry mediated by NCX in Ca2+ influx mode contributed directly to contraction of failing human ventricular myocytes during the early period of the cardiomyocyte action potential[6].
The study of NCX in molecular biology started after the cloning of canine cardiac NCX by Nicoll and Philipson[7]. Later in 1997, Schwarz and Benzer[8] first identified the highly conserved regions in all known members of the NCX family, designated the alpha-1 and alpha-2 repeats. These regions are highly conserved among different exchangers and between one another. In cardiac NCX, the alpha-1 repeat comprises most of the putative transmembrane segment 2 and 3 (TM2 and TM3) and their connecting loop, whereas alpha-2 locates in putative TM7 and its C-terminal sequence[9].
A recent study by Nicoll and Iwamoto demonstrated that the NCX1 had oppositely oriented reentrant loop domains in alpha-1 and alpha-2 repeats, and that these reentrant domains in the alpha-repeats might be involved in the formation of the ion transport pathway[9]. Mutation analysis also showed that alpha-repeats were involved in the interaction of the exchanger with transport substrates (Na+ and Ca2+), Ni2+, Li+ and KB-R7943[10–12].
Now that the alpha-repeats regions were considered important in the ion binding and translocation, it is possible that the antibodies against alpha-1 repeat and alpha-2 repeat may have a crucial action on Na+-Ca2+ exchanger activity. However, the effect of the antibody against alpha-2 repeat on INCX and its specificity is unclear until now. The goal of this study is to identify the effects of the antibody on Na+-Ca2+ exchanger current using a whole-cell patch-clamp technique. Furthermore, the functional specificity of the antibody was also investigated in adult rat cardiomyocytes.
Materials and methods
Ventricular myocyte isolation Single ventricular myocytes were isolated from Wistar rats (250–300 g) using an enzymatic dissociation procedure similar to that described by Mubagwa et al[13]. In brief, rats were anesthetized with sodium pentobarbital (30 mg/kg, ip) 30 min after having received heparin (500 U, ip). The heart was quickly removed, rinsed in ice-cold Ca2+ free Tyrode’s solution and perfused with oxygenated Ca2+ free Tyrode’s solution (at 37 °C) via aorta for approximately 7–8 min to wash out the blood. The composition of Tyrode’s solution was (in mmol/L): NaCl 135, KCl 5.4, CaCl2 1.8, MgCl2 1.0, NaH2PO4 0.33, HEPES (4-(2-hydroxyethyl)piperazine-1-ethanesulfonic acid) 10, glucose 10 (pH adjusted to 7.4 with NaOH). The composition of Ca2+-free Tyrode’s solution was the same to Tyrode’s solution except for the absence of CaCl2. Then the perfusate was switched to enzyme solution for 8–10 min. Enzyme solution contained (in mmol/L): NaCl 135, KCl 5.4, CaCl2 75 µmol/L, MgCl2 1.0, NaH2PO4 0.33, HEPES 10, glucose 10, taurine 20, collagenase P (Boehringer Mannheim, Mannheim, Germany) 100 mg/L (pH adjusted to 7.4 with NaOH). The ventricle was then separated and minced with a pair of surgical scissors in the Kraftbrühe (KB) solution. The isolated myocytes were stored in KB solution at room temperature (22 °C) at least 4 h before use. KB solution was composed of (in mmol/L): KOH 85, L-glutamic acid 50, KCl 30, MgCl2 1.0, KH2PO4 30, glucose 10, taurine 20, HEPES 10, EGTA[ethyleneglycol-bis(β-amino-ethylether)-N,N,N´,N´-tetraacetic acid] 0.5 (pH adjusted to 7.4 with KOH 1 mol/L).
Electrophysiological measurement Voltage-clamp recording was carried out in the whole-cell configuration of the patch-clamp method[14] using a Patchclamp Amplifier (Axopatch-200A, Axon Instruments, Foster City, CA, USA). Patch electrodes were made from thin-walled glass capillaries and the electrodes with resistance of 2–4 MΩ were filled with the pipette solution. Cell capacitance was measured by the method described by Coetzee et al[15]. Analysis was carried out using pClampfit 8.0 software (Axon Instruments).
