High-throughput screening of novel antagonists on melanin-concentrating hormone receptor-11
Introduction
Melanin-concentrating hormone (MCH) is a 17-amino acid cyclic peptide originally isolated and sequenced from the salmon pituitary in 1983. It was regarded as a regulator of the pigmentary changes in background adaptation[1]. Rat MCH, purified from the hypothalamus, is identical to that of humans, and is 19 amino acids in length, 2 amino acids longer than its salmon counterpart[2]. In mammalian species, MCH is predominately synthesized in 2 brain centers, namely the perikarya of the lateral hypothalamus and zona incerta, and becomes widely distributed throughout the central nervous system (CNS). The extensive terminal distribution suggests that MCH may serve as a neurotransmitter or modulator in regulating brain functions[3]. Previous studies have revealed that the biological effects of MCH are complex, including feeding and energy balance[4-6], sexual behavior[7], stress responses[8], neuroendocrine functions[9-11], anxiety[7,12], seizure[13], memory and/or learning[14,15], grooming and locomotor activities[16], and arousal regulation[17].
In 1999, 5 independent groups almost simultaneously reported the identification of a MCH receptor [MCHR; MCHR-1, MCH-1R, MCH1, MCH1, or SLC-1 (orphan somatostatin-like receptor 1)] through a reverse pharmacology approach[18-22]. A second MCH receptor (MCHR-2, MCH-2R, MCH2, MCH2, or SLT) was identified subsequently based on the sequence homology to MCHR-1[23-27]. However, the functions of MCHR-2 remain unclear at present due to its species-specific expression and the lack of non-rodent in vivo models for behavioral studies[28,29]. Therefore, the focus of the current research is directed towards MCHR-1.
The stimulation of MCHR-1 leads to the elevation of the intracellular Ca2+ level, inhibition of forskolin-stimulated cyclic adenosine monophosphate (cAMP) production, and activation of the mitogen-activated protein kinase cascade, indicating that MCHR-1 is a G-protein-coupled receptor (GPCR) linked to both Gi/o and Gq proteins[30]. MCHR-1 is different from the limited expression of MCH in the lateral hypothalamus and zona incerta, as it is widely distributed throughout the CNS, including the cerebral cortex, caudate-putamen, hippocampal formation, subiculum, the shell of the nucleus accumbens, amygdala, hypothalamus and thalamus, the locus coeruleus of the brainstem, various nuclei of the mesencephalon and rhombencephalon, as well as most anatomical areas implicated in the control of olfaction, with the exception of the main olfactory bulb[31,32]. Based upon a series of studies using MCHR-1 antagonists and knock-out mice lacking MCHR-1, it has been established that MCHR-1 mediates feeding behavior and energy expenditure[33-35]. Experimental evidence also demonstrates that MCHR-1 plays an important role in the regulation of mood and stress responses. Furthermore, the pharmacological properties of MCHR-1 antagonists in rodent models of stress-related disorders (such as depression and anxiety) have been elucidated[35,36].
The discovery of the MCH receptors has prompted pharmacological scientists and behavioral biologists to evaluate their potential therapeutic applications. Both peptidic and non-peptidic MCHR-1 ligands have been reported, and the effects of more than 50 MCH analogs have been investigated to understand their structure–activity relationships (SAR)[37]. Intracerebroventricular injection of these MCH analogs led to a rapid and significant increase in food intake, the efficacy of which was strongly correlated with their potency at MCHR-1[38]. The results of this study clearly suggest that MCHR-1 mediates the orexigenic effects of MCH[38]. Some non-peptidic MCHR-1-selective antagonists have been identified, such as T-226296 and SNAP7941, both of which have anorectic effects[35,39].
The scintillation proximity assay (SPA) uses homogeneous and radioisotopic technology that does not involve post-reaction liquid handling steps, and is well-suited to automation and high-throughput screening (HTS)[40]. In the SPA system, membranes that express a particular receptor are attached to a microbead coated with wheat germ agglutinin. An isotope (e.g. [3H]) is brought very close to the scintillant-impregnated microbead by binding to its surface. Because the emitted β particles can only travel short distances in the bulk solution, the microbead preferentially captures electrons from the bound radiolabeled ligand. The amount of light emitted from the scintillant in the microbead is thus directly proportional to the amount of bound radiolabeled ligands. In this study, we describe a SPA-based HTS campaign involving a diverse library of 48 240 synthetic and natural compounds. Using the SPA technology, human MCHR-1 (hMCHR-1) binding affinities were determined with [3H]SNAP7941 competitive displacement. The functionality (agonist or antagonist activities) was subsequently assessed by a guanosine 5' (γ-[35S]thio) triphosphate ([35S]GTPγS) binding assay[41]. As a result, a novel hMCHR-1 antagonist was discovered together with a series of ligands previously unreported for this receptor.
