Curcumin attenuates the release of pro-inflammatory cytokines in lipopolysaccharide-stimulated BV2 microglia1
Introduction
Microglia have been suggested to play a role in host defense and tissue repair in the central nervous system (CNS)[1]. The activation of microglia is also observed in brain injuries and is induced after exposure to lipopolysaccharides (LPS), interferon-γ, or β-amyloid[2,3]. Once chronically activated, microglia can produce a variety of proinflammatory mediators and potentially neurotoxic compounds. These mediators include proinflammatory cytokines [eg, interleukin (IL)-1β, IL-6, and TNF-α], reactive oxygen, nitric oxide (NO) and prostaglandin E2 (PGE2), which are thought to be responsible for brain injuries and diseases including trauma, ischemia, Alzheimer’s disease and neural death[4]. Therefore, it is not surprising that LPS was reported to increase the expressions of NO, PGE2, and pro-inflammatory cytokines in microglial cells after CNS injury[5]. Among these mediators, PGE2 and NO are the products of the inducible isoforms of cyclooxygenase (COX)-2 and inducible NO synthase (iNOS) enzymes, respectively[6]. The overexpression of pro-inflammatory cytokines has emerged as an important determinant of the cytotoxicity associated with inflammation in pathologies, such as Alzheimer’s disease, cerebral ischemia and multiple sclerosis[7]. Thus, the inhibition of these pro-inflammatory mediators offers a potentially effective therapeutic approach to mitigate the progression of neurodegen-erative diseases.
Curcumin, the active component in turmeric, has been shown to possess anti-inflammatory, antioxidant, and antitumor activities[8–10]. Our previous study has also shown that curcumin significantly ameliorates LPS-induced functional maturation of dendritic cells. This function involves the inhibition of NF-κB inhibition by blocking IκB degradation[11]. Recent evidence suggests that the anti-inflammatory effects of curcumin in BV2 microglia are closely related to the suppression of COX-2 expression through the inhibition of the NF-κB and AP-1 pathways[12]. Other reports have also ascribed curcumin’s neuroprotective effect against ischemia-induced neuronal damage to its antioxidant capacity, which would reduce oxidative stress and inhibit the activation of signaling cascades that lead to apoptotic cell death[13]. Curcumin has been strongly implicated in the expression of NF-κB, however the expression of NF-κB-related genes and proteins is largely unknown in microglia.
In this study, we investigated curcumin’s effects on the regulation of iNOS, NO, COX-2, PGE2, and pro-inflammatory cytokines (IL-1β, IL-6, and TNF-α) in LPS-stimulated BV2 microglia. Curcumin significantly inhibited the inflammatory mediators in LPS-stimulated BV2 microglia. Moreover, the biological effects of curcumin may involve the inhibition of the NF-κB pathway. The present study’s results may provide critical information that will contribute to the therapeutic use of curcumin in the prevention of microglial activation.
Materials and methods
Materials Curcumin, LPS, Tween-20 and p-nitrophenyl phosphate, 3-(4,5-dimethylthiazol-2-yl)-2,5-diphenyltetrazo-lium bromide (MTT) were purchased from Sigma (St Louis, MO, USA). Rabbit anti-human iNOS, COX-2, p65, and nucleolin polyclonal antibodies were purchased from Santa Cruz Biotechnology (Santa Cruz, CA, USA). The antibody against β-actin was also from Sigma. Peroxidase-labeled goat antirabbit immunoglobulin was purchased from Amersham (Arlington Heights, IL, USA). Dulbecco’s modified Eagle’s medium (DMEM)–containing L-arginine (200 mg/L)–fetal bovine serum (FBS), and other tissue culture reagents were purchased from Gibco (Grand Island, NY, USA). Other chemicals were purchased from Sigma.