For the measurement of Na+-Ca2+ exchange current (INa/Ca), the extracellular (test) solution contained (in mmol/L): NaCl 140, CaCl2 2.0, MgCl2 2.0, glucose 10, HEPES 5.0 (pH adjusted to 7.4 with CsOH). In addition, the Na+-K+ pump, K+ channel and Ca2+ channel were blocked with ouabain (Sigma Chemical, St Louis, MO, USA) 20 µmol/L, BaCl2 1.0 mmol/L, CsCl 2.0 mmol/L and nicardipine (Sigma) 1.0 µmol/L. The pipette solution contained (in mmol/L): EGTA 42, CaCl2 29, MgCl2 13, aspartate, K2ATP 10, Na2-cretinephosphate 5.0, TEA (tetraethylammonium) (Sigma) 20, HEPES 5.0 (pH adjusted to 7.4 with CsOH). To measure L-type Ca2+ current (ICa,L), the extracellular (test) solution contained (in mmol/L): NaCl 135, CaCl2 1.8, MgCl2 1.0, KCl 5.4, glucose 10, NaH2PO4 0.33, HEPES 10 (pH adjusted to 7.4 with NaOH). The pipette solution contained (in mmol/L): EGTA 10, KCl 140, Na2ATP 2.0, HEPES 5.0, 4-AP 5.0, MgCl2 1.0 (pH adjusted to 7.3 with KOH). To record voltage-gated Na+ current (INa), the extracellular (test) solution contained (in mmol/L): NaCl 60, CsCl 5.0, CdCl2 0.1, MgCl2 2.5, glucose 10, 4-AP 5.0, HEPES 5.0, saccharose 80 (pH adjusted to 7.4 with NaOH). The pipette solution contained (in mmol/L): EGTA 11, KCl 130, Na2ATP 5.0, HEPES 10, MgCl2 2.0, CaCl2 1.0, 4-AP 5.0 (pH adjusted to 7.2 with CsOH). For the measurement of delayed rectifier K+ current (IK), the extracellular (test) solution contained (in mmol/L): NaCl 145, KCl 4.0, MgCl2 1.0, HEPES 10, glucose 5.0, CaCl2 0.l, CdC12 0.1 (pH adjusted to 7.4 with NaOH). The pipette solution contained (in mmol/L): KCl 130, MgCl2 2.0, CaCl2 1.0, EGTA 11, MgATP 5, K2ATP 5.0, HEPES 10 (pH adjusted to 7.4 with KOH).
Antibody preparation The antibody against alpha-2 repeat of Na+-Ca2+ exchanger was prepared in our laboratory[16]. Briefly, peptide corresponding to alpha-2 repeat (815 TFASKVAATQDQYADASIGNVTGSN 839) in cardiac NCX was synthesized by CL (Xi’an) Bioscientific Incorporation. Then the rats were randomly divided into two groups: control and immunized groups. Rats in immunized groups were immunized with the synthesized alpha-2 repeat emulsified in equal volume of Freund’s adjuvant (CFA, Sigma). The positive antiserum with high titer (≥1:640 by enzyme-linked immunosorbent assay [ELISA]) were affinity-purified using a Mab Trap Kit (Amersham Pharmacia Biotech, Uppsala, Sweden). The concentration of the purified antibody was determined using the method described by Bradford[17]. The control group received the same disposal as described above except that the peptide was substituted for saline solution.
Data analysis Results were expressed as mean±SD, and analyzed with least-significant difference (LSD) test of ANOVA in SPSS 11. P<0.05 was considered significant. EC50 values were determined using GraphPad Prism 4 software.
Results
Measurement of the Na+-Ca2+ exchange current (INa/Ca) INa/Ca was measured as the current sensitive to 5.0 mmol/L Ni2+[18] by the voltage protocol shown at the top of Figure 1. Ramp voltage-clamp pulse from 60 to –120 mV (90 mV/s) was applied from a holding potential of –40 mV. The current-voltage relationship was constructed from the declining slope of the ramp pulse. After application of Ni2+ at the concentration of 5.0 mmol/L, the current immediately decreased, at both positive and negative potentials (Figure 1). The difference between current–voltage relationships in the absence and presence of Ni2+ reflected INa/Ca (Ni2+-sensitive current). Significant run-down of the Ni2+-sensitive current was not observed during the experiment.