Materials and methods
Reagents The radioligand [3H]SNAP7941 (specific activity: 2.26 TBq/mmol) and [35S]GTPγS (specific activity: 37 TBq/mmol) were purchased from Amersham (Buckinghamshire, UK). FlashBlue GPCR beads were obtained from Perkin–Elmer (Boston, MA, USA). Guanosine diphosphate (GDP), GTPγS, saponin, HEPES, glutamine, bovine serum albumin (BSA), and MCH are the products of Sigma–Aldrich (St Louis, MO, USA). Fetal bovine serum (FBS) was bought from Hyclone (Logan, UT, USA). Dulbecco’s modified Eagle’s medium (DMEM) with high glucose was the product of GIBCO BRL (Grand island, NY, USA). SNAP7941 was provided by Servier (Neuilly-sur-Seine, France).
Membrane preparation Human embryonic kidney cells (HEK293) stably expressing the hMCHR-1 receptor were cultured in DMEM with high glucose supplemented with 10% FBS, 2 mmol/L glutamine, 1×105 IU/L penicillin, 100 mg/L streptomycin, and 400 mg/L G418. The cells were grown at confluence, harvested in phosphate-buffered saline, and centrifuged at 1000×g for 5 min (4 °C). The resulting pellet was suspended in isotonic buffer (5 mmol/L Tris/HCl, 0.2 mmol/L MgCl2, and 0.25 mol/L sucrose, pH 7.4) and homogenized using the BioNeb Cell Disruption System (Terre Haute, IN, USA). The homogenate was then centrifuged (20 000×g for 30 min at 4 °C), and the resulting pellet was resuspended in the binding buffer 1 (50 mmol/L Tris/HCl, 120 mmol/L NaCl, 5 mmol/L KCl, 1 mmol/L MgCl2, 2.5 mmol/L CaCl2, pH 7.4). Protein content was determined using the Bradford assay[42]. Aliquots of membrane preparations were stored at –80 °C until use.
Homogeneous hMCHR-1 binding assay The membranes were incubated overnight in binding buffer 1 containing 2.5 nmol/L [3H]SNAP7941, 0.75 g/L FlashBlue GPCR beads, various titrations of SNAP7941 from a stock solution of 40 μmol/L, and the library compounds with an average concentration of 6.7 μmol/L (final volume: 100 μL). Non-specific binding was defined with 1 μmol/L SNAP7941. Data were analyzed with GraphPad PRISM (GraphPad Software, San Diego, CA, USA). The competitive inhibition constant (Ki) was calculated according to the Cheng–Prussof equation: Ki=IC50/[1+(L/Kd)], where IC50 is the concentration that produced 50% inhibition, and L is the concentration of radiolabeled ligands used[43].
[35S]GTPγS binding assay The membranes and test compounds were diluted in the binding buffer 2 (50 mmol/L HEPES, 100 mmol/L NaCl, 5 mmol/L MgCl2, 3 μmol/L GDP, 0.1% BSA, pH 7.4) in the presence of 10 mg/L saponin, in order to enhance the agonist-induced stimulation level[37,44], and then premixed with 1 g/L FlashBlue GPCR beads. For the agonist test, incubation was started by the addition of 0.2 nmol/L [35S]GTPγS to the membranes and test compounds, and carried on for 1 h at room temperature in a final volume of 100 μL. To study the antagonist activity, the membranes were pre-incubated for 2 h with MCH (200 nmol/L) in conjunction with a given concentration of a test compound. Reaction was begun with the addition of 0.2 nmol/L [35S]GTPγS followed by 1 h incubation at room temperature. Non-specific binding was assessed using non-radiolabeled GTPγS (10 μmol/L). Data were analyzed with GraphPad PRISM to calculate the 50% effective concentration (EC50), and the maximal effect (Emax) was expressed as a percentage of that observed with 1 μmol/L MCH (100%) for agonists. KB was used to describe antagonist potency: KB=IC50/(1+[agonist]/EC50), where IC50 is the antagonist concentration that gives 50% inhibition of [35S]GTPγS binding in the presence of a fixed concentration of an agonist, and EC50 is the 50% effective concentration of an agonist when tested alone[43]. The maximal inhibitory effect (Imax) was expressed as a percentage of that observed with 200 nmol/L MCH on hMCHR-1.
