Electrophysiological characterization of a novel Kv channel blocker N,N’-[oxybis(2,1-ethanediyloxy-2,1-ethanediyl)]bis(4-methyl)-benzenesulfonamide found in virtual screening1
Introduction
Voltage-gated K+ (Kv) channels show enormous molecular diversity with approximately 40 pore-forming principal subunits identified that constitute 12 subfamilies (Kv1-Kv12)[1]. The heteromultimeric assembly of different subunits provides a base for further diversity and leads to a huge number of functionally diverse Kv channels with distinct biophysical, pharm-acological, and regulation properties[2]. Increasing evidence shows that the dysfunction of Kv channels is associated with epilepsy, cardiac arrhythmias, skeletal muscle disorders, neurodegenerative diseases, and other diseases[3–5]. Thus, the pharmacological modulation of Kv channels was proposed as a therapeutic strategy in the treatment of disorders[4,6].
High-throughput screening (HTS) technologies have been widely employed to search for drug candidates in pharmaceutical industries. In contrast to those successfully applied in screening enzyme inhibitors or ligands for the G-protein coupled receptor, HTS technologies for ion channel drugs remain a challenge. New methods, such as fluorescence-based assays, radioactive efflux assays, and radiolabeled-ligand binding assays, although representing “industry standards”, still rely on conventional patch-clamping recording for assessing the functional interaction of a compound with an ion channel[7,8]. Recently, a variety of prototypes of high-throughput electrophysiology has emerged; however, the throughput is generally low[8,9]. Virtual screening complements bioactivity screening. Nowadays, both ligand- and target-based virtual screening are used as reliable methods in the discovery of drug candidates in pharmaceutical industries[10,11]. Based on the crystal structure of the KcsA channel from Streptomyces lividans[12], we constructed a 3-D model of a eukaryotic Kv channel and developed a computational virtual screening approach to search large databases for novel Kv channel blockers[13,14]. As a result, we found nearly a dozen hit compounds in both the China Natural Products Database (CNPD) and the MDL Available Chemicals Directory (ACD) that potently inhibited voltage-activated K+ currents in rat hippocampal neurons.
Several hit compounds have been further characterized with respect to their potency, efficacy and specificity. Among them, OMBSA is the most potent hit compound found in the ACD database[14] (Figure 1). However, it remains unclear whether the hit compound inhibits voltage-activated K+ currents through the blocking of Kv channels, modification of gating, or shift of voltage dependence[15]. In the present study, the inhibitory effects of OMBSA on voltage-activated K+ currents in rat hippocampal neurons and the relevant mechanisms were further investigated by using a whole-cell voltage-clamp recording technique.
Materials and methods
Materials OMBSA (molecular weight 500.1, purity >99.5%) was synthesized in our laboratory. Other chemicals were purchased from Sigma-Aldrich China Inc (China).
Preparation of dissociated hippocampal neurons Sprague-Dawley rats (5–9 d old) were provided by the Shanghai Experimental Animal Center, Chinese Academy of Sciences (Shanghai, China). Dissociated hippocampal neurons were prepared as described previously[16]. Briefly, hippocampal slices (500 μm) were cut in oxygenated ice-cold dissociation solution containing (in mmol/L): 82 Na2SO4, 30 K2SO4, 5 MgCl2, 10 HEPES, and 10 glucose (pH 7.3 with NaOH). The slices were incubated in dissociation solution containing protease XXIII (3 g/L) at 32 oC for 8 min, and then placed in dissociation solution containing trypsin inhibitor type II-S (1 g/L) and bovine serum albumin (1 g/L) at 24–25 oC in an oxygen atmosphere. Before recording, the CA1 regions of several slices were dissected and triturated using a series of fire-polished Pasteur pipettes with decreasing tip diameters. Dissociated neurons were placed in a recording dish and superfused with an external solution containing the following (in mmol/L): 135 NaCl, 5 KCl, 1 CaCl2, 2 MgCl2, 10 HEPES, 10 glucose, and 0.001 tetrodotoxin (pH 7.4 with NaOH).