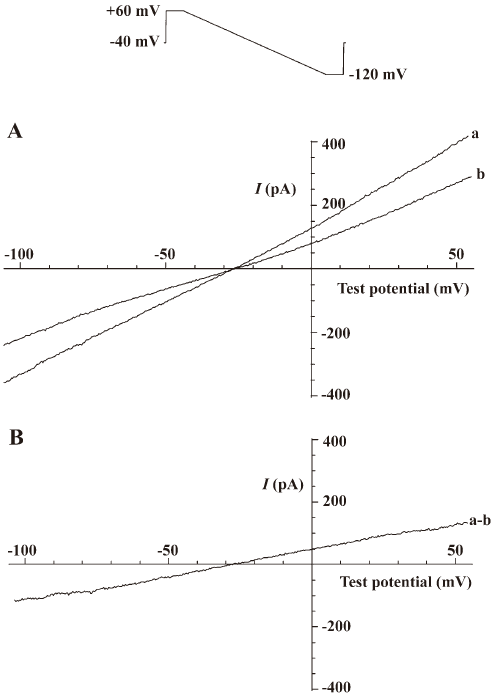
Stimulating effect of antibody against alpha-2 repeat on Na+-Ca2+ exchange current (INa/Ca) Antibody against alpha-2 repeat had a stimulating effect on performance of Na+-Ca2+ exchange, as demonstrated by the present study. It was shown that this antibody increased both the outward current and inward current concentration-dependently with EC50 values of 27.9 nmol/L and 24.7 nmol/L, respectively (Figure 2, Table 1). The stimulating effects on both outward and inward current of INa/Ca were abolished when the antibody was incubated with synthesized alpha-2 repeat before it was applied to cells.
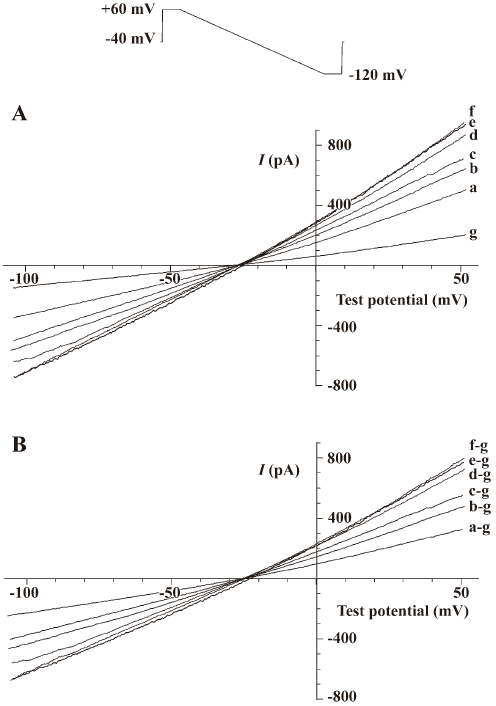
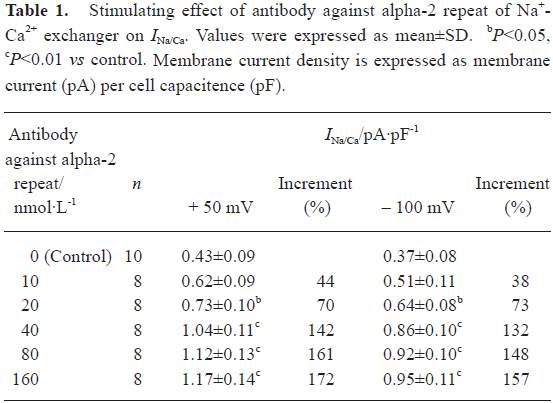
Full table
Effects of the antibody against alpha-2 repeat on ICa, L, INa and IK To evaluate the functional selectivity of the antibody against alpha-2 repeat, its effects on ICa,L, INa in adult rat hearts and IK in adult guinea-pig hearts were also observed. It was shown that this antibody could also increase ICa,L in a concentration-dependent manner and EC50 was 33.6 nmol/L. After washing with Tyrode solution, the effect could be partly abolished. Nicardipine could inhibit the above inward current completely, which proved that the current was ICa,L (Figure 3, Table 2). Furthermore, the current-voltage (I–V) relationship curve did not shift after application of the 40 nmol/L antibody, although peak Ca2+ current increased at +10 mV (Figure 4). Effects of the antibody on INa (Figure 5) and IK (Figure 6) were not observed in the present study.