Results
HTS campaign In the validation process, various assay parameters were studied. Different concentrations of [3H]SNAP7941 were tested, and it was found that low concentrations of this radioligand resulted in poor signal detection. When the concentration was increased to 2.5 nmol/L, a satisfactory signal (~100-fold over the instrument noise) and signal/background ratio were achieved. Under this experimental condition, the optimal concentrations of receptor protein extract and microbeads were determined to be 1.3 mg/L and 0.75 g/L, respectively (Figure 1). The control compound, SNAP7941, was then tested on hMCHR-1 to validate the assay system. As shown in Figure 2, the Ki value of SNAP7941 was calculated to be 0.16 nmol/L, similar to that reported in the literature[35,45]. In order to apply the assay to a HTS format, the Z’ value and signal/background (S/B) ratio (i.e. specific vs non-specific binding) were studied. As shown in Figure 3, the Z’ value of this SPA method was 0.77, with an S/B ratio of 6.59 and a coefficient of variation (CV) value of 4.7%. These characteristics suggest that the assay system is of high quality and suitable for HTS[46]. The scatter plots of the HTS campaign directed towards hMCHR-1 are shown in Figure 4. Of the 48 240 samples screened, 24 initial hits were identified (≥50% inhibition of SNAP7941 binding to hMCHR-1). Among them, 12 hits were subsequently confirmed and their IC50 values determined.
