Ginsenoside Rg1 activated CaMKIIa mediated extracellular signal-regulated kinase/mitogen activated protein kinase signaling pathway1
Introduction
The mitogen-activated protein kinase (MAPK) is a family of serine/threonine protein kinases that transduce extracellular stimuli into intracellular posttranslational and transcriptional responses[1,2]. The MAPK family mainly includes extracellular signal-regulated protein kinase (ERK), p38 MAPK, c-Jun N-terminal kinase/stress-activated protein kinase (JNK/SAPK), and ERK5. The ERK cascade, like the other MAPK cascades, is distinguished by a characteristic core cascade of the three kinases. The first kinase is a so-called MAP kinase kinase kinase (MAPKKK, Raf-1 and B-Raf in the ERK cascade), which activates the second, a MAP kinase kinase (MAPKK, MEK in the ERK cascade), by serine/threonine phosphorylation. MEKs are dual specificity kinases, which in turn activate a MAPK kinase (P44 MAPK=ERK1, P42 MAPK=ERK2) by phosphorylating both a threonine and a tyrosine residue[3].
The ERK/MAPK kinase pathway was first discovered for its role in regulating the cell cycle. Recent research indicates that ERK/MAPK plays important roles in the central nervous system: promoting the differentiation of cells; involving rodent behavior, learning and memory; and acting as a critical factor for neuronal plasticity such as long-term potentiation (LTP)[4–6]. Stimuli that induce LTP in area CA1 of the hippocampus also potently activate ERK/MAPK[7], and pharmacological inhibition of MEK partially inhibited LTP formation[4]. Furthermore, the gene expression-dependent L-LTP is blocked completely by the MEK inhibitor PD98059. Thus, ERK/MAPK signal pathway not only promotes the induction of LTP, but is also involved in the maintenance of LTP by promoting the transcription and translation of new proteins. Thus, ERK is an important regulator of neuronal function in the physiological and pathological condition. The ERK/MAPK pathways in the primary afferents, as well as in the spinal cord and central nervous system may be potential targets for pharmacological intervention for synaptic plasticity or learning and memory improvement.
Ginsenoside Rg1, is extracted from ginseng, the root of Panax Ginseng C A Meyer (Araliaceae). Ginseng, as one of the most extensively used traditional Chinese medicines throughout the world, has a number of pharmacological effects. Accumulating evidence demonstrates that ginsenosides, also called ginseng saponins, are the major active ingredients. Within more than 30 different ginsenosides, Rg1 has been shown to have beneficial effects on the central nervous system, especially in improving learning and memory[8,9]. Rg1 improved cognition in several animal models, such as aged, OVX and cerebral ischemia-reperfusion rats[9–11]. In vitro, neuroprotective effects were demonstrated on neuronal cell lines, primary cortical neurons and nigral neurons damaged by glutamate, β-amyloid protein or rotenome[12,13]. Rg1 increased synaptic plasticity of freely moving rats and enhanced the basic synaptic transmission and the magnitude of LTP induced by high frequency stimulation[14,15].
The study of the action mechanism of Rg1 on cognition and LTP showed that the increased synaptic plasticity may be attributed to the increased expression of GAP-43 in granule cell layer of DG and the onset of the sprouting of granule cell axon-MF. Nitric oxide produced by nNOS played a role in the induction of PP-DG LTP in anesthetized rats[14,15]. Furthermore, recent studies showed that ginsenoside Rg1 exerted its presynaptic facilitatory effect likely through the activation of protein kinase A. Our lab also previously demonstrated Rg1 promoted the release of neurotransmission[16,17]. However, the molecular mechanism of Rg1 in neuron was not still elucidated clearly. In the present study, the effect of Rg1 on ERK/MAPK pathway was investigated.