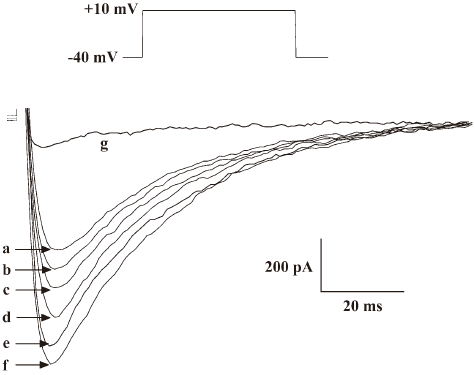
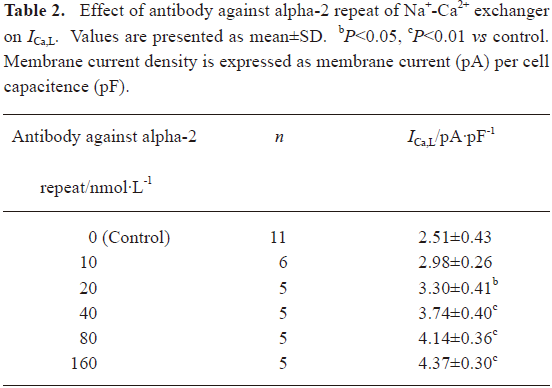
Full table
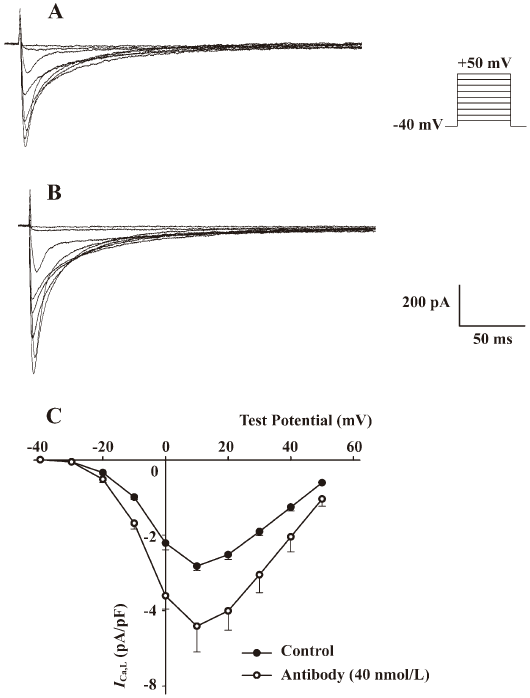
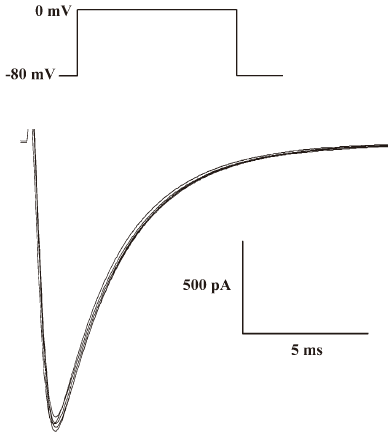
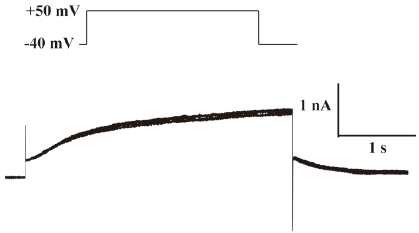
Discussion
Under physiological conditions, NCX operates mainly in Ca2+ efflux mode (Na+ influx), and only a very small quantity of Ca2+ enters the cell during the very early rising phase(1–4 ms) of the action potential via NCX in Ca2+ influx mode[19]. This is not the case, however, in pathological conditions such as heart failure (HF). It was shown that NCX could bring a larger amount of Ca2+ into the cell in HF than in normal conditions during the action potential, which partly compensated for the downregulated SR Ca-ATPase function and supported contraction in the patients with HF[20–22]. Because the antibody against alpha-2 repeat was proven to increase both INa/Ca and ICa,L in the present study, it may have the therapeutic potential to improve systolic function in HF patients by increasing Ca2+ entry via NCX and also the L-type Ca2+ channel.