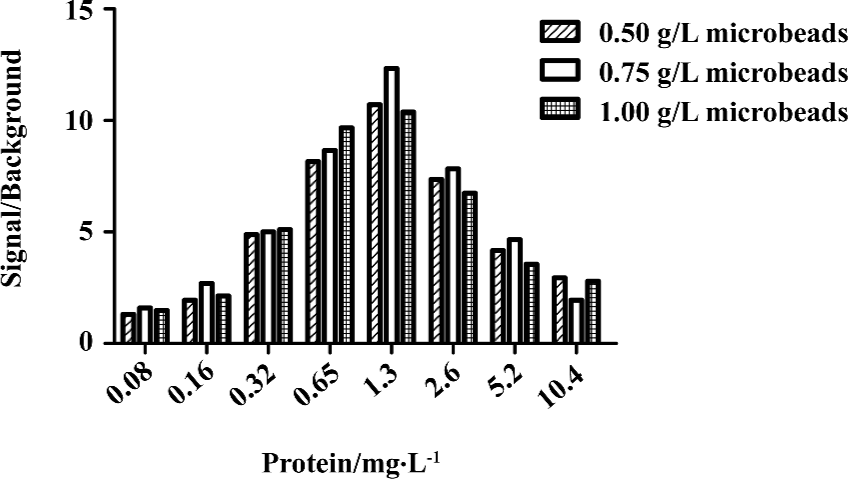
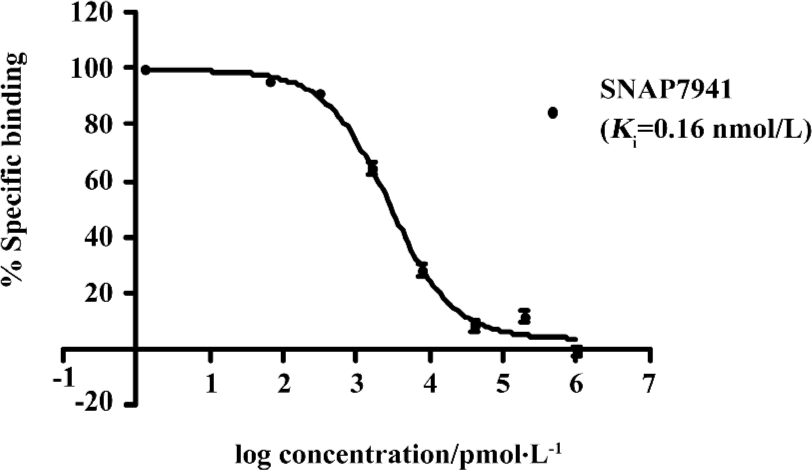
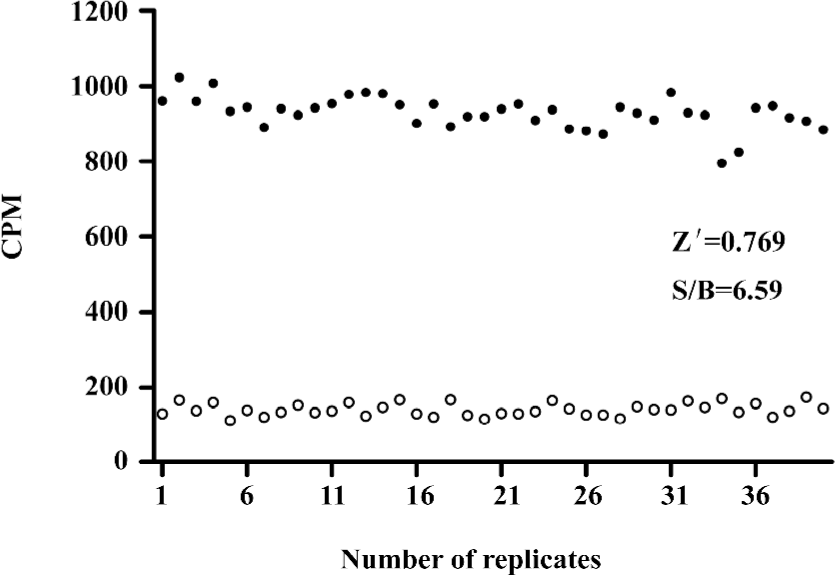
Functionality characterization To examine the pharmacological properties (agonist or antagonist activities) of these hit compounds on hMCHR-1, [35S]GTPγS binding assays were performed. The antagonist activity of SNAP7941 is shown in Figure 5. Based on the potency displayed in the receptor-binding assay, we selected 8 confirmed hits identified from the HTS campaign for simultaneous functionality studies. Two compounds exhibited an antagonist profile and none demonstrated agonist activity (Table 1). Four of the 8 compounds (NC127816, NC127844, NC127847, and NC127853) share a general chemical skeleton of 1-ethoxy-2H-2-aza-1-phospha-naphthalene 1-oxide (Figure 6), including 1 hMCHR-1 antagonist (NC127816; Figure 5), as determined by the GTPγS assay. Compound NC127398 with antagonist activity and a different chemical scaffold had a lower hMCHR-1 binding affinity and was less potent compared to NC127816 (Table 1). The other 3 confirmed hits, namely, NC091703, NC093198, and NC127858, with different scaffolds, bound to the receptor at submicromolar range, without apparent functional properties (Table 1).
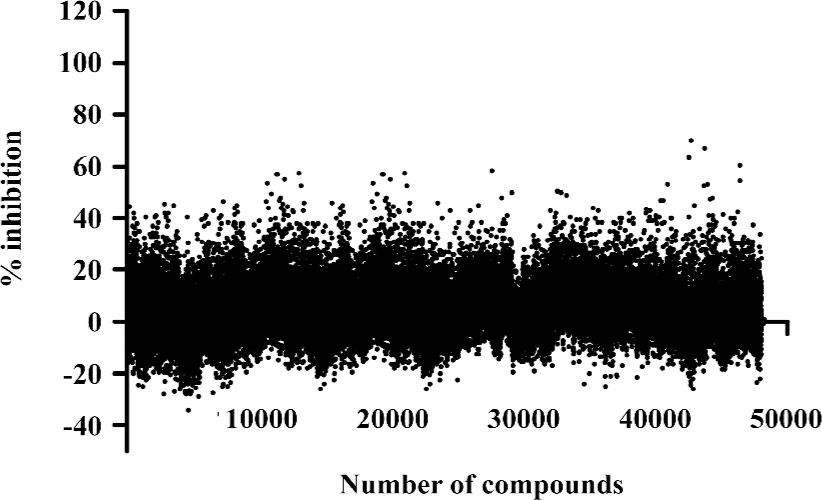
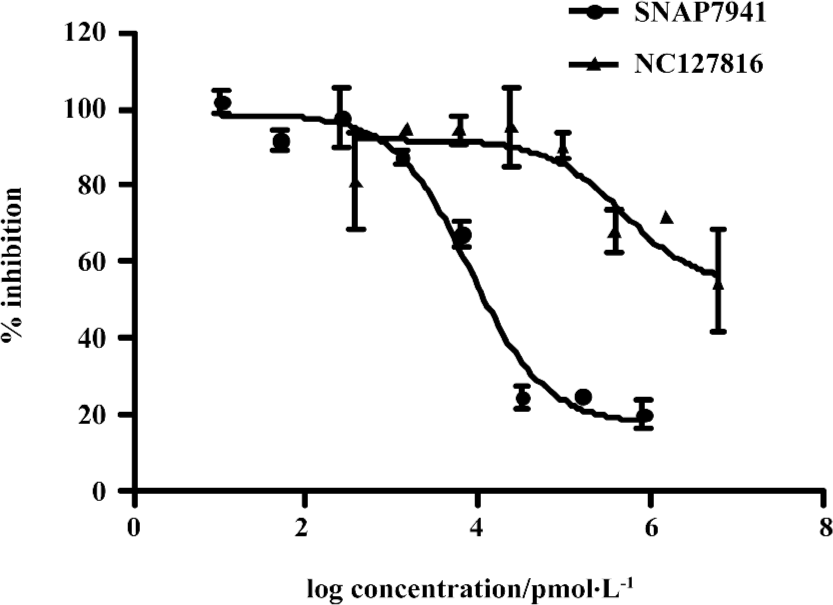
