Genistein stimulates osteoblastic differentiation via p38 MAPK–Cbfa1 pathway in bone marrow culture1
Introduction
Genistein, a soybean phytoestrogen, has estrogen-like activity in mammals, including anabolic effects on bone through mechanisms that remain to be elucidated[1]. Several studies have shown that the incidence of mammary and other cancers is inversely related to the dietary intake of soy containing genistein and other phytoestrogens[2]. Unlike endogenous hormone 17β-estradiol (E2), genistein lacks stimulation on mammary glands and uteri, which makes it a possible alternative to estrogen replacement therapy for osteoporosis after menopause. While public and scientific interest in genistein and other phytoestrogens is growing, their mechanism of action on bone cells has largely remained unclear.
Core binding factor 1 (Cbfa1), belonging to the runt domain transcription factor family, is shown to be a master regulator of osteoblastic differentiation. Komori et al[3] observed that the gene-targeted disruption of osteoblast-specific Cbfa1 in the in vivo mouse model blocked skeleto-genesis, and that heterozygous mutations of Cbfa1 in both humans and mice led to cleidocranial dysplasia, an autosomal dominant disorder. As further evidence of its role in differentiation, Ducy et al[4] showed that the forced expression of Cbfa1 induced the expression of principal osteoblast-specific genes, such as bone sialoprotein, osteopontin, and osteocalcin in non-osteoblastic cells. Cbfa1 also plays an important role in mouse bone marrow-derived mesenchymal stem cells (BMSC), becoming the osteoblastic lineage in vivo and in vitro.
p38 Mitogen-activated protein kinase (MAPK) is a conserved subfamily of MAPK involved in inflammatory response, stress response, cell growth and survival, as well as differentiation of a variety of cell types. Several studies have suggested that p38 MAPK is involved in osteoblastic differentiation[5]. Our previous work has also shown that exposure of BMSC to SB203580, the inhibitor of p38 MAPK, resulted in reduced alkaline phosphatase (ALP) activity (a marker of early osteoblastic differentiation) and calcium deposition (a marker of late osteoblastic maturation)[6]. Because the p38 MAPK pathway and Cbfa1 are essential for osteoblastic differentiation, we inferred that the anabolic effect of genistein might be through the p38 MAPK pathway and activates the osteoblastic-specific transcription factor, Cbfa1. In the present study, we examined the role of p38 MAPK and Cbfa1 activity in mediating the effects of genistein on osteoblastic differentiation by using mouse BMSC cultures, a population of pluripotent cells within the bone marrow microenvironment which has a capacity to undergo osteoblastic differentiation and mineralization in vitro.
Materials and methods
Chemicals Alpha minimum essential medium (α-MEM), fetal bovine serum (FBS), trypsin-EDTA, penicillin–streptomycin solution, and SDS were obtained from Gibco-BRL (Grand Island, NY, USA). The phosphorylated p38 MAPK assay kit, SB203580 was purchased from New England Biolabs (Beverly, MA, USA). The TRIzol total RNA isolation kit and Prime-It II random primer labeling kit were obtained from Stratagene (La Jolla, CA, USA). Anti-NF-κB antibody and anti-Cbfa1 antibody were purchased from Santa Cruz Biotech, Inc (Santa Cruz, CA, USA). Other regents, antibodies, and reagent kits were purchased from Sigma Chemical Co (St Louise, MO, USA).
Cell culture The bone marrow cells were obtained from 8- to 10-week-old female mice of the Kunming strain (Experimental Animal Center of Xiangya Medical College, Central South University, Changsha, China), as previously described[6]. Briefly, the femurs and tibias were dissected, the ends of the bones were cut, and the marrow was flushed out with 2 mL of ice-cold α-MEM containing 10% FBS (v/v) by using a needle and syringe. A suspension of bone marrow cells was obtained by repeated aspiration of the cell preparation through a 22 gauge needle and then the cells were counted with a hemocytometer. The cells were seeded onto 12-well plates or 60 mm plates at a density of 1×107 cells/mL and cultured for 5 d in α-MEM supplemented with 15% FBS (v/v), 100 U/mL sodium penicillin G, and 100 mg/L streptomycin sulfate in a humidified incubator with 5% CO2 and 95% air at 37 °C. On d 5, all non-adherent cells were removed with the first medium change and then the adherent cells (representing BMSC) were grown for additional periods of up to 12 d in the differentiation medium (phenol red-free α-MEM containing 10% FBS [v/v] supplemented with 5 mmol/L β-glycerophosphate and 25 mg/L ascorbic acid) for inducing the osteoblastic differentiation of BMSC. In the subsequent experiments, the beginning day of culture in the differentiation medium was defined as d 0. The genistein (1 μmol/L) and genistein (1 μmol/L) concurrent with SB203580 (1 μmol/L) were respectively added every 2 d and the medium was replaced every 4 d thereafter.