Our investigation showed that the antibody against alpha-2 repeat could also increase ICa,L besides enhancement of Na+-Ca2+ exchange current. Moreover, the current-voltage (I–V) relationship curve of ICa,L was not shifted by the antibody (40 nmol/L) and EC50 of ICa,L was similar to that of INa/Ca. An early study has shown that in cardiac muscle where Ca influx across the sarcolemma is essential for contraction, the L-type Ca2+ channel has four homologous domains (I–IV), each comprising six transmembrane segments (S1–S6)[23]. Mutational analysis indicated that S5–S6 linkers were highly conserved in domains I–IV[23] and contributed to formation of the ion pore[24]. Residues 1079–1110 were supposed to be located on the S5–S6 linker in domain III[25]. Meanwhile, mutation analysis showed that residues 815–839 of alpha-2 repeat in NCX were involved in the interaction of the exchanger with Na+ and Ca2+[9–12]. We compared the amino acid alignment of the alpha-2 repeat in NCX with the residues 1079–1110 of the L-type Ca2+ channel using EMBOSS Pairwise Alignment Algorithms (European Bioinformatics Institute), which showed that the degree of amino acid similarity was 28.1% between these two functional segments (Figure 7), providing a clue for the non-specific action of the antibody on ICa,L.

From the genetic and evolutionary points of view, intramolecular repeats are thought to arise from intra-genetic duplications, and can not survive throughout evolution unless they are essential to protein function[8]. As we know, alpha-repeats exist in all known members of the NCX family, which implies that alpha-repeats arose early in the evolutionary history and furthermore, their existing as a tandem pair is essential for the protein to operate properly. In the present study, we first observed the effect of the antibody against alpha-2 repeat on INa/Ca in adult rat cardiomyocytes with the whole-cell patch clamp technique. Our results showed that antibody against alpha-2 repeat was a stimulating antibody because it increased INa/Ca in a concentration-dependent manner, which provided supplemental evidence that alpha-2 repeat was essential to translocation of sodium and calcium by NCX.
Recent topological research showed that the alpha-2 repeat comprised putative transmembrane 7 and its C-terminal segment and formed a domain mostly acces-sible from the cytoplasm[26]. However, our results in this study indicated that the antibody against alpha-2 repeat could stimulate Na+-Ca2+ exchange from the external side of the cardiomyocytes. Then why did the antibody play its role from the extracelluar side? One possibility is that the interaction between antibody against alpha-2 repeat and NCX might lead to conformation alteration of the exchanger molecule, just as what happens to KB-R7943[27].
The present study showed that the antibody against alpha-2 repeat of Na+-Ca2+ exchanger was a stimulating antibody to NCX and could also increase ICa,L in a concentration-dependent manner, whereas it did not have an obvious effect on INa and IK.
References
- Reuter H, Seitz N. The dependence of calcium efflux from cardiac muscle on temperature and external ion composition. J Physiol 1968;195:451-70.
- Baker PF, Blaustein MP, Hodgkin AL, Steinhardt RA. The influence of calcium on sodium efflux in squid axons. J Physiol 1969;200:431-58.
- Kang TM, Hilgemann DW. Multiple transport modes of the cardiac Na+/Ca2+ exchanger. Nature 2004;427:544-8.
- Bers DM. Altered cardiac myocyte Ca regulation in heart failure. Physiology 2006;21:380-7.
- Reppel M, Fleischmann BK, Reuter H, Pillekamp F, Schunkert H, Hescheler J. Regulation of Na+/Ca2+ exchange current in the normal and failing heart. Ann NY Acad Sci 2007;1099:361-72.
- Weisser TJ, Piacentino V III, Gaughan JP, Margulies K, Houser SR. Calcium entry via Na/Ca exchange during the action potential directly contributes to contraction of failing human ventricular myocytes. Cardiovasc Res 2003;57:974-85.
- Nicoll DA, Longoni S, Philipson KD. Molecular cloning and functional expression of the cardiac sarcolemmal Na+-Ca2+ exchanger. Science 1990;250:562-5.