Materials and methods
Materials Anti-phospho-ERK1/2, anti-phospho-CaMKIIα subunit, anti-β-actin primary antibodies and anti-rabbit IgG secondary antibody were purchased from Santa Cruz Biotechnology (Santa Cruz, CA, USA). Anti-phospho-CREB antibody was obtained from Cell Signaling Biotechnology (Hertfordshire, England). KN93, PD98059, Hoechst 33258 were obtained from Sigma. Dulbecco’s Modified Eagle’s Medium (DMEM), fetal bovine serum (FBS) and equine serum were purchased from Gibco BRL (New York, NY, USA). Fluorescein isothiocyanate (FITC) labeled goat anti-rabbit IgG and Fluo-3/AM ester were purchased from Biotium Inc. (CA, USA). Enhanced chemiluminescent (ECL) substrate was from Pierce (Rockford, IL, USA). Ginsenoside Rg1 with purity greater than 98% was obtained from Jilin University (Changchun, Jilin Province, China) and triturated into fine powder before being dissolved in double distilled water (DDW).
Animals Male Wistar rats (180–220 g) in this study were provided by the Experimental Animal Center of the Chinese Academy of Medical Sciences. Rats were housed in a temperature- and light-control room (23°C, 12 h light cycle) and had free access to food and water. All animals were handled in accordance with the standards established in the Guide for the Care and Use of Laboratory Animals published by the Institute of Laboratory Animal Resources of the National Research Council (United States) and approved by the Animal Care Committee of the Peking Union Medical College and the Chinese Academy of Medical Sciences.
Cell cultures and treatment with Rg1 PC12 cells, purchased from the American Tissue Type Collection, were grown in 75-cm2 tissue culture flasks in DMEM supplemented with 5% FBS and 5% equine serum, penicillin (100 IU/mL), streptomycin (100 mg/L), and L-glutamine (2 mmol/L). Cultures were maintained at 37 °C in 95% air, 5% CO2 in a humidified incubator. PC12 cells were seeded into a 35-mm culture dish. Cells were incubated under either experimental or control conditions for a total of 8 min, beginning with the change of the culture medium with HEPES-buffered saline (HBS) containing 150 mmol/L NaCl, 5.4 mmol/L KCl, 1.8 mmol/L CaCl2, 0.8 mmol/L MgSO4, 20 mmol/L glucose, and 20 mmol/L HEPES (pH 7.2). Various concentrations of Rg1 (0.1, 1 and 10 µmol/L) were added into PC12 cells for a dose-dependent study. In the time-dependent study, the cells were incubated with Rg1 for 0, 1, 2, 4, 6, and 8 min, respectively. After a 30 min pre-incubation with or without 10 µmol/L KN93 or 50 µmol/L PD98059, the PC12 cells were stimulated by adding Rg1 and incubated for a specified period. Then the cells were rapidly washed with HBS and then either fixed for immunofluorescence experiments or lysed for immunoblotting. The final concentration of Rg1 and the duration of the treatments as indicated in the results sections were optimized to maximize the effects on phosphorylation.
Preparation of synaptosomes and treatment with Rg1 Synaptosomes were prepared from the cerebral cortices of 2-month-old male Sprague-Dawley rats[18]. Cerebral cortices were dissected and homogenized in 0.32 mol/L sucrose buffer at 4 °C with 12 strokes in a glass teflon homogenizer at 900 r/min. Nuclei and debris were removed by centrifugation (900×g for 10 min). Crude synaptosomes were isolated from the resultant supernatants by centrifugation at 14 400×g for 20 min and resuspended in freshly aerated (95% O2, 5% CO2 for 10 min) Krebs-Henseleit-HEPES (KHH) buffer containing 118 mmol/L NaCl, 3.5 mmol/L KCl, 1.2 mmol/L CaCl2, 1.2 mmol/L MgSO4, 1.2 mmol/L KH2PO4, 25 mmol/L NaHCO3, 10 mmol/L HEPES–NaOH, and 11.5 mmol/L glucose (pH 7.4). The aliquots of synaptosomes were incubated at 37 °C without (control) or with 10 μmol/L Rg1 in KHH buffer for 1, 2, 4, 6, and 8 min. The incubated synaptosomes were then pelleted by centrifugation (735×g for 20 min).
