Valproic acid-mediated transcriptional regulation of human GM3 synthase (hST3Gal V) in SK-N-BE(2)-C human neuroblastoma cells1
Introduction
Valproic acid (VPA), a simple branched-chain fatty acid, is an anti-convulsant that is widely used to treat epilepsy and bipolar disorder[1]. Like several other short-chain fatty acids, such as phenylbutyrate and phenylacetate, VPA also mediates anti-cancer activity on several fronts: it inhibits cell growth, induces differentiation, and promotes apoptosis[2,3]. Recent studies have suggested that these effects are at least partly due to VPA-mediated inhibition of histone deacetylases (HDACs), which causes hyperacetylation and subsequent de-repression and transcriptional activation of genes that are silenced in cancer[4–6].
Gangliosides are sialic acid-containing glycosphingolipids found in the outer leaflets of vertebrate plasma membranes; they are particularly abundant in cells of the central nervous system[7]. They play important roles in multiple biological processes, such as cell–cell interaction, adhesion, cell differentiation, growth control, and receptor function[8,9]. In addition, they have been studied as brain tissue markers in various mammals[7,8] and tumor markers in neuroectoderm-derived cells[10] and neuroblastoma cells[11].
Recent reports demonstrated that VPA could modulate the mRNA expression of two polysialyltransferases (ST8Sia II and IV) responsible for polysialylation of neural cell adhesion molecule (NCAM) in human neuroblastoma cells[12]. NCAM is downregulated during the migratory phase of brain tumor invasion, whereas ganglioside expression is upregulated[13], but it is not known whether VPA-mediated changes in sialyltransferase gene expression are responsible for this ganglioside upregulation.
To answer this question, we designed the present study, in which we investigated whether VPA modulates human GM3 synthase (hST3Gal V) mRNA expression, and whether such an effect is correlated to ganglioside GM3 biosynthesis in human neuroblastoma cells. We demonstrate for the first time that VPA does indeed induce hST3Gal V mRNA expression in the human neuroblastoma cell line SK-N-BE(2)-C. Furthermore, to investigate the molecular mechanism underlying this increase in hST3Gal V gene expression, we functionally characterized how its promoter region upregulated transcription of a luciferase reporter in response to VPA.
Materials and methods
Cell cultures The human neuroblastoma cell line SK-N-BE(2)-C, obtained from the American Type Culture Collection (Manassas, VA, USA) was maintained at 37 °C in a 5% CO2 incubator and cultured in Dulbecco’s modified Eagle’s medium (DMEM; WelGENE Co, Daegu, Korea) containing 1 mmol/L sodium pyruvate and 1× MEM non-essential amino acids, supplemented with 100 U/mL of penicillin, 100 µg/mL of streptomycin, and 10% (v/v) fetal bovine serum (FBS) (Gibco BRL, Life Technologies, Grand Island, NY, USA). To induce expression of the hST3Gal V gene with VPA, SK-N-BE(2)-C cells were cultured for various time periods in the presence of 5 mmol/L VPA.
Evaluation of ganglioside GM3 surface expression by VPA To analyze ganglioside GM3 levels on the cell membrane, SK-N-BE(2)-C cells were seeded on round cover slips (pretreated with 2% 3-aminopropyl-triethoxysilan) in an 8-well multi-plate. Upon reaching confluence, cells were given 5 mmol/L VPA for 6 to 12 h. After various incubation times, cell cultures were washed twice with distilled phosphate-buffered saline (PBS), fixed with 4% room-temperature paraformaldehyde (PFA), washed again with PBS, and blocked (5% bovine serum albumin [BSA] in PBS). Cells were then incubated overnight at 4 °C with a GM3 monoclonal antibody (mouse IgM, Kappa-chain, clone: GMR6; Seigakagu; Japan) diluted 1:100 with 5% BSA in PBS. After another wash, cells were labeled with 1:100 fluorescein isothiocyanate (FITC)-conjugated goat anti-mouse IgM (Sigma, St. Louis, MO, USA) as a secondary antibody. The samples were mounted with glycerol and analyzed by confocal laser-scanning microscope (LSM 510; Zeiss, Jena, Germany).