ALP Cellular ALP activity was assayed according to our previous study[7]. At the completion of the incubation period, the cells were harvested after removing the medium, washed twice with phosphate-buffered saline (PBS), and treated for 10 min with 0.25% trypsin to detach the cells. Enzyme activity was determined colorimetrically after incubation at 37 °C for 30 min with p-nitrophenylphosphate as the substrate at pH 10.3; the optical density was read at 400 nm. The total protein content was measured by the method of Bradford.
Quantitation of calcium deposition The calcification of BMSC was assessed by a modification of the Wada procedure[8]. The cultures were decalcified with 0.6 mol/L HCl for 24 h. The calcium content was determined by measuring the concentrations of calcium in the HCl supernatant by the o-cresolphthalein complexone method. After decalcification, the cultures were washed with PBS and solubilized with 0.1 mol/L NaOH/0.1% SDS. Total protein content was measured by the method of Bradford. The calcium content of the cell layer was normalized to the protein content.
Electrophoretic mobility shift assay Nuclear extracts were prepared from BMSC treated with vehicle alone or genistein in the presence or absence of 1 μmol/L SB203580. Synthetic double-strand oligonucleotides of consensus Cbfa1 binding sequence 5'-AGCTGCAATCACCAACCACAGCA-3' was end-labeled with (γ–32P)ATP using the T4 polynucleotide kinase. Nuclear extracts were incubated with binding buffer containing 20 mmol/L Tris-HCl (pH 8), 10 mmol/L NaCl, 3 mmol/L EDTA, 0.05% Nonidet P-40, 5 mmol/L dithiothreitol, 5% glycerol, and 1 mg poly (dI-dC) for 15 min, after which the labeled probe was added and incubated for an additional 15–20 min. The samples were subjected to electrophoresis at room temperature on chilled 5% polyacrylamide gel. For experiments using the anti-Cbfa1 antibody, the antibody was added to the reaction mixture for 30 min prior to the addition of the labeled probe. The samples were subjected to electrophoresis at room temperature on 5% polyacrylamide gel, which was dried and visualized by autoradiography.
RT-PCR Total RNA was extracted from cells using the TRIzol total RNA isolation kit (Stratagene, La Jolla, CA, USA). The RNA isolated from 8 d cultured cells was used for the analyses of ALP and bone sialoprotein (BSP) expression, whereas RNA isolated from 12 d cultured cells was used for the analyses of osteocalcin (OC) expression. The RNA (3 μg) was reverse-transcribed in 50 μL reaction buffer containing 0.5 mmol/L each of dNTP, 50–80 units of RNase block, 50 units of Moloney murine leukemia virus reverse transcri-ptase, and 750 ng oligo(dT) for 90 min at 37 °C.
PCR using primers specific for ALP and BSP (the early makers of osteoblastic differentiation), OC (the late osteogenic marker), and GAPDH (internal control) were carried out in a volume of 10 μL with 1×Pfu polymerase buffer, 190 μmol/L dNTP, 28 ng of each primer, 0.45 units Pfu polymerase, 0.18 μCi (α-32P)dCTP, and 1.0 μL of the template (from the above 50 μL RT reaction). Thermal cycling was carried out for 21 cycles (GAPDH) or 30 cycles (ALP, BSP, and OC) at 62 °C, annealing for ALP, BSP, and GAPDH, and 72 °C annealing for OC. The amplified fragments were isolated on polyacrylamide gel (29:1 acrylamide to bis-acrylamide) and the autoradiographs were scanned with the Gel Doc 2000 system (Bio-Rad, Hercules, CA, USA). The primers used for the PCR amplification were designed by computer assistance according to GeneBank:
ALP: forward, 5'-CTTGCTGGTGGAAGGAGGCAGG-3' and reverse, 5'-CACGTCTTCTCCACCGTGGGTC-3'; BSP: forward, 5'-CTCGGGTGTAACAGCTAGCTAC-3' and reverse, 5'-CGTTCAGAAGGACAGCTGTCTG-3'; OC: forward, 5'-CT-CTGTCTCTCTGACCTCACAG-3' and reverse, 5'-GGAGCT-GCTGTGACATCCATAC-3'; and GAPDH: forward, 5'-TGGC-ACAGTCAAGGCCTGAGA-3' and reverse, 5'-CTTCTGA-GTGGCAGTGATGG-3'.