Cell culture The BV2, immortalized murine microglial cell line was provided by Dr E J CHOI (Korea University, South Korea). The BV2 cells were constructed by infecting primary microglia with a v-raf/v-myc oncogene-carrying retrovirus (J2). The murine BV2 microglia were cultured in DMEM supplemented with 10% FBS, 100 U/mL penicillin, and 100 mg/mL streptomycin, and maintained in a humidified incubator with 5% CO2. In all the experiments, the cells were pretreated for 60 min in the presence of the indicated concentrations of curcumin before the addition of LPS (0.5 µg/mL) in serum-free DMEM.
Cytotoxicity assay Cell viability was evaluated by the MTT reduction assay. In brief, cells (5×105 cells/mL) were seeded and treated with various reagents for the indicated time periods. After various treatments, the medium was removed and the cells were incubated with 0.5 mg/mL of MTT solution. After incubation for 3 h at 37 °C and in 5% CO2, the supernatant was removed and the formation of formazan crystals was measured at 540 nm with a microplate reader.
Isolation of total RNA and RT-PCR Total RNA was isolated according to the manufacturer’s instructions. RNA (3 µg) was reverse-transcribed using M-MLV reverse transcriptase (Promega, Madison, WI, USA). Single-stranded cDNA was amplified by PCR with primers for iNOS, COX-2, GAPDH, IL-1β, IL-6, and TNF-α, respectively. The primer sequences for these cDNA have been described in previous studies[14,15]. The following PCR conditions were applied. GAPDH: 18 cycles of denaturation at 94 °C for 30 s, annealing at 57 °C for 30 s, and extension at 72 °C for 30 s; iNOS, COX-2, IL-1β, IL-6, and TNF-α: 25 cycles of denaturation at 94 °C for 30 s, annealing at 52 °C for 30 s, and extension at 72 °C for 30 s. The GAPDH was used as an internal control to evaluate the relative expressions of COX-2 and iNOS, IL-1β, IL-6, and TNF-α.
Western blot analysis The cells were washed with phosphate-buffered solution three times, incubated at 4 °C, and lysed for 30 min in lysis buffer (1% Triton X-100, 1% deoxycholate, and 0.1% NaN3). The lysates were then centrifuged at 12 000×g at 4 °C. The supernatants were collected for further analysis. Equal amounts of protein were separated electrophoretically using 10% SDS-PAGE and then the gel was transferred to 0.45 µm polyvinylidene fluoride (PVDF: Millipore, Bedford, MA, USA). The membranes were soaked in blocking buffer (5% skimmed milk), incubated overnight with primary antibodies, incubated with horseradish peroxidase conjugated antibodies, and then detected by the enhanced chemiluminescence detection system according to the recommended procedure (Amer-sham, USA). In a parallel experiment, the nuclear protein was prepared using lysis buffer [10 mmol/L Tris-Cl (pH 7.4), 3 mmol/L CaCl2, 2 mmol/L MgCl2, 0.5 mmol/L dithiothreitol, 0.5 mmol/L phenylmethylsulfonyl fluoride, and protease inhibitors] for 15 min at 4 °C. After centrifugation and washing of the nuclei pellet, ice-cold hypertonic extraction buffer [20 mmol/L HEPES (pH 7.1), 25% glycerol, 420 mmol/L NaCl, 0.2 mmol/L EDTA, 1.5 mmol/L MgCl2, 0.5 mmol/L dithiothreitol, and protease inhibitors] was added and the samples were incubated at 4 °C for 30 min with constant shaking. The nuclear protein extracts were isolated by centrifugation at 12 000×g for 30 min.
Nitrite assays (Griess assay) The NO levels in the culture supernatants were measured by a Griess reaction. The cells were seeded in 24-well plates (5×105 cells/mL) and stimulated for 24 h with LPS. After LPS stimulation, 100 µL conditioned culture medium from each sample was mixed with the same volume of the Griess reagent (1% sulfanilamide/0.1% N-(1-naphthyl)-ethylenediamine dihydrochloride/2.5% H3PO4). The NO concentration was determined by measuring the absorbance at 540 nm with a 96-well microplate spectrophotometer (Labsystems Inc, Franklin, MA, USA). The nitrite concentration was calculated with reference to a standard curve of sodium nitrite generated by known concentrations.