Whole-cell voltage-clamp recording Voltage-activated K+ currents were recorded in large, pyramidal-shaped neurons using an Axopatch 200A amplifier (Axon Instruments, CA, USA) at 24–25 oC. Voltage protocols were controlled by pClamp 9.0 software (Axon Instruments) via a DigiData-1322A interface (Axon Instruments). Recording electrodes (with a tip resistance of 3–5 MΩ) were pulled from borosilicate grass pipettes (Sutter Instruments, USA) and filled with a pipette solution containing the following composition (in mmol/L): 140 KCl, 2 MgCl2, 1 CaCl2, 10 HEPES, and 10 EGTA (pH 7.4 with KOH). Signals were filtered at 2–10 kHz and sampled at frequencies of 10–40 kHz. Series resistance was compensated by 75%–85%. Linear leak and residual capacitance currents were subtracted online using a P/4 protocol.
Drug application OMBSA was dissolved in DMSO to prepare a stock solution of 10 mmol/L and stored at –20°C. The stock solution was diluted to desired concentrations with the external solution before use and applied to the neuron using RSC-100 rapid solution changer with a 9-tube head (BioLogic, France). DMSO (less than 0.1% in the final dilution) had no observed effect on the voltage-activated K+ currents. For intracellular dialysis, OMBSA in the pipette solution was diffused into the recorded neuron immediately after the patch membrane ruptured[17].
Data analysis The peak amplitude of the fast transient K+ channel (IA) was measured, whereas the amplitude of the delayed rectifier channel (IK) was measured with a 300 ms latency. The decay time constants (τ) of the currents were obtained by fitting the decay time course with a mono-exponential function. The concentrations of OMBSA to yield a 50% block of the K+ currents (IC50) were obtained by fitting normalized concentration-inhibition relationships to the following equation:
I/I0=1/(1+[{C}/IC50]n),
where I0 and I are current amplitudes measured in the control and in the presence of OMBSA, C is the concentration of OMBSA in the external solution, and n is the Hill coefficient. For analyzing the voltage-dependence of steady-state activation or inactivation of the K+ currents, normalized conductance or current was plotted against the membrane potential and fitted to the Boltzmann equation:
Y=1/(1+exp[{V-V1/2}/k]),
where Y is the normalized conductance or current, V is the test potential, V1/2 is the voltage at half-maximal activation or inactivation, and k is the slope factor. The time course of recovery of the K+ currents from inactivation was fitted with a mono-exponential function:
I/Imax=A*(1-exp[-Δt/τ]),
where Imax is the maximal current amplitude, I is the current after a recovery period of Δt, τ is the time constant, and A is the amplitude coefficient. Data are presented as mean±SEM. Statistical significance was assessed using a Student’s t-test or one-way ANOVA as appropriate, and P<0.05 was considered significant. All analyses were performed using GraphPad Prism 4 (GraphPad Software, Inc, USA) and Excel 2000 software.
Results
Inhibition of voltage-activated K+ currents by OMBSA in hippocampal neurons As shown in the left panel of Figure 2A, both IK and IA were simultaneously recorded from a dissociated pyramidal neuron by using the voltage protocols and a subtraction procedure. Superfusion with OMBSA (30 μmol/L) remarkably reduced the amplitudes of both the voltage-activated K+ currents elicited by all depolarizing steps (middle panel of Figure 2A). The inhibitory effects had a rapid onset and reached steady-state levels within 10 s. The K+ currents recovered immediately upon washing out of the compound (Figure 2B, 2C).