Northern blot analysis The BMSC grown in the 60 mm dishes were treated at subconfluence with vehicle alone or genistein in the presence or absence of 1 μmol/L SB203580 for the indicated period, and total RNA was isolated using TRIzol total RNA isolation kit. The RNA isolated from the 8 d cultured cells were used for the analyses of osteopontin (OPN) expression, whereas the RNA isolated from the 4 d cultured cells were used for the analyses of Cbfa1 expression. The total RNA (10 μg) samples were separated on 1% agarose gels and transferred overnight to nitrocellulose membranes, which were cross-linked with UV light. The membranes were hybridized overnight at 58 °C with the 32P-labeled probe prepared according to the protocols of the Prime-It II random primer labeling kit (Stratagene). The membranes were washed twice at room temperature for 20 min with 2× SSC (1×SSC=150 mmol/L NaCl, 15 mmol/L Na citrate, pH 7.0) with 0.2% SDS and twice at 58 °C for 20 min with 1×SSC with 0.2% SDS before autoradiography. After stripping with 0.1×SSC with 0.1% SDS, the same membranes were probed with mouse 28S rRNA using 55 °C hybridization overnight and washed with 2×SSC with 0.1% SDS for 5 min at room temperature once and 0.5×SSC with 0.1% SDS for 30 min twice at 55 °C.
Western blot analysis Western blotting was carried out by using the phosphorylated p38 MAPK assay kit according to the manufacture’s protocol. Briefly, the cells were lysated in SDS sample buffer (62.5 mmol/L Tris-HCl, 2% SDS, 10% glycerol, and 50 mmol/L dithiothreitol). After being sonicated and denatured by boiling for 5 min, the protein concentration was measured by the method of Bradford. 25 μg of proteins were separated on 10% SDS–PAGE and transferred to a polyvinylidene difluoride membrane (Millipore Corp, Bedford, MA, USA). Non-specific binding sites on the membrane were blocked for 1 h in blocking buffer (50 mmol/L Tris-HCl, 0.1% Tween 20, and 5% non-fat milk). The membrane was exposed to a primary monoclonal antibody of phosphorylated p38 MAPK or non-phosphorylated p38 MAPK (1:1000 dilution) overnight at 4 °C and then exposed to the second antibody (horseradish peroxidase-conjugated, 1:2000 dilution) for 1 h. Detection was performed with an enhanced chemiluminescence (ECL) reagent.
For the detection of the expression level of Cbfa1, nuclear extracts (15 μg) prepared from cells at d 8 were separated on 10% SDS–PAGE and electrotransferred to a PVDF membrane. The blots were probed with an anti-Cbfa1 antibody at a dilution of 1:500 for 2 h at room temperature. The Cbfa1 protein was detected by ECL according to manufacturer’s recommendations (Amersham Pharmacia Biotech, Tokyo, Japan).
Statistical analysis The data were the mean values of at least 3 different experiments and expressed as mean±SD. Student’s t-test was used to compare data. P<0.05 was considered statistically significant.
Results
Effects of genistein on p38 MAPK activation The exposure of BMSC to genistein (1 μmol/L) resulted in the rapid and sustained activation of p38 MAPK, which reached its maximal activation at 5 min and even lasted for 360 min after exposure (Figure 1A). The genistein-induced activation of p38 MAPK was in a dose-dependent manner and 1 μmol/L genistein was found to give the maximal response (Figure 1B). The activation of p38 MAPK was significantly attenuated when genistein was incubated with 1 μmol/L of p38 MAPK inhibitor SB203580 (Figure 1C).