Measurement of PGE2 TheBV2 cells were subcultured in 6-well plates (5×105 cells /mL) and incubated with curcumin (0, 5, 10, and 20 μmol/L, respectively) in the presence or absence of LPS (0.5 μg/mL) for 24 h. One hundred microliters of culture-medium supernatant was collected for the determination of the PGE2 concentration by ELISA kit (Cayman, MI, USA).
ELISA The BV2 cells were subcultured in 6-well plates (5×105 cells /mL) and incubated with curcumin (0, 5, 10, and 20 µmol/L) in the presence or absence of LPS (0.5 µg/mL) for 24 h. The cell-free supernatant was collected at 24 h stimulation with LPS, and IL-1β, IL-6, and TNF-α were measured by ELISA kits (R&D, Minneapolis, MN, USA) according to the manufacturer’s instructions. The absorbance at 450 nm was determined using a microplate reader. The minimum detection levels were as follows: 10 ng/mL for IL-1β, 15.6 ng/mL for IL-6, and 15.6 pg/mL for TNF-α.
NF-κB luciferase assay A total of 1×106 BV2 cells were transfected with 2 µg NF-κB-luciferase reporter plasmids (BD Sciences, San Jose, CA, USA) using Lipofecta-mine according to the manufacturer’s protocol (Gibco, USA). After incubating with DNA–Lipofectamine mixtures, the cells were pre-incubated in the presence or absence of curcumin before being stimulated with LPS for 6 h. After stimulation, the cells were washed, lysed, and assayed for luciferase activity according to the manufacturer’s instructions (Luciferase Assay System kit; Promega) with a microplate luminometer LB96V (Perkin–Elmer, Foster City, CA, USA).
Statistical analysis Data were presented as the mean±SD of at least 3 separate experiments. Comparisons between 2 groups were analyzed using Student’s t-test. P-values less than 0.05 were considered to be statistically significant.
Results
Curcumin decreases NO and PGE2 production in LPS-stimulated BV2 microglia in a dose-dependent manner To evaluate the anti-inflammatory effects of curcumin on LPS-stimulated BV2 microglia, cell culture media were collected, and NO and PGE2 levels were measured in LPS-stimulated BV2 microglia. The amount of NO significantly increased from 7.5±3.3 µmol/L to 33.9 ± 4.2 µmol/L 24 h after LPS stimulation (Figure 1). Curcumin suppressed NO production in LPS-stimulated BV2 microglia to 21.2±3.4 µmol/L and 12.3±3.8 µmol/L at a concentration of 10 and 20 µmol/L, respectively. To investigate whether curcumin inhibits PGE2 production, the cells were also pretreated with curcumin for 1 h and then stimulated with 0.5 µg/mL LPS for 24 h. While the unstimulated cells produced low levels of PGE2, the LPS-stimulated cells exhibited an 8-fold increase in their PGE2 expression (Figure 1B). Curcumin inhibited LPS-mediated PGE2 production in a dose-dependent manner. These results indicate that curcumin significantly inhibited NO and PGE2 production in BV2 microglia following LPS stimulation.
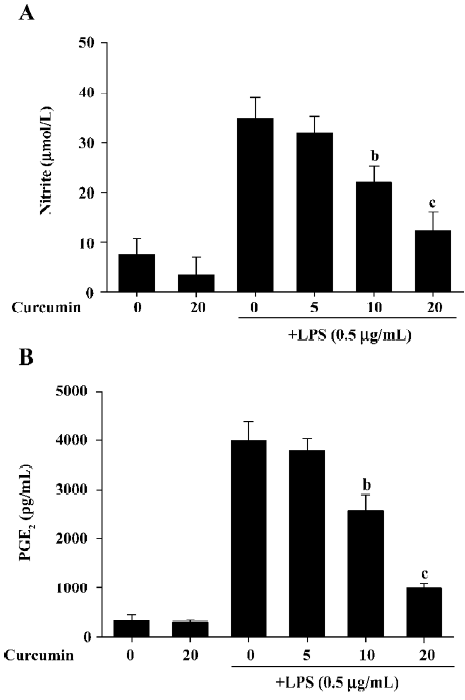
It is possible that the observed inhibition of NO and PGE2 production was due to curcumin’s cytotoxicity in LPS-stimulated BV2 microglia. In order to exclude this possibility, we performed MTT assays in BV2 microglia treated with curcumin for 24 h. The results demonstrated that curcumin treatment did not increase the MTT activity, even at a concentration of 20 µmol/L (Figure 2). These data confirmed that the inhibition of NO and PGE2 production in LPS-stimulated BV2 microglia was not due to a cytotoxic action of curcumin.