Western blotting analysis Cells or synaptosomes were collected and lysed in a lysis buffer (pH=8.0) containing 50 mmol/L Tris-Cl, 150 mmol/L NaCl, 1% NP-40, 1 mmol/L PMSF, 1 mmol/L EDTA, 50 mmol/L NaF, 1 mmol/L dithiothreitol (DTT), 1 mg/L aprotinin and 1% Triton X-100 on ice. The protein concentration was determined by using a BCA protein assay kit. Equal amount of proteins were separated by 9% sodium dodecyl sulfate-polyacrylamide gel electrophoresis (SDS-PAGE), then transferred to polyvinylidene fluoride (PVDF) membrane (Millipore). The membrane was blocked by 3% bovine serum albumin (BSA) and incubated with anti-phospho-ERK1/2, anti-phospho-CaMKIIα, anti-phospho-CREB antibody, followed by horseradish peroxides (HRP)-conjugated secondary antibody, and detected with the ECL plus detection system (Molecular Devices, Lmax). The density of each band was quantified by using image analysis software (Science Lab 2005 Image Guage; Fuji Film Co. Ltd, Tokyo, Japan).
Confocal microscopy analysis Measurement of calcium flux was carried out by confocal microscopy. Briefly, PC12 cells were grown in specialized glass-bottom microwell dishes (Corning Corporation, USA) and loaded with 10 µmol/L Fluo-3/AM in HEPES-buffered saline at 37 °C for 30 min in the dark. The cells were rinsed with HEPES-buffered saline and stimulated with 10 µmol/L Rg1. Fluorescence was monitored at 490 nm (excitation wavelength) and 530 nm (emission wavelength) every 5 s using a Leica TCSNT confocal fluorescence microscope. The measurement was completed at room temperature and each field of cells was selected randomly. The images were analyzed for relative fluorescence using Leica confocal software. All calcium flux assays were carried out in the presence of extracellular calcium in the assay buffers. Therefore, both intracellular calcium release and extracellular calcium influx were analyzed.
The effects of Rg1 on the phosphorylation of ERK1/2, CREB, CaMKIIα were also observed by confocal microscopy. The PC12 cells were seeded in a slide pre-coating with poly-L-lysine and treated with Rg1 at the final concentration of 10 µmol/L. The PC12 cells were fixed in 4% paraformaldehyde containing 4% sucrose and 4 mmol/L egtazic acid (EGTA) for 10 min at room temperature. After fixation, the cells were washed thrice with phosphate-buffered saline (PBS) and then permeabilized with 0.2% (v/v) Triton X-100 in PBS. Subsequently, the cells were blocked with 5% goat serum in PBS for 1 h at room temperature and washed once with PBS. The cells were then incubated at 4 °C overnight with a 1:200 dilution of anti-phospho-ERK1/2, anti-phospho-CaMKIIα, or anti-phospho-CREB antibody in PBS containing 5% goat serum. They were washed thrice with PBS for 10 min each and then incubated with a 1:50 dilution of FITC-conjugated goat anti-rabbit IgG for 1 h. Immunoreactivity was monitored by a Leica TCSNT confocal fluorescence microscope.
Statistic analysis Data are expressed as mean±SD as indicated. ANOVA followed by Newman-Keuls post hoc tests were carried out to assess the differences between the relevant control and each experimental group. P-values of <0.05 and 0.01 were regarded as statistically significant.
Results
Effects of Rg1 on the phosphorylation of ERK1/2 in PC12 cells and synaptosomes First, we determined the effect of Rg1 at doses of 0.1, 1.0, and 10.0 µmol/L on the phosphorylation of ERK1/2. The results showed that Rg1 enhanced the phosphorylation of ERK1/2, and the maximum effect was observed at the concentration of 10 µmol/L (Figure 1). Thus, 10 µmol/L Rg1 was selected for the following study.