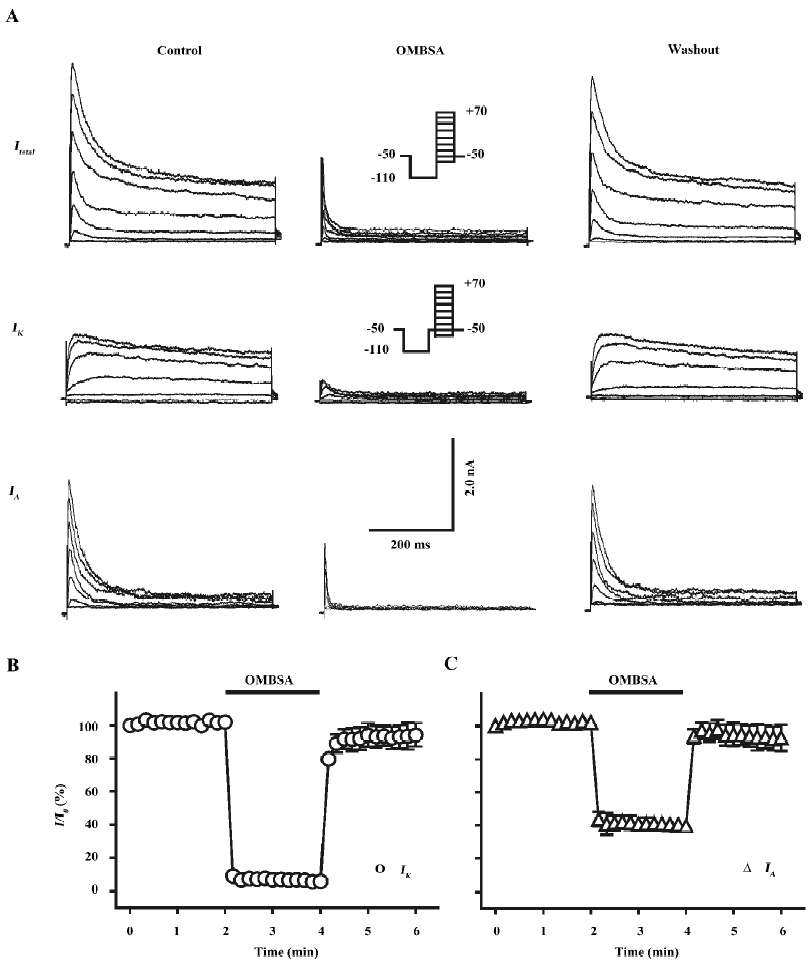
In addition to causing a reduction in the amplitude of the IK, superfusion with OMBSA (30 μmol/L) markedly accelerated the decay of the current (Figure 3A). The τ for IK was drastically reduced from 271.3±6.2 ms to 23.6±3.9 ms (n=6, P<0.01; Figure 3B). In contrast, OMBSA exerted a negligible effect on the decay time course of IA (Figure 3C). The value of τ for IA was reduced from 20.9±1.1 ms to 16.5±2.3 ms (n=6), but the change was statistically non-significant (P>0.05; Figure 3D). The results suggest that the compound differentially modulated the inactivation of K+ currents.
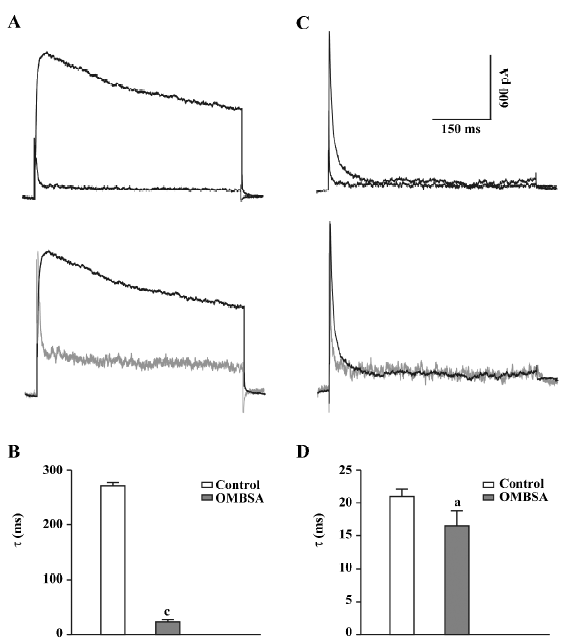
Analyses of the concentration-inhibition relationships of OMBSA have revealed that the compound preferentially inhibited IK to IA. As shown in Figure 4A, the threshold concentration for the inhibition of IK was between 0.1 and 1 μmol/L, and IK was reduced by approximately 90% at 100 μmol/L. In contrast, the threshold concentration for the inhibition of IA was between 1 and 10 μmol/L, and the amplitude of IA was reduced by approximately 70% at the maximal concentration tested (300 μmol/L). The IC50 value for the inhibition of IK was 2.1±1.1 μmol/L (Hill coefficient =0.94±0.16, n=6), and that for the inhibition of IA was 27.8±1.5 μmol/L (Hill coefficient =1.3±0.3, n=6).