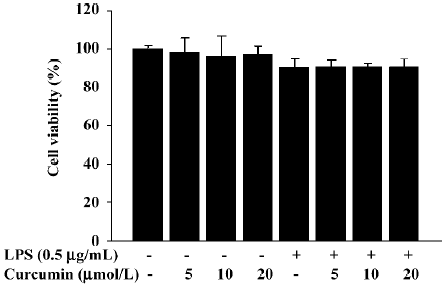
Curcumin attenuates the expressions of LPS-stimulated iNOS and the COX-2 protein and mRNA We further examined whether curcumin’s inhibitory effect on NO and PGE2 production was associated with decreased iNOS and COX-2 expressions in LPS-stimulated BV2 microglia. A Western blot analysis revealed that iNOS and COX-2 protein levels were undetectable in unstimulated BV2 microglia. LPS treatment significantly increased iNOS and COX-2 protein levels; however, these expressions were markedly attenuated in BV2 microglia pretreated with curcumin (Figure 3A). Consistent with these results, the RT-PCR analysis showed that iNOS and COX-2 mRNA transcription also decreased following curcumin pretreatment (Figure 3B). These results showed that curcumin significantly suppressed the expressions of iNOS and COX-2, which was upregulated by LPS treatment through transcriptional inhibition.
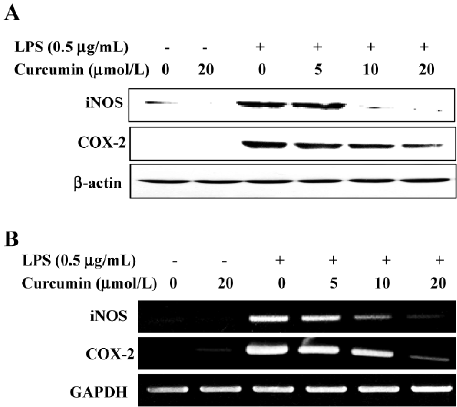
Curcumin inhibits the production of pro-inflammatory cytokines, such as IL-1β, IL-6 and TNF-α at transcriptional levels We next investigated curcumin’s effect on the expression of pro-inflammatory cytokines, such as IL-1β, IL-6 and TNF-α. BV2 microglia were incubated with curcumin (0, 5, 10, and 20 µmol/L) in the presence or absence of LPS (0.5 µg/mL). After treatment with LPS for 24 h, the levels of the cytokines in the culture media were measured by ELISA. Curcumin had no effect on the production of IL-1β, IL-6, and TNF-α in normal BV2 microglia. However, the levels of IL-1β, IL-6, and TNF-α levels increased in the culture media containing LPS-stimulated BV2 microglia (Figure 4A). Pretreatment with curcumin resulted in a dose-dependent decrease in cytokine production. We next determined whether curcumin could regulate the transcription of IL-1β, IL-6, and TNF-α mRNA. IL-1β, IL-6, and TNF-α mRNA were not detectable under normal culture conditions; however, RT–PCR assays revealed that these cytokines were significantly upregulated following stimulation with LPS (0.5 µg/mL) for 6 h (Figure 4B). Pretreatment with curcumin for 1 h attenuated the upregulation of these cytokines in a dose-dependent manner. These results suggested that curcumin acted principally by preventing the accumulation of proinflammatory cytokines by inhibiting the transcription of these genes.