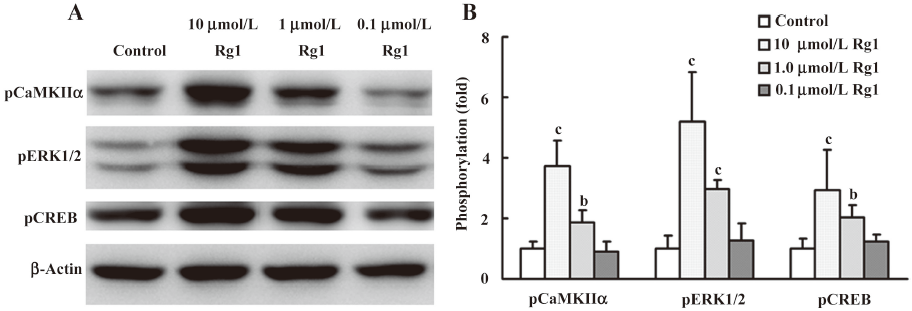
Then, the time-dependent action of Rg1 on the phosphorylation of ERK1/2 was investigated. As shown in (Figure 2), the phosphorylation of ERK1/2 in PC12 cells stimulated by Rg1 was increased gradually within 4 min and decreased from 4 to 8 min, the maximum was reached at 4 min, and the phosphorylation of ERK1/2 has no difference between 8 min and 0 min. To confirm the role of Rg1 on ERK1/2, we also observed its effect on the phosphorylation of ERK1/2 in synaptosomes and similar results were obtained.
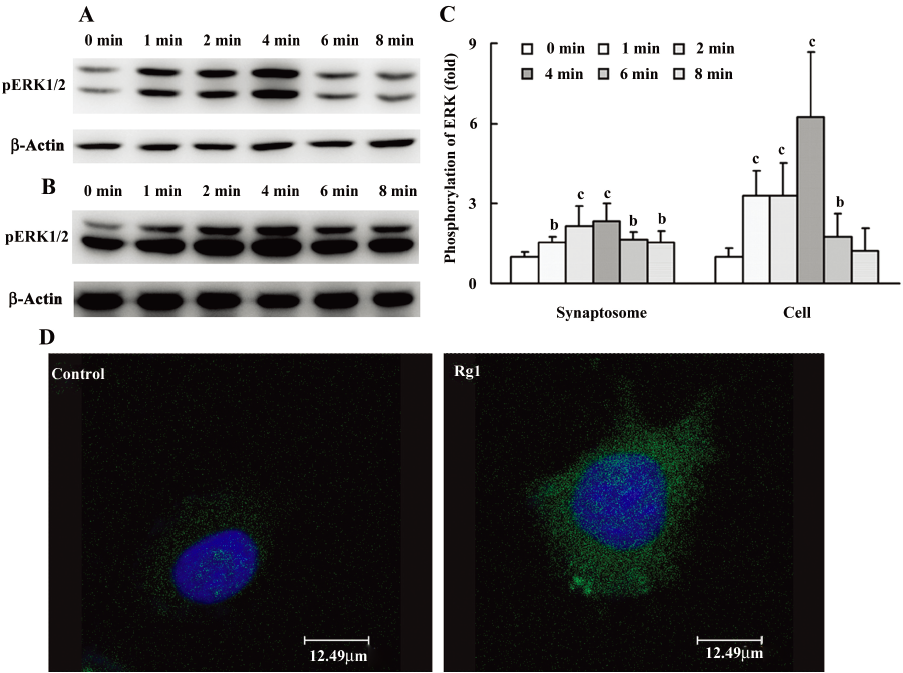
The activation of ERK1/2 by Rg1 in PC12 cells was subsequently detected with confocal microscopy. The results showed that Rg1 increased phosphorylation of ERK increased in both cytoplasm and cell nucleus, indicating that activated ERK1/2 further activated the downstream transcription factors.
Rg1 promoted the intracellular calcium ion flux and activated CaMKIIα It has been demonstrated that Ca2+ activates the ERK/MAPK pathway in PC12 cells and primary neuron cultures[19]. Thus, the intracellular calcium ion flux was determined by confocal microscopy. The results showed that Rg1 promoted the increase of intracellular calcium ion rapidly (Figure 3), suggesting that intracellular calcium ion flux in PC12 cells induced by Rg1 played an important role during the activation of ERK1/2.
CaMKII is known as one of the downstream effecters of calcium signals and exerts its effect at least in part via the ERK1/2 pathway[20]. So the effect of Rg1 on the phosphorylation of CaMKIIα at Thr286 was detected by both Western blotting analysis and confocal microscopy. The results showed that Rg1 activated CaMKIIα at a concentration-dependent manner (Figure 1). The phosphorylated CaMKIIα in the Rg1-treated group increased rapidly within 2 min and was stronger than that in the control for 8 min (Figure 4).