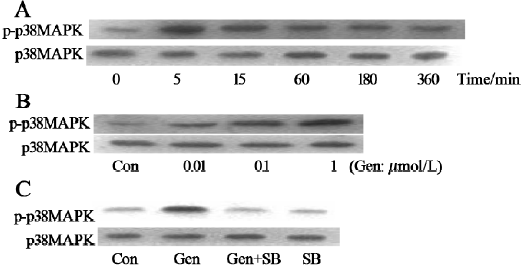
Effects of genistein on osteoblastic differentiation and mineralization The BMSC were continuously cultured with vehicle or genistein (1 μmol/L), and ALP activity and calcium deposition were measured over a period of 0, 4, 8, and 12 d. Genistein markedly increased ALP activity and the calcium deposition of the BMSC culture as a function of cultured time compared with the control (Figure 2). The increased ALP activity and calcium deposition could be abolished by concurrent treatment with SB203580 (Figure 2). There were slight, but significant reductions on ALP activity and calcium deposition when SB203580 was used alone.
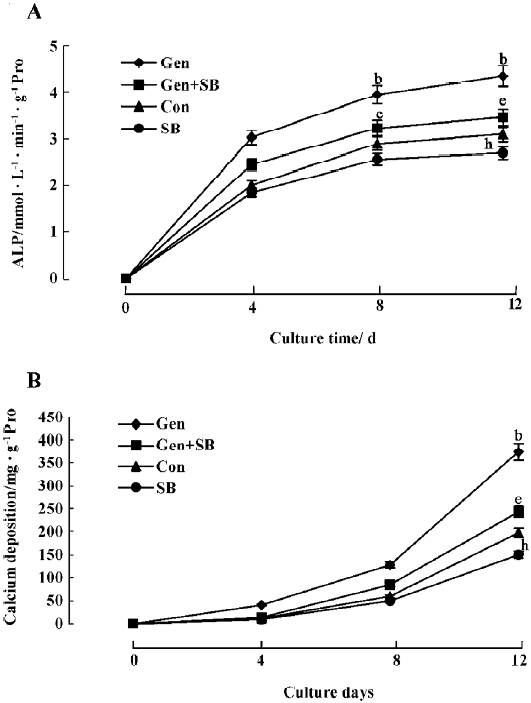
Effects of genistein on Cbfa1 DNA binding activity Cbfa1-consensus DNA binding activity was markedly increased in the genistein-treated cells compared with the untreated control. However, the DNA binding activity was diminished in the presence of the p38 MAPK inhibitor (Figure 3A). The addition of the anti-Cbfa1 antibody resulted in an upper super-shifted band and a decrease in the lower binding complex, indicating that the binding was apparently specific to Cbfa1, since an irrelevant antibody(anti-NF-κB antibody) had no effect (Figure 3B).
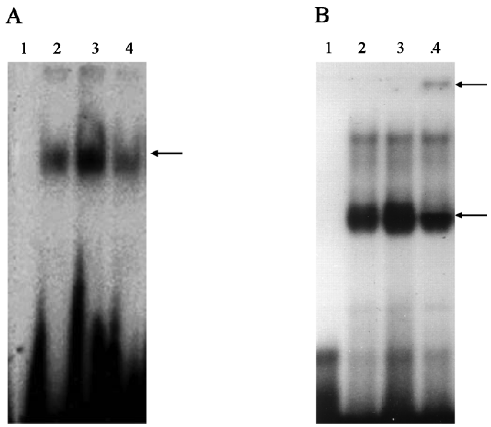
Effects of genistein on the transcription of osteoblastic differentiation genes The RT-PCR analysis showed that the expression of ALP and BSP (the earlier makers of osteoblastic differentiation at d 8) and OC (the later osteogenic marker at d 12) were upregulated in genistein-treated BMSC cultures. In contrast, SB203580 clearly inhibited the genistein-mediated osteoblastic gene expression (Figure 4).
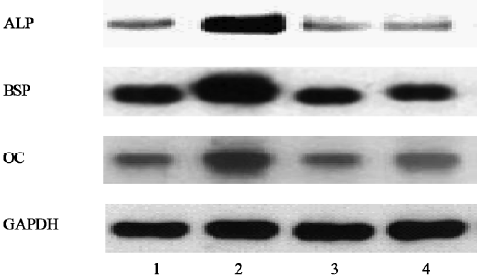
Effects of genistein on Cbfa1 expression The Western blot analysis showed that the protein level of Cbfa1, detected as 2 immunoreactive species of 60 and 65 kDa sizes, was significantly increased in the genistein-treated samples compared with the control. The increased Cbfa1 protein level was also reduced by SB203580 treatment (Figure 5A). The Northern blot analysis showed that the mRNA levels of Cbfa1 at d 4 and OPN at d 8 were markedly upregulated in the genistein-treated cells. Again, SB203580 treatment clearly inhibited the genistein-mediated osteoblastic Cbfa1 and its downstream gene OPN gene expressions (Figure 5B).