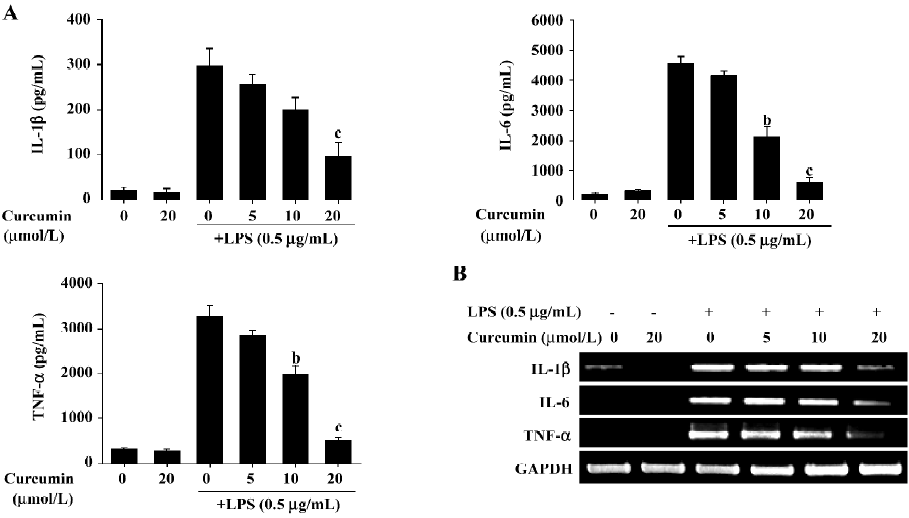
Inhibitory effects of curcumin are mediated by NF-κB suppression in LPS-stimulated BV2 microglia Because the activation of NF-κB by LPS can induce the expression of proinflammatory mediators[16,17], we next investigated curcumin’s effects on NF-κB activity using luciferase assays and Western blot analysis. BV2 microglia were pretreated with curcumin for 1 h and then stimulated with LPS for 6 h. The expression of a NF-κB reporter construct was measured by relative luciferase activity. As shown in Figure 5A, LPS significantly enhanced NF-κB activity. This activity was significantly inhibited following a 1 h pretreatment with 10 or 20 µmol/L curcumin. Curcumin’s effect on LPS-induced NF-κB p65 translocation into the nucleus was also investigated. Significant NF-κB p65 proteins were localized within the nucleus at 15 and 30 min after LPS treatment (Figure 5B). Curcumin pretreatment significantly attenuated the observed nuclear translocation (Figure 5). These results indicated that curcumin’s inhibition of NF-κB nuclear translocation may be the mechanism responsible for the suppression of NO, PGE2, and pro-inflammatory cytokines in LPS-stimulated BV2 microglia.
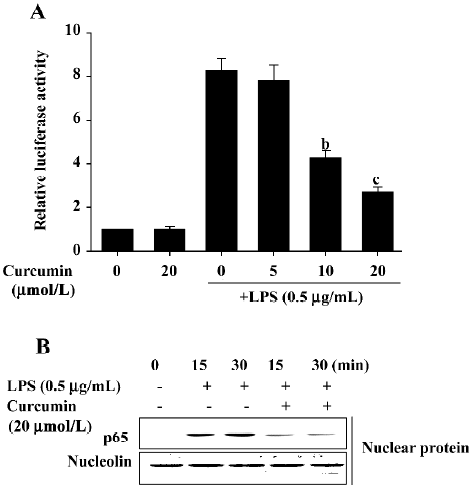
Discussion
The present results showed that more than 10 µmol/L of curcumin significantly inhibited the production of NO and PGE2 in LPS-stimulated BV2 microglia. These inhibitory effects of curcumin were accompanied by the decreases in the expression of iNOS and COX-2 at the transcriptional levels. The magnitude of this inhibition was directly proportional to the concentration of curcumin added to the culture media. In addition, curcumin significantly attenuated the transcription of proinflammatory cytokines, such as IL-1β, IL-6, and TNF-α. Furthermore, this suppression was associated with a decrease in NF-κB transcriptional activity.