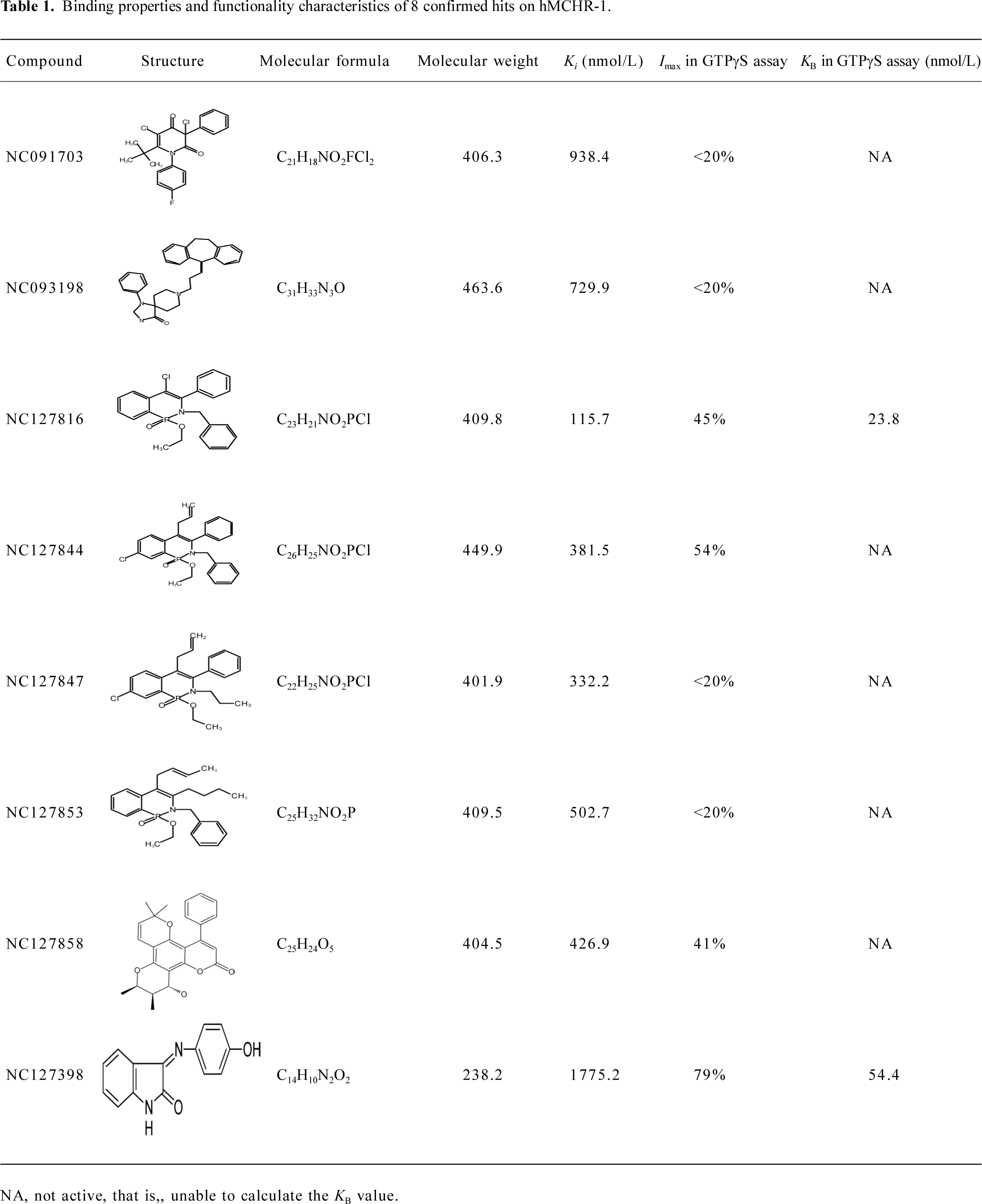
Full table
Discussion
The increasing demand for better therapeutic agents to control the worldwide obesity epidemic has led to some major efforts in this highlighted research area. As reported previously, transgenic mice overexpressing the MCH gene are susceptible to insulin resistance and obesity, while mice lacking the gene encoding MCH or MCHR-1 are lean, resistant to diet-induced obesity, and maintain elevated metabolic rates[47]. There exists compelling evidence indicating that the antagonism of the MCHR-1 system could be an effective means of treating obesity. This understanding has generated much interest among pharmaceutical/biotechnology companies in the discovery of MCHR-1 antagonists. As a result, many MCHR-1 antagonists with diverse structures have been identified, including aminoquinoline, indazole, biphenyl carboxamide, and thienopyrimidinone scaffolds. However, significant development hurdles concerning safety, selectivity, and metabolic stability remain to be overcome before they can be declared as drug candidates[48]. In addition, the broad distribution of MCH and MCHR-1 within the CNS bring about a number of diverse functions, which may cause adverse effects in chronic treatment of obesity. Thus novel scaffolds are needed to achieve the ultimate goal of discovering safer and more selective MCHR-1 antagonists.