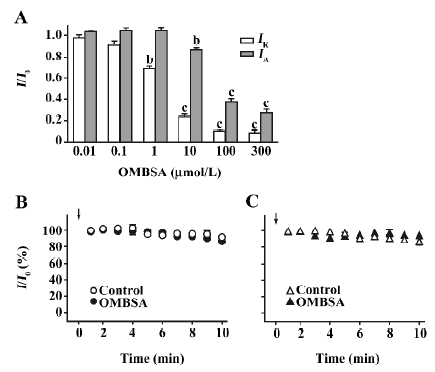
Lack of effects of intracellular dialysis of OMBSA on voltage-activated K+ currents In order to determine the action site of the compound on the Kv channels, the effects of intracellular dialysis of OMBSA on the K+ currents were investigated. The concentration for intracellular dialysis was 100 μmol/L, which inhibited IK and IA by 90% and 65%, respectively, when applied externally (Figure 4A). Throughout the 10 min recording period, the relative amplitudes of IK and IA in the neurons dialyzed with OMBSA were almost identical to those in the respective control groups (Figure 4B,4C). At the end of the 10 min recording, the relative amplitude of IK in the control and in the neurons dialyzed with OMBSA were 89.5%±0.1% and 86.3%±0.1%, respectively (n=6 for each, P>0.05); the relative amplitude of IA in the control and in the neurons dialyzed with OMBSA were 82.7%±0.1% and 92.3%±0.1%, respectively (n=7 for each, P>0.05). The results suggest that OMBSA acts at an extracellular site of the Kv channels.
Voltage-independent inhibition of voltage-activated K+ currents by OMBSA The compound was 13-fold more potent in the inhibition of IK than of IA. In order to cause comparable effect on the 2 types of K+ currents, concentrations of 3 and 30 μmol/L were used for studying IK and IA, respectively. The current-voltage (I/V) curves of IK in the control and in the presence of OMBSA (3 μmol/L) revealed that the compound did not change the threshold for the activation of IK (Figure 5A). OMBSA apparently caused a linear downward shift of the curve and reduced its amplitude over the entire range of activation.
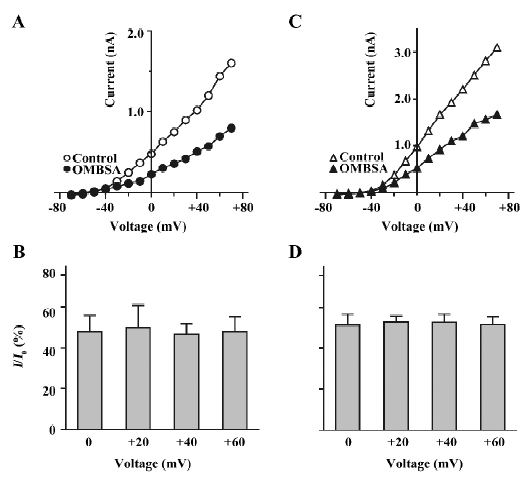
The relative amplitudes of IK elicited by depolarizing steps at 0, +20, +40 and +60 mV were 47.7%±8.0%, 49.9%±10.8%, 46.6%±5.1%, and 47.7%±7.6%, respectively (n=7, P>0.05, ANOVA; Figure 5B). Similar results were observed for the I/V curve of IA in the presence of OMBSA (30 μmol/L; Figure 5C). The relative amplitudes of IA elicited by depolarizing in steps at 0, +20, +40 and +60 mV were 51.4%±5.3%, 52.7%±3.0%, 52.6%±4.0% and 51.5%±4.0%, respectively (n=6, P>0.05, ANOVA; Figure 5D). These results demonstrated that the inhibition of IK and IA by OMBSA was voltage independent.