Reverse transcription-polymerase chain reaction Using Trizol reagent (Invitrogen, Carlsbad, CA, USA), total RNA was isolated from SK-N-BE(2)-C cells treated with a vehicle control or VPA, and 2 µg of the RNA was subjected to reverse transcription with random nonamers, using a Takara RNA PCR kit (Takara Bio, Shiga, Japan) according to the manufacturer’s protocol. The resulting cDNA was amplified by PCR with specific hST3Gal V primers (413 bp), 5’-CCCTGCCATTCTGGGTACGAC-3’ (sense) and 5’-CACGATCAATGCCTCCACTGAGATC-3’ (antisense); β-actin (247 bp), 5’-CAAGAGATGGCCACGGCTGCT-3’ (sense) and 5’-TCCTTCTGCATCCTGTCGGCA-3’ (antisense) was also amplified as a loading control. PCR products were analyzed by 1.5% agarose gel electrophoresis and visualized with ethidium bromide. The intensity of the bands obtained from the RT-PCR product was estimated with a Scion Image Instrument (Scion Corp, Frederick, MD, USA). The values were calculated as a percent of the control and are expressed as means±SD.
Assessment of cytotoxic effects The cytotoxic effect of VPA on SK-N-BE(2)-C cells was investigated using a commercially-available proliferation kit (XTT; Boehringer Mannheim, Mannheim, Germany). Briefly, the cells were suspended in culture medium, plated in 96-well culture plates at a density of 3×104 cells per well, and incubated for 24 h in a 37 °C humidified incubator under an atmosphere of 5% CO2. The medium was replaced with 100 µL of serum-free DMEM culture medium containing various concentrations (0–10 mmol/L) of VPA. After incubation for 12 h or 24 h, each well received 50 µL of the XTT reaction solution, which was sodium 3'-[1-(phenyl-aminocarbonyl)-3,4-tetrazolium]-bis (4-methoxy-6-nitro) benzenesulfonic acid hydrate and N-methyl dibenzopyrazine methyl sulfate; mixed in a 50:1 ratio (5 mL of XTT-labeling reagent and 100 µL of electron coupling reagent). After 4 h of incubation at 37 °C in a 5% CO2 incubator, absorbance was measured on an enzyme-linked immunosorbent assay (ELISA) plate reader (Bio-Rad, Hercules, CA, USA) at the test wavelength of 490 nm. All determinations were confirmed using at least three identical replicates. The data shown are the means±SD of three independent experiments.
Transfection and luciferase assay The luciferase reporter plasmids used herein, namely pGL3-1600 and its derivatives (pGL3-83 to pGL3-1210) with base substitutions in the cAMP-responsive element (CRE) binding protein (CREB) binding site, have been described elsewhere[14]. To analyze hST3Gal V promoter activity in response to VPA treatment, SK-N-BE(2)-C cells (3.0×105 cells/well) were seeded in 24-well tissue culture plates and allowed to grow to 70% confluence, at which point they were transiently co-transfected with 0.5 μg of the indicated reporter plasmid and 50 ng of the control Renilla luciferase vector pRL-TK (Promega, Madison, WI, USA), using 1 µL Lipofectamine 2000 (Invitrogen). After a 12-h recovery in normal medium without VPA, the medium was changed to medium containing 5 mmol/L VPA and incubated for an additional 9 h, after which cells were collected and treated with passive lysis buffer (Promega). Firefly and Renilla luciferase activities were measured using the dual-luciferase reporter assay system (Promega), according to the manufacturer’s instructions, and a GloMax 20/20 luminometer (Promega). Firefly luciferase activity of the reporter plasmid was normalized to Renilla luciferase activity and expressed as a fold induction over the empty pGL3-Basic vector, used as a negative control. Independent triplicate experiments were carried out for each plasmid.
Electrophoretic mobility shift assay Electrophoretic mobility shift assay (EMSA) was carried out using a gel shift assay system kit (Promega) according to the manufacturer’s instructions. Nuclear extracts of VPA-induced SK-N-BE(2)-C cells were prepared as described previously[14], and the protein concentrations of the extracts were determined using a Bio-Rad protein assay kit. EMSA probes were constructed as follows: double-stranded oligonucleotides were synthesized using two sets of oligonucleotides encompassing the CREB binding site; subsequently, they were end-labeled with [γ-32P] dATP using a T4 polynucleotide kinase.