In the present study, we describe an HTS campaign and subsequent identification of a novel antagonist to MCHR-1 and a series of new ligands with chemical skeletons not reported previously. The primary screening assay, that is, the SPA technology-based competitive receptor binding assay, was fully validated to possess an optimal Z’ factor, S/B (or signal to noise) ratio, and CV value for HTS. Of the 48 240 synthetic and natural compounds screened, 24 hits that showed ≥50% inhibition in competition with the control compound, SNAP7941, were initially identified (initial hit rate =0.05%). Among them, 12 hits (final hit rate =0.025%) were subsequently confirmed. [35S]GTPγS binding methods in both agonist and antagonist modes were used as secondary functionality screening. Among the 8 confirmed hits selected, only 1 compound (NC127816) displayed moderate hMCHR-1 binding affinity (Ki=115.7 nmol/L) and relatively potent antagonism (KB=23.8 nmol/L). Compared to the long chain structures of MCHR-1 modulators documented in the literature, NC127816 and its 3 analogs have an obviously simpler structure (Figure 6) and smaller molecular size. Based on this skeleton, we made some slight modifications according to electronic properties, steric effects, and bioisosteric replacement principles. To our surprise, most of the newly synthesized analogs bound to MCHR-1 with different affinities (data not shown), indicating the potential of using 1-ethoxy-2H-2-aza-1-phospha-naphthalene 1-oxide as a scaffold for further SAR analyses.
It is known that three dimensional (3-D) shape and electrostatic similarity play important roles in defining MCHR-1 antagonists[49]. Considering the marked difference in molecular shape and electrostatic status between NC127816 and the structures reported elsewhere, we anticipate that the derivatives of 1-ethoxy-2H-2-aza-1-phospha-naphthalene 1-oxide may represent a new class of potent MCHR-1 modulators, which could lead to not only new therapeutics for obesity, but also further comprehension of MCH/MCHR-1-related biology.
Author contribution
Ming-wei WANG designed the research; Jian-hua YAN, Qun-yi LI performed the research;Jean A BOUTIN, M Pierre RENARD, Yi-xiang DING, Xiao-jiang HAO, Wei-min ZHAO contributed new reagents or analytic tools; Ming-wei WANG and Jian-hua YAN analyzed data; Ming-wei WANG, Jian-hua YAN wrote the paper.
Acknowledgements
We are indebted to Mr Xin HUI, Ms Hong YU, Dr Ming-kai XU, and Mr Qiang SHEN for their technical assistance, and to Dr Dale E Mais for valuable discussion.
References
- Kawauchi H, Kawazoe I, Tsubokawa M, Kishida M, Baker BI. Characterization of melanin-concentrating hormone in chum salmon pituitaries. Nature 1983;305:321-3.
- Presse F, Nahon JL, Fischer WH, Vale W. Structure of the human melanin concentrating hormone mRNA. Mol Endocrinol 1990;4:632-7.
- Bittencourt JC, Presse F, Arias C, Peto C, Vaughan J, Nahon JL, et al. The melanin-concentrating hormone system of the rat brain: an immuno- and hybridization histochemical characterization. J Comp Neurol 1992;319:218-45.
- Ludwig DS, Tritos NA, Mastaitis JW, Kulkarni R, Kokkotou E, Elmquist J, et al. Melanin-concentrating hormone overexpression in transgenic mice leads to obesity and insulin resistance. J Clin Invest 2001;107:379-86.
- Qu D, Ludwig DS, Gammeltoft S, Piper M, Pelleymounter MA, Cullen MJ, et al. A role for melanin-concentrating hormone in the central regulation of feeding behaviour. Nature 1996;380:243-7.
- Shimada M, Tritos NA, Lowell BB, Flier JS, Maratos-Flier E. Mice lacking melanin-concentrating hormone are hypophagic and lean. Nature 1998;396:670-4.
- Gonzalez MI, Vaziri S, Wilson CA. Behavioral effects of alpha-MSH and MCH after central administration in the female rat. Peptides 1996;17:171-7.
- Jezova D, Bartanusz V, Westergren I, Johansson BB, Rivier J, Vale W, et al. Rat melanin-concentrating hormone stimulates adrenocorticotropin secretion: evidence for a site of action in brain regions protected by the blood–brain barrier. Endocrinology 1992;130:1024-9.
- Chiocchio SR, Gallardo MG, Louzan P, Gutnisky V, Tramezzani JH. Melanin-concentrating hormone stimulates the release of luteinizing hormone-releasing hormone and gonadotropins in the female rat acting at both median eminence and pituitary levels. Biol Reprod 2001;64:1466-72.