Effects of OMBSA on activation and steady-state inactivation of voltage-activated K+ currents in hippocampal neurons Superfusion of OMBSA (3 μmol/L) had no significant effect on the steady-state activation of IK, nor did it affect its steady-state inactivation and the time course of recovery from inactivation (Figure 6A,6C,6E). OMBSA (30 μmol/L) had no effect on the voltage dependence of the steady-state activation of IA, but resulted in a significant hyperpolarizing shift (nearly 8 mV) of its steady-state inactivation curve, and significantly slowed down its recovery from inactivation (Figure 6B,6D,6F). The effects of OMBSA on the kinetic parameters of IA and IK are summarized in Table 1.
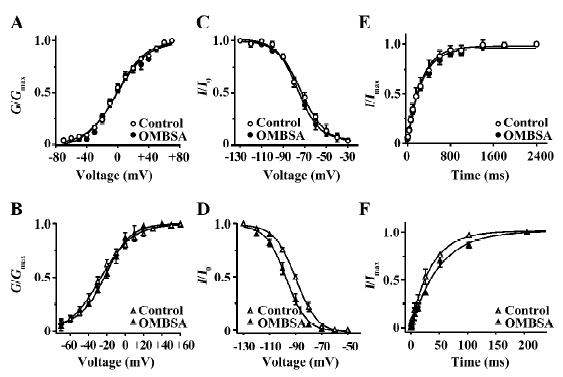
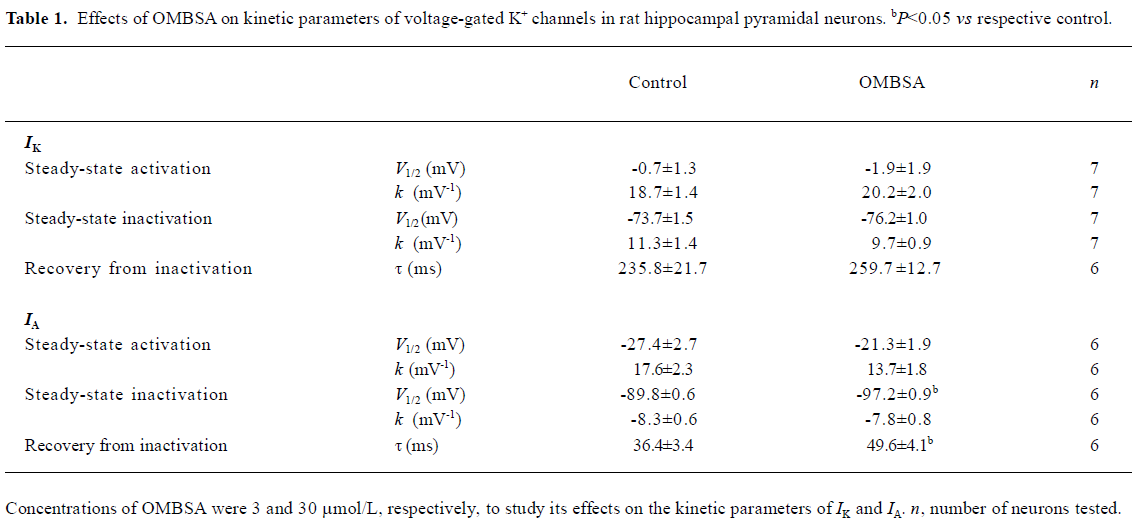
Full table
Discussion
Due to the lack of HTS techniques for ion channel drugs[7,9], virtual screening approaches have been developed for discovering new blockers of Kv channels[13,18]. In a virtual screening study[14], a comprehensive assessment of electrostatic and hydrophobic interactions with the Kv channel, and solvation free energy suggests OMBSA to be a hit compound with the most potent Kv channel-blocking activities in the ACD database.