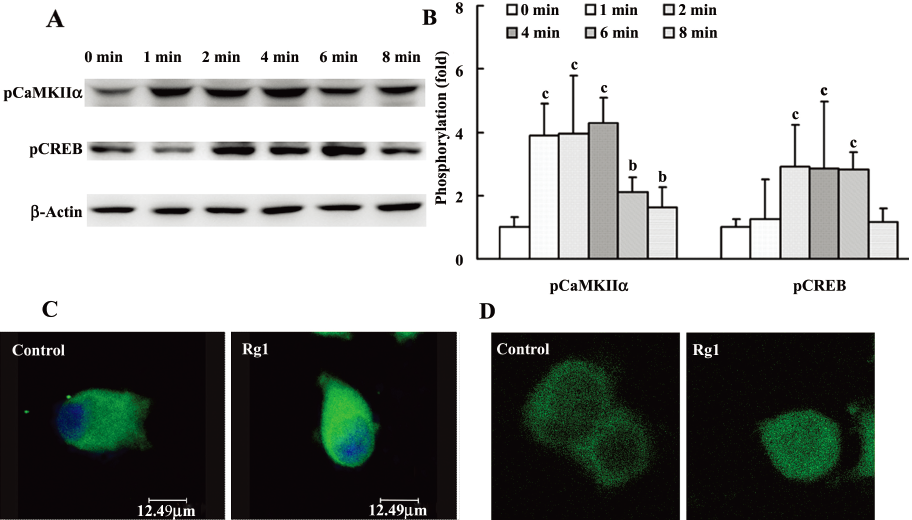
CREB was activated by Rg1 in PC12 cells CREB is one of the downstream proteins of ERK1/2, which is activated by phosphorylating at ser133 in the kinase-inducible domain of the N-terminal[21]. The results demonstrated that the activation of CREB reached a maximum at the concentration of 10 µmol/L (Figure 1). It is interesting that CREB was persistently activated within 8 min (Figure 4). And the results of confocal microscopy also manifested that the phosphorylation of CREB increased remarkably in the cell nucleus.
Effects of KN93 and PD98059 on the phosphorylation of ERK1/2 and CREB induced by Rg1 in PC12 cells It is well-known that CaMKIIα is located upstream of ERK1/2. Therefore, we tried to investigate whether the enhancement of phosphorylation of ERK1/2 induced by Rg1 was only dependent on the phosphorylation of CaMKIIα. Thus, KN93, the inhibitor of CaMKIIα, was pre-incubated with the PC12 cells for 30 min before Rg1 was added. The results showed that the phosphorylation of ERK1/2 was decreased in the presence of KN93, compared with the Rg1-treated group, but still remarkably increased compared with that in the control group (Figure 5), indicating that Rg1 activated ERK1/2 by other pathways besides CaMKIIα.
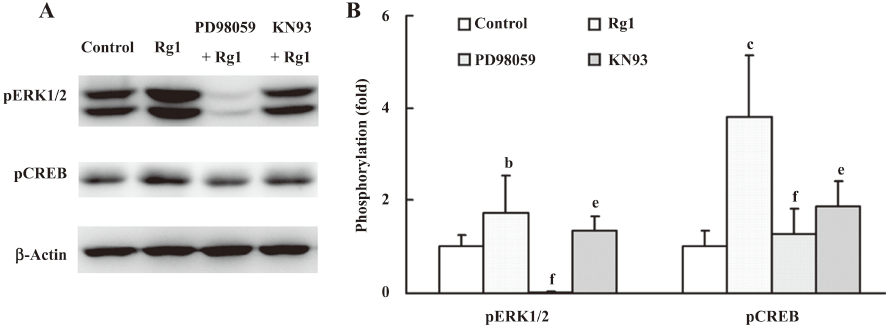
CREB is a transcription factor and the converge point of many protein kinases, including ERK, CaMKII, protein kinase A (PKA), protein kinase C (PKC) and so on[22]. PD98059, an inhibitor of MEK, and KN93 attenuated the phosphorylation of CREB (Figure 5), suggesting ERK1/2 and CaMKIIα are involved in the activation of CREB. Therefore, multiple kinase pathways mediated the activation of CREB induced by Rg1.