Results
VPA induced hST3Gal V gene expression, and subsequent GM3 expression, in SK-N-BE(2)-C cells To determine whether stimulation with VPA upregulated the hST3Gal V gene in SK-N-BE(2)-C cells, we treated cells with varying doses of VPA for varying periods of time, and we examined their expression of hST3Gal V using RT-PCR. As shown in Figure 1, hST3Gal V mRNA levels were higher in SK-N-BE(2)-C cells that received VPA. During stimulation with 5 mmol/L VPA, hST3Gal V mRNA increased remarkably after 6 h of treatment, but it decreased after 24 h. In cells treated with 1 or 3 mmol/L VPA, however, we detected hST3Gal V mRNA only after 24 h of treatment (data not shown). These results clearly demonstrated that VPA stimulated the expression of hST3Gal V. To investigate whether VPA-mediated hST3Gal V induction increased cellular levels of the ganglioside GM3, we used immunofluorescent confocal microscopy to visualize GM3 expression in VPA-stimulated SK-N-BE(2)-C cells. We observed higher GM3 expression in VPA-treated cells than in control cells not subjected to VPA treatment (Figure 2).
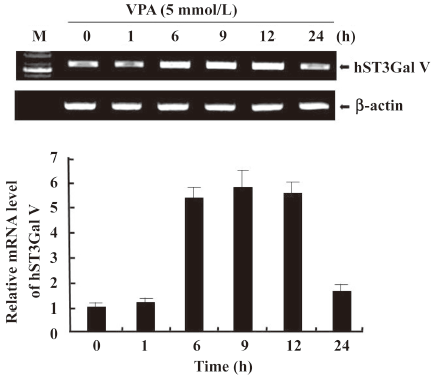
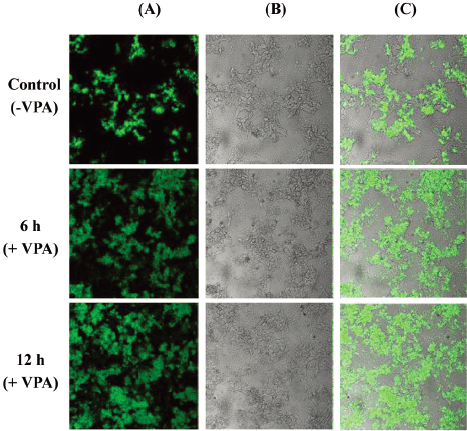
Cell growth and morphological features of VPA-stimulated SK-N-BE(2)-C cells In previous studies, VPA induced apoptosis and differentiation of neuroblastoma cells, both in vivo and in vitro[15–17]. To recapitulate these observations in SK-N-BE(2)-C cells, we used an XTT assay to investigate whether VPA exhibited any cytotoxic effects, and we microscopically examined the cells to monitor morphological changes associated with differentiation. As shown in Figure 3, 5 mmol/L VPA was not cytotoxic to the cells after 24 h. Moreover, even at twice that dose (10 mmol/L), VPA did not significantly affect cell viability after 24 h. With regard to morphological changes, although a 1 h treatment with 5 mmol/L VPA had no detectable effect on cell morphology, a 6 h treatment induced a change in morphology that made cells appear more differentiated compared with the negative control (Figure 4).
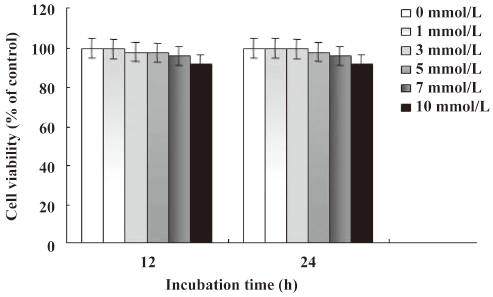
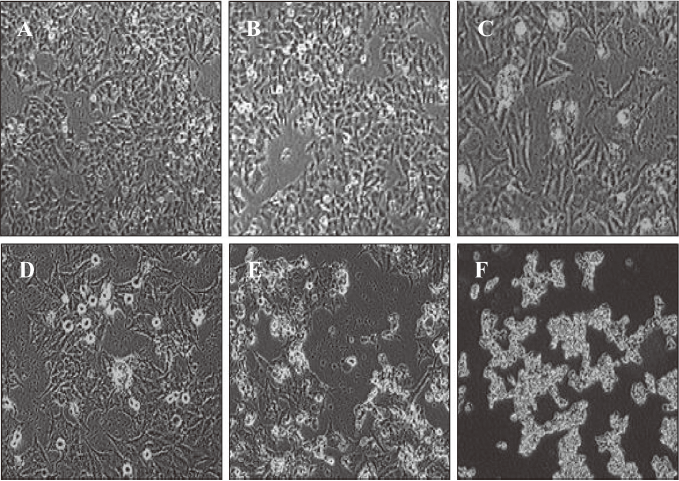
Analysis of transcriptional activity of hST3Gal V promoter by VPA in SK-N-BE(2)-C cells To determine whether the 5'-flanking sequence of the hST3Gal V gene contains a VPA-responsive promoter, we prepared luciferase constructs carrying serial 5’deletions of the hST3Gal V promoter, transfected them into SK-N-BE(2)-C cells, and treated the transfected cells with VPA. We monitored subsequent expression of the luciferase reporter gene using the dual-luciferase reporter assay system, after which we measured luciferase activity with a luminometer. As shown in Figure 5, cells harboring the pGL3-177 construct showed a remarkable increase in luciferase activity after VPA treatment, about 2-fold higher than untreated transfected cells. In contrast, VPA stimulation did not change the luciferase activity in cells expressing the pGL3-basic (negative control) or the 5'-deleted hST3Gal V promoter construct. These results suggested that potential positive regulatory elements existed in the region contained in the pGL3-177 construct, and that the upstream region (-1600 to -177) negatively regulated transcriptional activity. Because deleting the promoter region to nucleotide –83 markedly reduced reporter activity to negative control levels, we concluded that the region containing nucleotides -177 to -83 had a pivotal role in inducing the expression of hST3Gal V. Based on our results, we proposed that this region functioned as the VPA-inducible promoter in SK-N-BE(2)-C cells.