In the present study, we showed that OMBSA potently inhibited both IK and IA in rat hippocampal neurons. The compound is 500-fold more potent than tetraethylammonium (TEA) in the inhibition of IK (the IC50 value of TEA was 1.05±0.21 mmol/L[19]), and approximately 180-fold more potent than 4-aminopyridine in the inhibition of IA (the IC50 value of 4-aminopyridine was 4.92±0.65 mmol/L, unpublished data). Furthermore, several interesting clues were found that seemed to be helpful in elucidating the interaction of the compound with Kv channels: (1) the onset of the inhibition and recovery from the inhibition were fast (Figure 2B,2C), suggesting that OMBSA rapidly binds to, and dissociates from the binding site on Kv channels; (2) the inhibition was voltage independent (Figure 5), which was similar to the blocking of IK by externally-applied TEA in hippocampal neurons[19]; and (3) intracellular dialysis of OMBSA was ineffective (Figure 4B,4C). The results demonstrate that the compound is most likely to act as a blocker at the outer mouth of the Kv channels, as predicted by the molecular docking model in the virtual screening[13,14]. A similar mechanism has been found for 14-benzoyltalatisamine, a hit compound found in the CNPD database, which selectively blocks IK through binding to its external pore entry with partial insertion into the selectivity filter[19].
It is also evident that the mode of actions of OMBSA on the 2 types of Kv channels differs: (1) OMBSA is 13-fold more potent in blocking IK than IA; (2) OMBSA may bind to IK with 1:1 stoichiometry (Hill coefficient =0.94±0.16), and to IA with 2:1 stoichiometry (Hill coefficient =1.3±0.3); and (3) in addition to acting as a channel blocker molecule, OMBSA differentially modulates the kinetics of the Kv channels; it markedly accelerated the inactivation of IK without significant effect on that of IA. Moreover, the compound did not change the activation, steady-state inactivation of IK, and its recovery from inactivation, but caused a significant hyperpolarizing shift of the voltage dependence of the steady-state inactivation of IA, and slowed down its recovery from inactivation, which led to fewer fast transient K+ channels available for activation.
In vitro studies have shown that loss of intracellular K+ ions through enhanced IK (mainly the Kv2.1 channel) mediates apoptosis of cultured cortical neurons induced by a variety of treatments, and the blocking of the Kv channel reduces neuronal death[20–22]. A number of in vivo studies also showed that transient forebrain ischemia resulted in a progressive increase of IK and a transient upregulation of IA in hippocampal CA1 pyramidal neurons that led to neuronal injury and programmed cell death[23,24], while blocking IK by TEA effectively promoted neuronal survival in the CA1 region[25,26]. Recently, the inhibition of an A-type transient K+ current was found to protect cerebellar granule cells against low K+/serum deprivation-induced apoptosis[27,28]. Because OMBSA potently blocks both IK and IA in hippocampal CA1 pyramidal neurons, it will be interesting to test whether the compound possesses neuroprotective effects.
References
- Gutman GA, Chandy KG, Adelman JP, Aiyar J, Bayliss DA, Clapham DE, et al. International Union of Pharmacology. XLI. Compendium of voltage-gated ion channels: potassium channels. Pharmacol Rev 2003;55:583-6.
- Coetzee WA, Amarillo Y, Chiu J, Chow A, Lau D, McCormack T, et al. Molecular diversity of K+ channels. Ann N Y Acad Sci 1999;868:233-85.
- Curran ME. Potassium ion channels and human disease: phenotypes to drug targets? Curr Opin Biotech 1998;9:566-72.
- Shieh CC, Coghlan M, Sullivan JP, Gopalakrishnan M. Potassium channels: molecular defects, diseases, and therapeutic opportunities. Pharmacol Rev 2000;52:557-93.
- Wickenden AD. K. + channels as therapeutic drug targets. Pharmacol Therapeut 2002;94:157-82.
- Zaks-Makhina E, Kim Y, Aizenman E, Levitan ES. Novel neuroprotective K+ channel inhibitor identified by high-throughput screening in yeast. Mol Pharmacol 2004;65:214-9.
- Xu J, Wang X, Ensign B, Li M, Wu L, Guia A, et al. Ion-channel assay technologies: quo vadis? Drug Discov Today 2001;6:1278-87.
- Worley J III. Guest editor’s introduction: an evolution of electrophysiology. Receptor Channel 2003;9:1-2.
- Willumsen NJ, Bech M, Olesen SP, Jensen BS, Korsgaard MPG, Christophersen P. High throughput electrophysiology: new perspectives for ion channel drug discovery. Receptor Channel 2003;9:3-12.