Discussion
In the present study, we have demonstrated that Rg1 activated the ERK1/2 pathway through promoting intracellular calcium flux and CaMKIIα phosphorylation. Furthermore, the ERK1/2 and CaMKIIα pathways at least in part mediated the activation of CREB induced by Rg1.
Although the ERK/MAPK kinase pathway was first discovered for its role in regulating the cell cycle, when members of the kinase cascade were found to be present in neurons, another role was ascribed to ERK/MAPK kinase: regulating synaptic plasticity and memory formation[23]. Recent research indicates that ERK/MAPK signaling plays a key role in the induction of LTP. For example, stimuli that induce LTP in area CA1 of the hippocampus also potently activate MAPK[7,24] and the inhibitor of ERK blocks LTP formation in area CA1 of the hippocampus[4]. Several studies have shown that ERK/MAPK is rapidly phosphorylated after LTP and that this activation is essential for the expression of LTP in CA1 in vitro. Furthermore, some work has highlighted the potential role of the ERK/MAPK cascade as a critical trigger to initiate gene transcription after synaptic activation. For example, Early growth response (Egr) transcription factors are among a relatively small number of regulatory immediate early genes (IEGs) that are expressed in response to neuronal activity and coupled to ERK/MAPK signaling[3,4]. Thus, we observed the change of ERK1/2 phosphorylation stimulated by Rg1 in PC12 cells and synaptosome within 8 min. In fact, Rg1 promoted the activation of ERK1/2 at 1 min and reached a maximum at 4 min, which may provide a reasonable explanation for its effects on LTP induction and maintenance.
Activity-dependent change in neuronal processes such as synaptic plasticity and neuronal survival are mediated in large part through elevations in intracellular calcium levels[25]. Many complicated downstream calcium-dependent kinase signaling cascades were revealed, among which, ERK/MAPK is emerging as an important target for neuronal calcium signaling. ERK activation by neuronal calcium flux has been demonstrated both in vitro and in vivo[26,27]. We observed the calcium flux by confocal microscopy. The results showed that Rg1 increased intracellular calcium ion flux rapidly. Thus, in the context of ERK1/2 activation, the calcium flux may be an important factor in determining signaling events.
It is now well established that ERK activation is via multiple molecular partners[28,29]. Among them, CaMKIIα is the major target protein activated by the second messenger calcium ion. We found that Rg1 promoted the activation of CaMKIIα within 2 min. CaMKII is a holoenzyme made up of 8–12 homologous peptides called α and β subunits[30]. When Ca2+/calmodulin binds a subunit of CaMKII, calmodulin displaces the autoregulatory domain of the subunit, enabling it to become phosphorylated by the catalytic domain of a neighboring subunit. Once phosphorylated, the subunit can remain active for a prolonged period, even in the absence of a sustained elevation in Ca2+[31]. The phosphorylation of CaMKIIα induced by Rg1 maintained an increase over 8 min, which may partially contribute to its autophosphorylation.
Regulation of the ERK cascade is complex. The trikinase cascade consists of Raf-1 and B-raf, which activate MEK1/2, the obligate upstream activator of ERK1/2, and upstream of the Raf-MEK-ERK pathway is an enormous variety of membrane receptors, ions and protein kinases[32]. Cross-talk between kinase pathways indicates that ERK/MAPK may be a point of convergence integrating CaMKII, PKA signals and so on[22]. According to the time of reaching the maximum activation of CaMKIIα and ERK1/2, we speculated that CaMKIIα is one of the upstream kinases of ERK1/2 induced by Rg1. Therefore, the CaMKIIα inhibitor KN93 was treated with PC12 cells before Rg1 was added. The results revealed that CaMKIIα mediated the activation of ERK1/2, but only partially. The other protein kinases that participate in the ERK1/2 pathway activated by Rg1 will be further investigated.