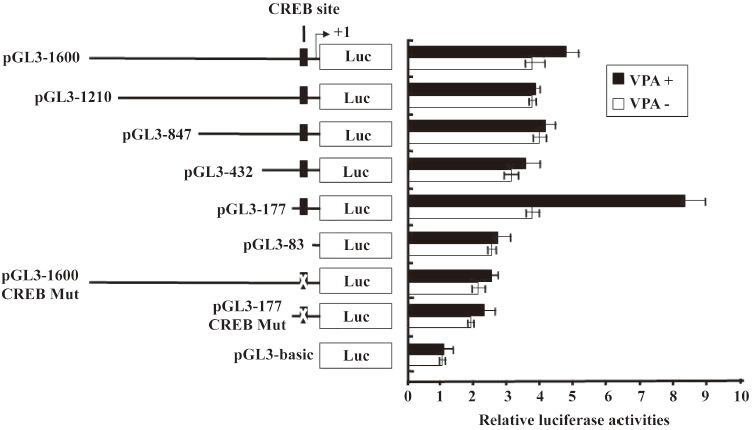
Our previous study[18] demonstrated that the region from -177 to -83 contained NFY, CREB, SP1, EGR3, and MZF1 binding sites, but that only the CREB and MZF1 binding sites were present in the correct orientation. This consensus CREB binding site (TGACGTCA), namely a CRE at position -143 to -136, was crucial for TPA-induced expression of hST3Gal V in HL-60 cells [14,19].
To determine whether this CRE contributed to VPA-induced expression of hST3Gal V in SK-N-BE(2)-C cells, we carried out EMSA on nuclear extracts from VPA-treated cells, using a double-stranded 32P-labeled oligo fragment (20 bp) containing the CRE consensus sequence as the probe. In VPA-treated nuclear extracts, we detected a DNA-protein complex that we did not detect in untreated nuclear extract (Figure 6). This complex completely disappeared after specific competitive inhibition with a 50-fold molar excess of unlabeled oligo fragment, but not after a 50-fold molar excess of an unlabeled mutant CRE oligonucleotide (a nonspecific competitor). These results indicated that this complex contains CREB protein or other protein binding to this oligo fragment (20 bp).
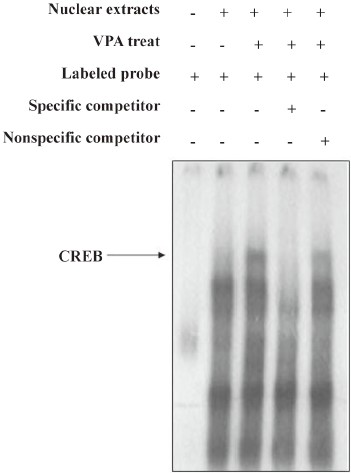
To verify whether CRE played an important role in VPA-induced expression of hST3Gal V in neuroblastoma cells, we prepared plasmids expressing mutations at the pGL3-1600 or pGL3-177 CRE sites (dubbed pGL3-1600mtCREB and pGL3-177mtCREB, respectively), and we transfected them into SK-N-BE(2)-C cells. Subsequently, we treated these transfected cells with VPA or a vehicle control. VPA-treated cells harboring these mutations markedly exhibited reduced luciferase activity compared with cells expressing the wild-type reporter construct (Figure 5). Taken as a whole, these results indicated that this CRE site is crucial for VPA-induced expression of hST3Gal V in SK-N-BE(2)-C cells, and that VPA induces the interaction between the CREB protein and this region of DNA to induce hST3Gal V expression.