Several lines of evidence have shown that the expression of COX-2 and iNOS, the key enzymes for NO and PGE2 production, is upregulated in activated glial cells[18]. Additionally, cytokines such as IL-1β, IL-6, and TNF-α, are known to promote pro-inflammatory responses, both in vitro and in vivo[19,20]. As previous studies have shown curcumin suppresses LPS-induced COX-2 expression in BV2 microlgial cells[12] and NO production in primary rat microglia[21]. Although previous data also have suggested that brain injuries stimulate the expression of pro-inflammatory cytokines, NO, and PGE2 in microglia, curcumin’s effects on LPS-stimulated microglia have yet to be elucidated. Like the previous data, the present study revealed that curcumin treatment significantly inhibited NO and PGE2 production in LPS-stimulated BV2 microglia. Curcumin’s inhibitory effects further attenuated the expression of iNOS and COX-2 mRNA/protein levels, indicating that curcumin influenced the transcriptional level. Our data first indicate that curcumin suppresses the production of pro-inflammatory cytokines, including IL-1β, IL-6, and TNF-α. These results indicated that approximately more than 10 µmol/L curcumin may be a promising candidate for mitigating LPS-stimulated microglial activation.
NF-κB is known to play a critical role in the regulation of cell survival genes and to coordinate the expression of pro-inflammatory enzymes and cytokines, such as iNOS, COX-2, IL-1β, IL-6, and TNF-α[22–24]. Furthermore, blocking the transcriptional activity of NF-κB in the microglial nucleus can suppress the expression of iNOS, COX-2, and pro-inflammatory cytokines[25,26]. Previous studies have revealed that curcumin inhibits NF-κB and AP-1, and reduces the production of iNOS, COX-2, and TNF-α in human cancer cells[27,28]. Because the expression of these pro-inflammatory mediators is known to be modulated by NF-κB, we used luciferase reporter assays and investigated p65 nuclear translocation assays to elucidate whether curcumin inhibits NF-κB activity by using p65 translocation activity. The present study demonstrated that curcumin’s inhibition of NF-κB activation is responsible for the inhibition of pro-inflammatory mediators. The present study also indicated that the amount of p65 translocated in the nucleus was significantly attenuated in BV2 microglia by pretreatment with curcumin. Our findings suggested that curcumin’s transcriptional down-regulation of pro-inflammatory mediators was due to the inhibition of the NF-κB signaling pathway.
Some reports have shown that curcumin inhibits cytokine-mediated NF-κB activation by blocking IκB kinase activity in intestinal epithelial and mouse fibroblast cells and that it also attenuates phorbol ester-induced c-Jun/AP-1 activation[29–31]. Curcumin also suppresses Janus kinase-signal transducers and activator of transcription inflammatory signaling by activating Src homology 2 domaining tyrosine phosphatase 2 in brain microglia[32]. A recent study has shown that curcumin reduced oxidative damage and amyloid pathology in an Alzheimer’s transgenic mouse[33,34]. Recent data also have suggested that curcumin may effectively modulate mitogen-activated protein kinases (MAPK) to regulate anti-inflammatory effects in stimulated microglia[21]. Therefore, future experiments are necessary to confirm whether curcumin tightly regulates the expression of other nuclear transcriptional factors and MAPK to induce anti-inflammatory effects in LPS-stimulated microglia.
In conclusion, the present study demonstrates that curcumin exhibits anti-inflammatory activity by suppressing the release of proinflammatory mediators in LPS-stimulated BV2 microglia. Curcumin significantly inhibited the release of NO, PGE2, IL-1β, IL-6 and TNF-α in a dose-dependent manner. Curcumin’s anti-inflammatory properties are mediated by the downregulation of NF-κB in LPS-stimulated BV2 microglia. These findings enhance curcumin’s importance as a compound for treating LPS-mediated sepsis syndrome and inflammatory disease.