The activation of ERK1/2 is linked to the regulation of several transcription factors, CREB is the major downstream target of ERK signaling, which exists in the nucleus and is related to synaptic plasticity. ERK1/2 phosphorylates pp90 ribosomal S6 kinases 1–3 (RSKs 1–3), which translocate to the nucleus and phosphorylate CREB at Ser133 to activate CRE-mediated gene expression. CREB-dependent gene transcription has been shown to be important for synaptic plasticity[22]. PD98059, the inhibitor of MEK, significantly inhibited the activation of CREB by Rg1, suggesting that Rg1 activated CREB through the ERK/MAPK pathway. However, the phosphorylation of CREB in the PD98059-treated group was still stronger than that in the control, which indicated that other kinases mediated the activation of CREB induced by Rg1. It has been found that CREB can be phosphorylated by multiple kinases including PKC, PKA, pp90 RSK, cGMP-dependent protein kinase, CaMKII and others[33]. The CaMKII inhibitor also partially attenuated the activation of CREB induced by Rg1. Therefore, both ERK1/2 and CaMKIIα participated in the activation of CREB induced by Rg1, while other protein kinases, such as PKA and PKC, are to be investigated in the future to confirm whether they mediate the pathway.
This is the first time that Rg1 has been identified to activate the ERK1/2 pathway by increasing the intracellular calcium ion flux and phosphorylating CaMKIIα, eventually activating CREB in PC12 cells. These findings imply that activation of the ERK/MAPK pathway may contribute to the effects of Rg1 on LTP induction and maintenance.
References
- Seger R, Krebs EG. The MAPK signaling cascade. FASEB J 1995;9:726-35.
- Obata K, Noguchi K. MAPK activation in nociceptive neurons and pain hypersensitivity. Life Sci 2004;74:2643-53.
- Sweatt JD. The neuronal MAP kinase cascade: a biochemical signal integration system subserving synaptic plasticity and memory. J Neurochem 2001;76:1-10.
- English JD, Sweatt JD. A requirement for the mitogen-activated protein kinase cascade in hippocampal long term potentiation. J Biol Chem 1997; 272: 19 103–6.
- Schafe GE, Atkins CM, Swank MW, Bauer EP, Sweatt JD, LeDoux JE. Activation of ERK/MAP kinase in the amygdala is required for memory consolidation of pavlovian fear conditioning. J Neurosci 2000;20:8177-87.
- Sweatt JD. Mitogen-activated protein kinases in synaptic plasticity and memory. Curr Opin Neurobiol 2004;14:311-17.
- English JD, Sweatt JD. Activation of p42 mitogen-activated protein kinase in hippocampal long term potentiation. J Biol Chem 1996; 271: 24 329–32.
- Attele AS, Wu JA, Yuan CS. Ginseng pharmacology: multiple constituents and multiple actions. Biochem Pharmacol 1999;58:1685-93.
- Radada K, Gilleb G, Moldzioa R, Saitoc H, Rausch W. Ginsenosides Rb1 and Rg1 effects on mesencephalic dopaminergic cells stressed with glutamate. Brain Res 2004;1021:41-53.
- Leung KW, Yung KK, Mak NK, Chan YS, Fan TP, Wong RN. Neuroprotective effects of ginsenoside-Rg1 in primary nigral neurons against rotenone toxicity. Neuropharmacology 2007;52:827-35.
- Chen XC, Chen LM, Zhu YG, Fang F, Zhou YC, Zhao CH. Involvement of CDK4, pRB, and E2F1 in ginsenoside Rg1 protecting rat cortical neurons from beta-amyloid-induced apoptosis. Acta Pharmacol Sin 2003;24:1259-64.
- Cheng Y, Shen LH, Zhang JT. Anti-amnestic and anti-aging effects of ginsenoside Rg1 and Rb1 and its mechanism of action. Acta Pharmacol Sin 2005;26:143-9.
- Zhang YG, Liu TP. Influences of ginsenosides Rb1 and Rg1 on reversible focal brain ischemia in rats. Acta Phammacol Sin 1996;17:44-8.
- Wang XY, Zhang JT. Effects of ginsenoside Rg1 on synaptic plasticity of freely moving rats and its mechanism of action. Acta Pharmacol Sin 2001;22:657-62.