- Lyne PD. Structure-based virtual screening: an overview. Drug Discov Today 2002;7:1047-55.
- Shoichet BK. Virtual screening of chemical libraries. Nature 2004;432:862-5.
- Doyle DA, Cabral JM, Pfuetzner RA, Kuo A, Gulbis JM, Cohen SL, et al. The structure of the potassium channel: molecular basis of K+ conduction and selectivity. Science 1998;280:69-77.
- Liu H, Li Y, Song M, Tan X, Cheng F, Zheng S, et al. Structure-based discovery of potassium channel blockers from natural products: virtual screening and electrophysiological assay testing. Chem Biol 2003;10:1103-13.
- Liu H, Gao ZB, Yao Z, Zheng S, Li Y, Zhu W, et al. Discovering potassium channel blockers from synthetic compound database by using structure-based virtual screening in conjunction with electrophysiological assay. J Med Chem 2007;50:83-93.
- Hille B. Ionic channels of excitable membranes, 2nd ed. Sunderland Massachusetts: Sinauer Associates Inc; 1992.
- Yu Y, Chen XQ, Cui YY, Hu GY. Electrophysiological actions of cyclosporin A and tacrolimus on rat hippocampal CA1 pyramidal neurons. Acta Pharmacol Sin 2007;28:1891-7.
- Hu GY, Biro Z, Hill RH, Grillner S. Intracellular QX-314 causes depression of membrane potential oscillations in lamprey spinal neurons during fictive locomotion. J Neurophysiol 2002;87:2676-83.
- Pirard B, Brendel J, Peukert S. The discovery of Kv1.5 blockers as a case study for the application of virtual screening approaches. J Chem Inf Model 2005;45:477-85.
- Song MK, Liu H, Jiang HL, Yue JM, Hu GY. Electrophysiological characterization of 14-benzoyltalatisamine, a selective blocker of the delayed rectifier K+ channel found in virtual screening. Eur J Pharmacol 2006;531:47-53.
- Yu SP, Yeh CH, Sensi SL, Gwag BJ, Canzoniero LMT, Farhangrazi ZS, et al. Mediation of neuronal apoptosis by enhancement of outward potassium current. Science 1997;278:114-7.
- Yu SP, Farhangrazi ZS, Ying HS, Yeh CH, Choi DW. Enhancement of outward potassium current may participate in β-amyloid peptide-induced cortical neuronal death. Neurobiol Dis 1998;5:81-8.
- Pal S, Hartnett KA, Nerbonne JM, Levitan ES, Aizenman E. Modulation of neuronal apoptosis by Kv2.1-encoded potassium channels. J Neurosci 2003;23:4798-802.
- Chi XX, Xu ZC. Differential changes of potassium currents in CA1 pyramidal neurons after transient forebrain ischemia. J Neurophysiol 2000;84:2834-43.
- Zou B, Li Y, Deng P, Xu ZC. Alterations of potassium currents in ischemia-vulnerable and ischemia-resistant neurons in the hippocampus after ischemia. Brain Res 2005;1033:78-89.
- Huang H, Gao TM, Gong LW, Zhuang ZY, Li X. Potassium channel blocker TEA prevents CA1 hippocampal injury following transient forebrain ischemia in adult rats. Neurosci Lett 2001;305:83-6.
- Wei L, Yu SP, Gottron F, Snider BJ, Zipfel GJ, Choi DW. Potassium channel blockers attenuate hypoxia- and ischemia-induced neuronal death in vitro and in vivo. Stroke 2003;34:1281-6.
- Hu CL, Liu Z, Gao ZY, Zhang ZH, Mei YA. 2-Iodomelatonin prevents apoptosis of cerebellar granule cells via inhibition of A-type transient outward K+ currents. J Pineal Res 2005;38:53-61.
- Hu CL, Liu Z, Zheng XM, Liu ZQ, Chen XH, Zhang ZH, et al. 4-Aminopyridine, a Kv channel antagonist, prevents apoptosis of rat cerebellar granule neurons. Neuropharmacology 2006;51:737-46.