Discussion
In the present study, we utilized mouse BMSC to test the hypothesis that genistein can stimulate osteoblastic differentiation through the p38 MAPK–Cbfa1 pathway. The results of our experiments demonstrated that: (i) the incubation of mouse BMSC with genistein resulted in enhanced osteoblastic differentiation as evidenced by increased ALP activity and calcium deposition. At the same time, genistein treatment led to the activation of the p38 MAPK pathway and increased Cbfa1 message as well as the DNA binding ability; (ii) the increased Cbfa1 DNA binding correlated with the activation of several downstream Cbfa1-regulated genes, including ALP, BSP, OPN, and OC; and (iii) the genistein-mediated increase of Cbfa1 DNA binding enhanced osteoblastic differentiation, and induced Cbfa1-regulated gene expressions were remarkably attenuated by SB203580 treatment. These data provide the first evidence that genistein is capable of increasing Cbfa1 activity through the p38 MAPK pathway, which is responsible for its induction of osteoblastic differentiation in BMSC cultures. This finding has important ramifications for the therapeutic potential of genistein as an anti-osteoporosis agent.
Several studies have focused attention on the effect of genistein on the MAPK pathway and reached different conclusions. Kansra et al[9] found that the exposure of intact platelets to genistein resulted in the activation of the p44/42 MAPK pathway, which was due to the inhibition of basal tyrosine kinase. Frey et al[10] demonstrated that the p38 MAPK pathway was involved in genistein-inhibited cell proliferation. In addition, some previous studies have shown that at low concentrations (<=1 μmol/L), genistein has ER (estrogen receptor)-dependent effects on osteogenesis, the effects are similar to those of E2. At a higher concentration (>10 μmol/L), however, genistein has anti-estrogenic actions, namely, it downregulates osteogenesis, which is opposite to the E2-induced effects[11]. In our current study, genistein showed a dose-dependent effect (0.01–1 μmol/L) on the activation of p38 MAPK, and 1 μmol/L genistein was found to give maximal stimulation, so genistein at a concentration of 1 μmol/L was used for further investigation in this study. The specific inhibitor of the p38 MAPK kinase, SB203580, which has little effect on other kinases, including the other members of the MAPK family, was used to block the activation of p38 MAPK. Our data show that SB203580 was able to block genistein-mediated osteoblastic differen-tiation, suggesting that p38 MAPK activation is critical for genistein-induced osteoblastic maturation. We also found that genistein does not profoundly affect ERK (extracellular signal-regulated kinase) activity, another important member of the MAPK pathway. PD98059, the specific inhibitor of the ERK kinase had no effect on osteoblastic differentiation in mouse BMSC cultures, suggesting that the ERK pathway is unlikely to be involved in genistein-induced osteoblastic differentiation (data not shown). In fact, our previous work has demonstrated that ERK is important in cell proliferation and genistein has little or no effect on the ERK pathway[6].
Since Cbfa1 is a master regulator of osteoblastic differen-tiation, we examined the effect of genistein on Cbfa1 activity. Our results showed that Cbfa1 was activated by genistein at both the mRNA and protein levels, which are associated with observed increased DNA binding activity. We noticed that the regulation of mRNA occurred at the earlier stage (d 4) of the culture period, while the protein level of Cbfa1 did not change until the later stage (d 8) of the culture period. One possible explanation for this is that the increased protein level is a result of the increased mRNA level induced by genistein in the earlier stage. Similar regulation of Cbfa1 at the mRNA level has also been reported with 1,25(OH)2-D3 and BMP(bone morphogenetic protein) treatment in osteoblastic cells[4].