References
- Perry VH, Gordon S. Macrophages and microglia in the nervous system. Trends Neurosci 1988;11:273-7.
- Giulian D, Li J, Li X, George J, Rutecki PA. The impact of microglia-derived cytokines upon gliosis in the CNS. Dev Neurosci 1994;16:128-36.
- Zielasek J, Hartung HP. Molecular mechanisms of microglial activation. Adv Neuroimmunol 1996;6:191-222.
- Meda L, Cassatella MA, Szendrei GI, Otvos L Jr, Baron P, Villalba M, et al. Activation of microglial cells by β-amyloid protein and IFN-γ. Nature 1995;374:647-50.
- Vegeto E, Bonincontro C, Pollio G, Sala A, Viappiani S, Nardi F, et al. Estrogen prevents the lipopolysaccharide-induced inflammatory response in microglia. J Neurosci 2001;21:1809-18.
- Vane JR, Mitchell JA, Appleton I, Tomlinson A, Bishop-Bailey D, Croxtall J, et al. Inducible isoforms of cyclooxygenase and nitric-oxide synthase in inflammation. Proc Natl Acad Sci USA 1994;91:2046-50.
- Tomimoto H, Akiguchi I, Wakita H, Lin JX, Budka H. Cyclooxygenase-2 is induced in microglia during chronic cerebral ischemia in humans. Acta Neuropathol 2000;99:26-30.
- Oetari S, Sudibyo M, Commandeur JN, Samhoedi R, Vermeulen NP. Effects of curcumin on cytochrome P450 and glutathione S-transferase activities in rat liver. Biochem Pharmacol 1996;51:39-45.
- Huang MT, Lysz T, Ferraro T, Abidi TF, Laskin JD, Conney AH. Inhibitory effects of curcumin on in vitro lipoxygenase and cyclooxygenase activities in mouse epidermis. Cancer Res 1991;51:813-9.
- Rao CV, Rivenson A, Simi B, Reddy BS. Chemoprevention of colon carcinogenesis by dietary curcumin, a naturally occurring plant phenolic compound. Cancer Res 1995;55:259-66.
- Kim GY, Kim KH, Lee SH, Yoon MS, Lee HJ, Moon DO, et al. Curcumin inhibits immunostimulatory function of dendritic cells: MAPKs and translocation of NF-κB as potential targets. J Immunol 2005;174:8116-24.
- Kang G, Kong PJ, Yuh YJ, Lim SY, Yim SV, Chun W, et al. Curcumin suppresses lipopolysaccharide-induced cyclooxygenase-2 expression by inhibiting activator protein 1 and nuclear factor kB binding in BV2 microglia cells. J Pharmacol Sci 2004;94:325-8.
- Wang Q, Sun AY, Simonyi A, Jensen MD, Shelat PB, Rottinghaus GE, et al. Neuroprotective mechanisms of curcumin against cerebral ischemia-induced neuronal apoptosis and behavioral deficits. J Neurosci Res 2005;82:138-48.
- Tha KK, Okuma Y, Miyazaki H, Murayama T, Uehara T, Hatakeyama R, et al. Changes in expressions of proinflammatory cytokines IL-1β, TNF-α and IL-6 in the brain of senescence accelerated mouse (SAM) P8. Brain Res 2000;885:25-31.
- Kang JH, Sung MK, Kawada T, Yoo H, Kim YK, Kim JS, et al. Soybean saponins suppress the release of proinflammatory mediators by LPS-stimulated peritoneal macrophages. Cancer Lett 2005;230:219-27.
- Mukaida N, Ishikawa Y, Ikeda N, Fujioka N, Watanabe S, Kuno K, et al. Novel insight into molecular mechanism of endotoxin shock: biochemical analysis of LPS receptor signaling in a cell-free system targeting NF-κB and regulation of cytokine production/action through β2 integrin in vivo. J Leukoc Biol 1996;59:145-51.