- Wang XY, Zhang JT. NO mediates ginsenoside Rg1-induced long-term potentiation in anesthetized rats. Acta Pharmacol Sin 2001;22:1099-102.
- Xue JF, Liu ZJ, Hu JF, Chen H, Zhang JT, Chen NH. Ginsenoside Rb1 promotes neurotransmitter release by modulating phosphorylation of synapsins through a cAMP-dependent protein kinase pathway. Brain Res 2006;1106:91-8.
- Chang Y, Huang WJ, Tien LT, Wang SJ. Ginsenosides Rg1 and Rb1 enhance glutamate release through activation of protein kinase A in rat cerebrocortical nerve terminals (synaptosomes). Eur J Pharmacol 2008;578:28-36.
- Jovanovic JN, Sihra TS, Nairn AC, Hemmings HC Jr, Greengard P, Czernik AJ. Opposing changes in phosphorylation of specific sites in synapsin I during Ca2+-dependent glutamate release in isolated nerve terminals. J Neurosci 2001;21:7944-53.
- Bading H, Greenberg ME. Stimulation of protein tyrosine phosphorylation by NMDA receptor activation. Science 1991;253:912-4.
- Miyabe T, Miletic V. Multiple kinase pathways mediate the early sciatic ligation-associated activation of CREB in the rat spinal dorsal horn. Neurosci Lett 2005;381:80-5.
- Shaywitz AJ, Greenberg ME. CREB: a stimulus-induced transcription factor activated by a diverse array of extracellular signals. Annu Rev Biochem 1999;68:821-61.
- Impey S, Obrietan K, Storm DR. Making new connections: role of ERK/MAP kinase signaling in neuronal plasticity. Neuron 1999;23:11-4.
- Shalin SC, Hernandez CM, Dougherty MK, Morrison DK, Sweatt JD. Kinase suppressor of Ras1 compartmentalizes hippocampal signal transduction and subserves synaptic plasticity and memory formation. Neuron 2006;50:765-79.
- Impey S, Obrietan K, Wong ST, Poser S, Yano S, Wayman G, et al. Cross talk between ERK and PKA is required for Ca2+ stimulation of CREB-dependent transcription and ERK nuclear translocation. Neuron 1998;21:869-83.
- Bito H, Takemoto-Kimura S. Ca2+/CREB/CBP-dependent gene regulation: a shared mechanism critical in long-term synaptic plasticity and neuronal survival. Cell Calcium 2003;34:425-30.
- Cohen-Matsliah SI, Brosh I, Rosenblum K, Barkai E. A novel role for extracellular signal-regulated kinase in maintaining long-term memory-relevant excitability changes. J Neurosci 2007; 27: 12 584–9.
- Gorosito SV, Cambiasso MJ. Axogenic effect of estrogen in male rat hypothalamic neurons involves Ca2+, protein kinase C, and extracellular signal-regulated kinase signaling. J Neurosci Res 2008;86:145-57.
- Girault JA, Valjent E, Caboche J, Hervé D. ERK2: a logical AND gate critical for drug-induced plasticity? Curr Opin Pharmacol 2007;7:77-85.
- Valjent E, Caboche J, Vanhoutte P. Mitogen-activated protein kinase/extracellular signal-regulated kinase induced gene regulation in brain: a molecular substrate for learning and memory? Mol Neurobiol 2001;23:83-99.
- Kanaseki T, Ikeuchi Y, Sugiura H, Yamauchi T. Structural features of Ca2+/calmodulin-dependent protein kinase II revealed by electron microscopy. J Cell Biol 1991;115:1049-60.
- Tokumitsu H, Wayman GA, Muramatsu M, Soderling TR. Calcium/calmodulin-dependent protein kinase kinase: identification of regulatory domains. Biochemistry 1997; 36: 12 823–7.
- Shalin SC, Egli R, Birnbaum SG, Roth TL, Levenson JM, Sweatt JD. Signal transduction mechanisms in memory disorders. Prog Brain Res 2006;157:25-41.
- Bradley J, Finkbeiner S. An evaluation of specificity in activity-dependent gene expression in neurons. Prog Neurobiol 2002;67:469-77.