In order to examine the connection between the increased Cbfa1 activity and the activation of the p38 MAPK pathway in genistein-treated cells, we used SB203580 to block the activation of the p38 MAPK pathway and then check the Cbfa1 activity. Our results showed that the increased expression of Cbfa1 (both at the transcription and expression levels) by genistein was significantly attenuated by SB203580 treatment. Moreover, the inhibitory effect of SB203580 also affected the expression of several downstream Cbfa1-regulated genes induced by genistein, including ALP, BSP, OPN, and OC suggesting that p38 MAPK activation is upstream of Cbfa1 activity. Similar results have been reported by Jadlowice et al[12], which showed that p38 MAPK is involved in the gene expression levels of Cbfa1 and osteoblastic differentiation of human adult mesenchymal stem cells by phosphophoryn, a non-collagenous dentin ECM (extracel-lular matrix) protein and SIBLING (small integrin-binding ligand glycoprotein) protein family member. The p38 MAPK pathway is a major point of convergence for a variety of intracellular signals initiated by the growth/differentiation factor binding to receptor tyrosine kinases and mechanical stimulation. In addition, there is a considerable cross-talk between this pathway and other signaling events, such as those mediated by protein kinase C[13] and BMP[14].
Finally, our data indicate that genistein stimulates osteoblastic differentiation through the p38 MAPK–Cbfa1 pathway. These results provide new insight into the mechanistic basis of osteoblastic differentiation by drugs such as genistein.
References
- Anderson JJ, Garner SC. Phytoestrogens and bone. Baillieres Clin Endocrinol Metab 1998;12:543-57.
- Dixon-Shanies D, Shaikh N. Growth inhibition of human breast cancer cells by herbs and phytoestrogens. Oncol Rep 1999;6:1383-7.
- Komori T, Yagi H, Nomuras S, Yamaguchi A, Sasaki K, Deguchi K, et al. Targeted disruption of Cbfa1 results in a complete lack of bone formation owing to maturational arrest of osteoblasts. Cell 1997;89:755-64.
- Ducy P, Zhang R, Geoffroy V, Ridall AL, Karsenty G. Osf2/Cbfa1: a transcriptional activator of osteoblast differentiation. Cell 1997;89:747-54.
- Suzuki A, Guicheux J, Palmer G, Miura Y, Oiso Y, Bonjour JP, et al. Evidence for role of p38MAP kinases in expression of alkaline phosphatase during osteoblastic cell differentiation. Bone 2002;30:91-8.
- Liao QC, Xiao ZS, Qin YF, Zhao Y, Pan W, Liu T, et al. p44/42 and p38 MAPKs exert differences functions in osteoblastic differentiation of mouse bone marrow-derived mesenchymal stem cell cultures. Chin J Osteoporos 2004;10:267-71. (in Chinese).
- Xiao ZS, Quarles LD, Chen QQ, Yu YH, Qu XP, Jiang CH, et al. Effect of asymmetric dimethylarginine on osteoblastic differentiation. Kidney Int 2001;60:1699-704.
- Wada T, Mckee MD, Steitz S, Giachelli CM. Calcification of vascular smooth muscle cell cultures: inhibition by osteopontin. Circ Res 1999;84:166-78.
- Kansra SV, Reddy MA, Weng Y-I, Shivendra D. Activation of mitogen activated protein kinase in human platelets by genistein. Pharmacol Res 1999;39:21-31.
- Frey RS, Singletary KW. Genistein activates p38 mitogen-activated protein kinase, inactivates ERK1/ERK2 and decreases Cdc25C expression in immortalized human mammary epithelial cells. J Nutr 2003;133:226-31.
- Dang ZC, Audinot V, Papapouls SE, Boutin JA, Lowik CW. Peroxisome proliferator-activated receptor g (PPAR g) as a molecular target for the soy phytoestrogen genistein. J Biol Chem 2003;278:962-7.
- Jadlowiec J, Koch H, Zhang X, Campbell PG, Seyedain M, Sfeir C. Phosphophoryn regulates the gene expression and differentiation of NIH3T3, MC3T3-E1, and human mesenchymal stem cells via the integrin/MAPK signaling pathway. J Biol Chem 2004;279:53323-30.
- Nakajima K, Tohyama Y, Kohsaka S, Kurihara T. Protein kinase C alpha requirement in the activation of p38 mitogen-activated protein kinase, which is linked to the induction of tumor necrosis factor alpha in lipopolysaccharide-stimulated microglia. Neurochem Int 2004;44:205-14.
- Scherberich A, Tucker RP, Degen M, Brown-Luedi M, Andres AC, Chiquet-Ehrismann R. Tenascin-W is found in malignant mammary tumors, promotes alpha8 integrin-dependent motility and requires p38MAPK activity for BMP-2 and TNF-alpha induced expression in vitro. Oncogene 2005;24:1525-32.