- Schwarz EM, Benzer S. Calx, a Na-Ca exchanger gene of . Proc Natl Acad Sci USA 1997; 94: 10 249–54.
- Iwamoto T, Nakamura TY, Pan Y, Uehara A, Imanaga I, Shigekawa M. Unique topology of the internal repeats in the cardiac Na+/Ca2+ exchanger. FEBS Lett 1999;446:264-8.
- Nicoll DA, Hryshko LV, Matsuoka S, Frank JS, Philipson KD. Mutation of amino acid residues in the putative transmembrane segments of the cardiac sarcolemmal Na-Ca exchanger. J Biol Chem 1996; 271: 13 385–91.
- Doering AE, Nicoll DA, Lu Y, Lu L, Weiss JN, Philipson KD. Topology of a functionally important region of the cardiac Na+/Ca2+ exchanger. J Biol Chem 1998;273:778-83.
- Iwamoto T, Uehara A, Nakamura TY, Imanaga I, Shigekawa M. Chimeric analysis of Na/Ca exchangers NCX1 and NCX3 reveals structural domains important for differential sensitivity to external Ni or Li. J Biol Chem 1999; 274: 23 094–102.
- Mubagwa K, Stengl M, Flameng W. Extracellular divalent cations block a cation non-selective conductance unrelated to calcium channels in rat cardiac muscle. J Physiol 1997;502:235-47.
- Hamill OP, Marty A, Neher E, Sakmann B, Sigworth FJ. Improved patch-clamp techniques for high-resolution current recording from cells and cell-free membrane patches. Pflugers Arch 1981;391:85-100.
- Coetzee WA, Ichikawa H, Hearse DJ. Oxidant stress inhibits Na-Ca-exchange current in cardiac myocytes: mediation by sulfhydryl groups? Am J Physiol Heart Circ Physiol 1994;266:H909-19.
- Feng QL, Fan GQ, Zhao JH, Zhao HC, Wu BW. Acquisition and purification of the antibody against α-1 and α-2 repeats of Na+-Ca2+ exchanger. J Shanxi Med Univ 2005;36:395-8.
- Bradford MM. A rapid and sensitive method for the quantitation of microgram quantities of protein utilizing the principle of protein-dye binding. Anal Biochem 1976;72:248-54.
- Kimura J, Miyamae S, Noma A. Identification of sodium-calcium exchange current in single ventricular cells of guinea-pig. J Physiol 1987;384:199-222.
- Bers DM. Cardiac excitation-contraction coupling. Nature 2002;415:198-205.
- Despa S, Islam M, Weber CR, Pogwizd SM, Bers DM. Intracellular Na+ concentration is elevated in heart failure but Na/K pump function is unchanged. Circulation 2002;105:2543-8.
- Weber CR, Piacentino V III, Ginsburg KS, Houser SR, Bers DM. Na+-Ca2+ exchange current and submembrane [Ca2+] during the cardiac action potential. Circ Res 2002;90:182-9.
- Weber CR, Piacentino V III, Houser SR, Bers DM. Dynamic regulation of sodium/calcium exchange function in human heart failure. Circulation 2003;108:2224-9.
- Mikami A, Imoto K, Tanabe T, Niidome T, Mori Y, Takeshima H, et al. Primary structure and functional expression of the cardiac dihydropyridine-sensitive calcium channel. Nature 1989;340:230-3.
- Nakai J, Adams BA, Imoto K, Beam KG. Critical roles of the S3 segment and S3–S4 linker of repeat I in activation of L-type calcium channels. Proc Natl Acad Sci USA 1994;91:1014-8.
- Murphy BJ, Tuana BS. Calcium ions inhibit the allosteric interaction between the dihydropyridine and phenylalkylamine binding site on the voltage-gated calcium channel in heart sarcolemma but not in skeletal muscle transverse tubules. Can J Physiol Pharmacol 1990;68:1389-95.
- Ren X, Nicoll DA, Philipson KD. Helix packing of the cardiac Na-Ca exchanger: proximity of transmembrane segments 1, 2, and 6. J Biol Chem 2006; 281: 22 808–14.
- Iwamoto T, Kita S, Uehara A. Structural domains influencing sensitivity to isothiourea derivative inhibitor KB-R7943 in cardiac Na+/Ca2+ exchanger. Mol Pharmacol 2001;59:524-31.