- Guha M, Mackman N. LPS induction of gene expression in human monocytes. Cell Signal 2001;13:85-94.
- McGeer PL, Kawamata T, Walker DG, Akiyama H, Tooyama I, McGeer EG. Microglia in degenerative neurological disease. Glia 1993;7:84-92.
- Dinarello CA. Cytokines as endogenous pyrogens. J Infect Dis 1999;179:S294-304.
- Feldmann M, Brennan FM, Maini R. Cytokines in autoimmune disorders. Int Rev Immunol 1998;17:217-28.
- Jung KK, Lee HS, Cho JY, Shin WC, Rhee MH, Kim TG, et al. Inhibitory effect of curcumin on nitric oxide production from lipopolysaccharide-activated primary microglia. Life Sci 2006;79:2022-31.
- Roshak AK, Jackson JR, McGough K, Chabot-Fletcher M, Mochan E, Marshall LA. Manipulation of distinct NF-κB proteins alters interleukin-1b-induced human rheumatoid synovial fibroblast prostaglandin E2 formation. J Biol Chem 1996;271:31496-501.
- Schmedtje JF, Ji YS, Liu WL, DuBois RN, Runge MS. Hypoxia induces cyclooxygenase-2 via the NF-κB p65 transcription factor in human vascular endothelial cells. J Biol Chem 1997;272:601-8.
- Xie QW, Kashiwabara Y, Nathan C. Role of transcription factor NF-κB/Rel in induction of nitric oxide synthase. J Biol Chem 1994;269:4705-8.
- Petrova TV, Akama KT, Van Eldik LJ. Cyclopentenone prostaglandins suppress activation of microglia: down-regulation of inducible nitric oxide synthase by 15-deoxy-Δ12,14-prostaglandin J2. Proc Natl Acad Sci USA 1999;96:4668-73.
- Ye SM, Johnson RW. Regulation of interleukin-6 gene expression in brain of aged mice by nuclear factor κB. J Neuroimmunol 2001;117:87-96.
- Han SS, Keum YS, Seo HJ, Surh YJ. Curcumin suppresses activation of NF-κB and AP-1 induced by phorbol ester in cultured human promyelocytic leukemia cells. J Biochem Mol Biol 2002;35:337-42.
- Surh YJ, Chun KS, Cha HH, Han SS, Keum YS, Park KK, et al. Molecular mechanisms underlying chemopreventive activities of anti-inflammatory phytochemicals: down-regulation of COX-2 and iNOS through suppression of NF-κB activation. Mutat Res 2001;480-481:243-68.
- Huang TS, Lee SC, Lin JK. Suppression of c-jun/AP-1 activation by an inhibitor of tumor promotion in mouse fibroblast cells. Proc Natl Acad Sci USA 1991;88:5292-6.
- Jobin C, Bradham CA, Russo MP, Juma B, Narula AS, Brenner DA, et al. Curcumin blocks cytokine-meidated NF-κB activation and proinflammatory gene expression by inhibiting inhibitory factor I-κB kinase activity. J Immunol 1999;163:3474-83.
- Singh S, Aggarwal BB. Activation of transcription factor NF-κB is suppressed by curcumin. J Biol Chem 1995;270:24995-5000.
- Kim HY, Park EJ, Joe EH, Jou I. Curcumin suppresses Janus kinases-STAT inflammatory signaling through activation of Src homology 2 domain-containing tyrosine phosphatase 2 in brain microglia. J Immunol 2003;171:6072-9.
- Lim GP, Chu T, Yang F, Beech W, Frautschy SA, Cole GM. The curry spice curcumin reduces oxidative damage and amyloid pathology in an Alzheimer transgenic mouse. J Neurosci 2001;21:8370-7.
- Lim CS, Jin DQ, Mok H, Oh SJ, Lee JU, Hwang JK, et al. Antioxidant and anti-inflammatory activities of xanthorrhizol in hippocampal neurons and primary cultured microglia. J Neurosci Res 2005;82:831-8.