- Gallardo MG, Chiocchio SR, Tramezzani JH. Changes of melanin-concentrating hormone related to LHRH release in the median eminence of rats. Brain Res 2004;1030:152-8.
- Kennedy AR, Todd JF, Stanley SA, Abbott CR, Small CJ, Ghatei MA, et al. Melanin-concentrating hormone (MCH) suppresses thyroid stimulating hormone (TSH) release, in vivo and in vitro, via the hypothalamus and the pituitary. Endocrinology 2001;142:3265-8.
- Monzon ME, De Barioglio SR. Response to novelty after i.c.v. injection of melanin-concentrating hormone (MCH) in rats. Physiol Behav 1999;67:813-7.
- Knigge KM, Wagner JE. Melanin-concentrating hormone (MCH) involvement in pentylenetetrazole (PTZ)-induced seizure in rat and guinea pig. Peptides 1997;18:1095-7.
- Monzon ME, de Souza MM, Izquierdo LA, Izquierdo I, Barros DM, de Barioglio SR. Melanin-concentrating hormone (MCH) modifies memory retention in rats. Peptides 1999;20:1517-9.
- Varas MM, Perez MF, Ramirez OA, de Barioglio SR. Increased susceptibility to LTP generation and changes in NMDA-NR1 and -NR2B subunits mRNA expression in rat hippocampus after MCH administration. Peptides 2003;24:1403-11.
- Sanchez M, Baker BI, Celis M. Melanin-concentrating hormone (MCH) antagonizes the effects of alpha-MSH and neuropeptide E-I on grooming and locomotor activities in the rat. Peptides 1997;18:393-6.
- Verret L, Goutagny R, Fort P, Cagnon L, Salvert D, Leger L, et al. A role of melanin-concentrating hormone producing neurons in the central regulation of paradoxical sleep. BMC Neurosci 2003;4:19.
- Bachner D, Kreienkamp H, Weise C, Buck F, Richter D. Identification of melanin concentrating hormone (MCH) as the natural ligand for the orphan somatostatin-like receptor 1 (SLC-1). FEBS Lett 1999;457:522-4.
- Chambers J, Ames RS, Bergsma D, Muir A, Fitzgerald LR, Hervieu G, et al. Melanin-concentrating hormone is the cognate ligand for the orphan G-protein-coupled receptor SLC-1. Nature 1999;400:261-5.
- Lembo PM, Grazzini E, Cao J, Hubatsch DA, Pelletier M, Hoffert C, et al. The receptor for the orexigenic peptide melanin-concentrating hormone is a G-protein-coupled receptor. Nat Cell Biol 1999;1:267-71.
- Saito Y, Nothacker HP, Wang Z, Lin SH, Leslie F, Civelli O. Molecular characterization of the melanin-concentrating-hormone receptor. Nature 1999;400:265-9.
- Shimomura Y, Mori M, Sugo T, Ishibashi Y, Abe M, Kurokawa T, et al. Isolation and identification of melanin-concentrating hormone as the endogenous ligand of the SLC-1 receptor. Biochem Biophys Res Commun 1999;261:622-6.
- An S, Cutler G, Zhao JJ, Huang SG, Tian H, Li W, et al. Identification and characterization of a melanin-concentrating hormone receptor. Proc Natl Acad Sci USA 2001;98:7576-81.
- Hill J, Duckworth M, Murdock P, Rennie G, Sabido-David C, Ames RS, . Molecular cloning and functional characterization of MCH2, a novel human MCH receptor. J Biol Chem 2001; 276: 20 125–9.
- Mori M, Harada M, Terao Y, Sugo T, Watanabe T, Shimomura Y, et al. Cloning of a novel G protein-coupled receptor, SLT, a subtype of the melanin-concentrating hormone receptor. Biochem Biophys Res Commun 2001;283:1013-8.
- Sailer AW, Sano H, Zeng Z, McDonald TP, Pan J, Pong SS, et al. Identification and characterization of a second melanin-concentrating hormone receptor, MCH-2R. Proc Natl Acad Sci USA 2001;98:7564-9.
- Rodriguez M, Beauverger P, Naime I, Rique H, Ouvry C, Souchaud S, et al. Cloning and molecular characterization of the novel human melanin-concentrating hormone receptor MCH2. Mol Pharmacol 2001;60:632-9.
- Tan CP, Sano H, Iwaasa H, Pan J, Sailer AW, Hreniuk DL, et al. Melanin-concentrating hormone receptor subtypes 1 and 2: species-specific gene expression. Genomics 2002;79:785-92.
- Saito Y, Maruyama K. Identification of melanin-concentrating hormone receptor and its impact on drug discovery. J Exp Zoolog A Comp Exp Biol 2006;305:761-8.