Discussion
In the present study, we demonstrated for the first time that VPA upregulated the expression of hST3Gal V mRNA in human neuroblastoma cells. Moreover, this increase was time-dependent: we detected the induced hST3Gal V mRNA signal after 6 h of VPA treatment, the signal increased up to the 12 h time point, and it had decreased by 24 h. As a downstream consequence, this VPA treatment markedly increased levels of the ganglioside GM3 in SK-N-BE(2)-C cells, as shown by immunofluorescent confocal microscopy, and our results suggested that the VPA-induced increase in hST3Gal V gene expression exhibited a close temporal relation to the GM3 induction. We also revealed that the promoter region of the hST3Gal V gene contains VPA-responsive element(s), which supported the idea that hST3Gal V gene induction would eventually direct GM3 formation in response to VPA.
In previous in vitro and in vivo studies, VPA induced apoptosis and differentiation of various neuroblastoma cell lines [15-17]. VPA also reportedly inhibited the growth and differentiation of SK-N-BE(2)-C cells, and these effects were associated with altered expression of differentiation genes and decreased adhesion of cells to the endothelium[20]. In line with these reports, the results herein revealed that VPA induced morphological differentiation of SK-N-BE(2)-C cells, but that it had no significant effects on cell viability even after a 24-h treatment with 10 mmol/L VPA.
We also unraveled a part of the transcriptional regulation mechanism that underlies hST3Gal V gene induction in response to VPA signaling. In order to investigate VPA-responsive elements involved in the enhanced expression of the hST3Gal V gene in SK-N-BE(2)-C cells, we first sought to identify the region within the hST3Gal V promoter that was critical for VPA-induced gene expression. We isolated the region between –177 and –83 as the core promoter; this region was required for transcriptional activation of hST3Gal V in VPA-induced SK-N-BE(2)-C cells.
One of our previous studies revealed several transcription factor binding sites in this region, most notably a consensus CRE (TGACGTCA) at position –143 to –136 [18]. This site was the only transcription factor binding site that contributed to hST3Gal V promoter activity during phorbol ester-induced monocytic differentiation of HL-60 cells[14,19]. In agreement with these findings, our present site-directed mutagenesis and EMSA analyses indicated that binding to this CRE element mediated VPA-dependent upregulation of hST3Gal V gene expression.
The CREB transcription factor is a downstream target of a variety of signaling transduction pathways, and it mediates various cell responses like proliferation, differentiation, and adaptive responses to cellular processes[21,22]. Several signaling pathways, such as PKA, PKC, Ca2+/CaMKs, SAPK/JNK, p38MAPK, and ERKs could recruit CREB to the CRE in the promoter, and the capacity of CREB to activate transcription is regulated by its phosphorylation state at serine 133[21,22]. It is known that CREB and AP-1 are abundantly expressed in neuronal cells[23]. Multiple intracellular signal transduction pathways control the DNA binding activities of AP-1 and CREB, both of which bind to unique DNA consensus sequences in the regulatory domain of genes[24].
Although VPA enhances AP-1 mediated gene expression by activating the MAP kinase signaling pathway in human neuroblastoma SH-SY5Y cells[25,26], no one has described a scenario in which VPA mediates gene expression through CREB in human neuroblastoma cells. Therefore, it is important to elucidate which signaling pathways are upstream of this CREB-mediated enhanced expression of the hST3Gal V gene, and such studies are currently in progress.
References
- Rogawski MA, Loscher W. The neurobiology of antiepileptic drugs. Nat Rev Neurosci 2004;5:553-64.
- Johannessen CU. Mechanisms of action of valproate: a commentatory. Neurochem Int 2000;37:103-10.
- Blaheta RA, Michaelis M, Driever PH, Cinatl J Jr. Evolving anticancer drug valproic acid: insights into the mechanism and clinical studies. Med Res Rev 2005;25:383-97.
- Gottlicher M, Minucci S, Zhu P, Kramer OH, Schimpf A, et al. Valproic acid defines a novel class of HDAC inhibitors inducing differentiation of transformed cells. EMBO J 2001;20:6969-78.
- Phiel CJ, Zhang F, Huang EY, Guenther MG, Lazar MA, Klein PS. Histone deacetylase is a direct target of valproic acid, a potent anticonvulsant, mood stabilizer, and teratogen. J Biol Chem 2001;276:36734-41.
- Gurvich N, Tsygankova OM, Meinkoth JL, Klein PS. Histone deacetylase is a target of valproic acid-mediated cellular differentiation. Cancer Res 2004;64:1079-86.
- Svennerholm L. Gangliosides and synaptic transmission. Adv Exp Med Biol 1980;125:533-44.
- Hakomori S, Igarashi Y. Gangliosides and glycosphingolipids as modulators of cell growth, adhesion, and transmembrane signaling. Adv Lipid Res 1993;25:147-62.
- Varki A. Biological roles of oligosaccharides: all of the theories are correct. Glycobiology 1993;3:97-130.
- Hakomori S. Glycosphingolipids in cellular interaction, differentiation, and oncogenesis. Annu Rev Biochem 1981;50:733-64.
- Cheung NK, Saarinen UM, Neely JE, Landmeier B, Donovan D, Coccia PF. Monoclonal antibodies to a glycolipid antigen on human neuroblastoma cells. Cancer Res 1985;45:2642-49.
- Beecken WD, Engl T, Ogbomo H, Relja B, Cinatl J, Bereiter-Hahn J, et al. Valproic acid modulates NCAM polysialylation and polysialyltransferase mRNA expression in human tumor cells. Int Immunopharmacol 2005;5:757-69.
- Gratsa A, Rooprai HK, Rogers JP, Martin KK, Pilkington GJ. Correlation of expression of NCAM and GD3 ganglioside to motile behavior in neoplastic glia. Anticancer Res 1997;17:4111-8.
- Chung TW, Choi HJ, Lee YC, Kim CH. Molecular mechanism for transcriptional activation of ganglioside GM3 synthase and its function in differentiation of HL-60 cells. Glycobiology 2005;15:233-44.
- Cinatl J Jr, Cinatl J, Driever PH, Kotchetkov R, Pouckova P, Kornhuber B, et al. Sodium valproate inhibits in vivo growth of human neuroblastoma cells. Anticancer Drugs 1997;8:958-63.
- Cinatl J Jr, Cinatl J, Scholz M, Driever PH, Henrich D, Kabickova H, et al. Antitumor activity of sodium valproate in cultures of human neuroblastoma cells. Anticancer Drugs 1996;7:766-73.
- Stockhausen MT, Sjölund J, Manetopoulos C, Axelson H. Effects of the histone deacetylase inhibitor valproic acid on Notch signalling in human neuroblastoma cells. Br J Cancer 2005;92:751-9.
- Kim SW, Lee SH, Kim KS, Kim CH, Choo YK, Lee YC. Isolation and characterization of the promoter region of the human GM3 synthase gene. Biochim Biophys Acta 2002;1578:84-9.
- Choi HJ, Chung TW, Kang NY, Kim KS, Lee YC, Kim CH. Transcriptional regulation of the human GM3 synthase (hST3Gal V) gene during monocytic differentiation of HL-60 cells. FEBS Lett 2003;555:204-8.
- Cinatl J Jr, Kotchetkov R, Blaheta R, Driever PH, Vogel JU, Cinatl J. Induction of differentiation and suppression of malignant phenotype of human neuroblastoma BE(2)-C cells by valproic acid: enhancement by combination with interferon-a. Int J Oncol 2002;20:97-106.
- De Cesare D, Fimia GM, Sassone-Corsi P. Signaling routes to CREM and CREB: plasticity in transcriptional activation. TIBS 1999;24:281-5.
- Shaywitz AJ, Greenberg ME. CREB: a stimulus-induced transcription factor activated by a diverse array of extracellular signals. Annu Rev Biochem 1999;68:821-61.
- Sheng M, Greenberg ME. The regulation and function of c-fos and other immediate early genes in the nervous system. Neuron 1990;4:477-85.
- Chen G, Yuan PX, Hawver DB, Potter WZ, Manji HK. Increase in AP-1 transcription factor DNA binding activity by valproic acid. Neuropsychopharmacology 1997;16:238-45.
- Chen G, Yuan PX, Jiang YM, Huang LD, Manji HK. Valproate robustly enhances AP-1 mediated gene expression. Mol Brain Res 1999;64:52-8.
- Yuan PX, Huang LD, Jiang YM, Gutkind JS, Manji HK, Chen G. The mood stabilizer valproic acid activates mitogen-activated protein kinases and promotes neurite growth. J Biol Chem 2001;276:31674-83.