- Hawes BE, Kil E, Green B, O’Neill K, Fried S, Graziano MP. The melanin-concentrating hormone receptor couples to multiple G proteins to activate diverse intracellular signaling pathways. Endocrinology 2000;141:4524-32.
- Hervieu GJ, Cluderay JE, Harrison D, Meakin J, Maycox P, Nasir S, et al. The distribution of the mRNA and protein products of the melanin-concentrating hormone (MCH) receptor gene, slc-1, in the central nervous system of the rat. Eur J Neurosci 2000;12:1194-216.
- Saito Y, Cheng M, Leslie FM, Civelli O. Expression of the melanin-concentrating hormone (MCH) receptor mRNA in the rat brain. J Comp Neurol 2001;435:26-40.
- Marsh DJ, Weingarth DT, Novi DE, Chen HY, Trumbauer ME, Chen AS, et al. Melanin-concentrating hormone 1 receptor-deficient mice are lean, hyperactive, and hyperphagic and have altered metabolism. Proc Natl Acad Sci USA 2002;99:3240-5.
- Chen Y, Hu C, Hsu CK, Zhang Q, Bi C, Asnicar M, et al. Targeted disruption of the melanin-concentrating hormone receptor-1 results in hyperphagia and resistance to diet-induced obesity. Endocrinology 2002;143:2469-77.
- Borowsky B, Durkin MM, Ogozalek K, Marzabadi MR, DeLeon J, Lagu B, et al. Antidepressant, anxiolytic and anorectic effects of a melanin-concentrating hormone-1 receptor antagonist. Nat Med 2002;8:825-30.
- Chaki S, Funakoshi T, Hirota-Okuno S, Nishiguchi M, Shimazaki T, Iijima M, et al. Anxiolytic- and antidepressant-like profile of ATC0065 and ATC0175: nonpeptidic and orally active melanin-concentrating hormone receptor 1 antagonists. J Pharmacol Exp Ther 2005;313:831-9.
- Audinot V, Beauverger P, Lahaye C, Suply T, Rodriguez M, Ouvry C, et al. Structure-activity relationship studies of melanin-concentrating hormone (MCH)-related peptide ligands at SLC-1, the human MCH receptor. J Biol Chem 2001;276:13554-62.
- Suply T, Della Zuana O, Audinot V, Rodriguez M, Beauverger P, Duhault J, et al. SLC-1 receptor mediates effect of melanin-concentrating hormone on feeding behavior in rat: a structure-activity study. J Pharmacol Exp Ther 2001;299:137-46.
- Takekawa S, Asami A, Ishihara Y, Terauchi J, Kato K, Shimomura Y, et al. T-226296: a novel, orally active and selective melanin-concentrating hormone receptor antagonist. Eur J Pharmacol 2002;438:129-35.
- Hui X, Gao J, Xie X, Suto N, Ogiku T, Wang MW. A robust homogeneous binding assay for α4β2 nicotinic acetylcholine receptor. Acta Pharmacol Sin 2005;26:1175-80.
- Harrison C, Traynor JR. The [35S]GTPγS binding assay: approaches and applications in pharmacology. Life Sci 2003;74:489-508.
- Bradford MM. A rapid and sensitive method for the quantitation of microgram quantities of protein utilizing the principle of protein-dye binding. Anal Biochem 1976;72:248-54.
- Cheng YC, Prussoff WH. Relationship between the inhibition constant (Ki) and the concentration of inhibitor which causes 50 per cent inhibition (IC50) of an enzymatic reaction. Biochem Pharmacol 1973;22:3099-108.
- Cohen FR, Lazareno S, Birdsall NJ. The effects of saponin on the binding and functional properties of the human adenosine A1 receptor. Br J Pharmacol 1996;117:1521-9.
- Chen CA, Jiang Y, Lu K, Daniewska I, Mazza CG, Negron L, et al. Synthesis and SAR investigations for novel melanin-concentrating hormone 1 receptor (MCH1) antagonists part 2: A hybrid strategy combining key fragments of HTS hits. J Med Chem 2007;50:3883-90.
- Zhang JH, Chung TD, Oldenburg KR. A simple statistical parameter for use in evaluation and validation of high throughput screening assays. J Biomol Screen 1999;4:67-73.
- Kowalski TJ, McBriar MD. Therapeutic potential of melanin-concentrating hormone-1 receptor antagonists for the treatment of obesity. Expert Opin Investig Drugs 2004;13:1113-22.
- Luthin DR. Anti-obesity effects of small molecule melanin-concentrating hormone receptor 1 (MCHR1) antagonists. Life Sci 2007;81:423-40.
- Muchmore SW, Souers AJ, Akritopoulou-Zanze I. The use of three-dimensional shape and electrostatic similarity searching in the identification of a melanin-concentrating hormone receptor 1 antagonist. Chem Biol Drug Des 2006;67:174-6.