Tea beverage in chemoprevention and chemotherapy of prostate cancer1
Introduction
Prostate cancer (PCa), one of the most invasive and most frequently diagnosed male malignancies in the United States, is the second leading cause of cancer-related deaths in American males with similar trends in other western countries[1]. In the year 2002, 679 023 new cases were reported worldwide and the disease killed 221 002 (three-quarters of all cases are in men aged 65 or more) making this the fifth most common cancer in the world and the second most common in men (11.7% of new cancer cases overall; 19% in developed countries and 5.3% in developing countries)[2]. According to a projection by the American Cancer Society, approximately 218 890 men will be diagnosed with PCa in the USA in the year 2007, and 27 050 PCa-related deaths are predicted[1]. The incidence of PCa increases rapidly with advancing age, and multiple genetic and epigenetic factors have been implicated in the development of PCa. Despite the substantial morbidity and mortality, the etiology of PCa is poorly understood. The limitations in the clinical management of PCa are derived not only from the fact that no single gene or molecule has served as a reliable marker of PCa but also from the reality that, as yet, an effective therapeutic regimen for its treatment is lacking. It is increasingly appreciated that environment and lifestyle, particularly dietary habits, also contribute substantially to the disease outcome[3]. It is of interest that in western countries PCa is more prevalent than their eastern counterparts, possibly due to the varied divergence in their dietary habits (Figure 1).
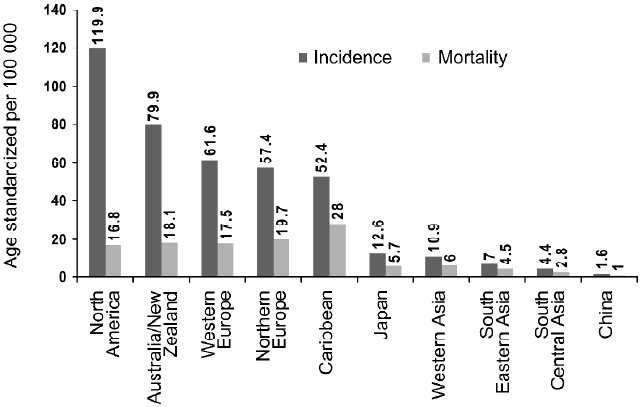
Evidence from geographic, epidemiological and in-vitro and in-vivo experiments suggest that environmental carcinogenic factors and nutrition play important causative roles in the initiation, promotion and progression of PCa[4–7]. Although clinical PCa incidence and mortality vary greatly among populations[3,8], the frequency of latent PCa is evenly distributed. The rising incidence of PCa in several countries that previously were considered to have low incidence rates appear to be coincident with the adoption of western lifestyle in those populations, implicating factors such as low levels of physical activity, high relative body weight and high dietary fat intake in the pathophysiology of PCa[9]. While environmental carcinogenic factors are difficult to control, dietary habits can easily be modified based only on individual decisions.
One case-control study has shown a positive association of PCa risk with total energy intake, as well as total fat intake[10]. These observations, combined with an improved understanding of the molecular biology of the disease provide leads to explore testable PCa prevention strategies. Current treatment strategies such as hormone therapy, radiation and surgery are proving useful in reducing the mortality associated with PCa, however, malignant and non-malignant tumors continue to progress and become refractory to these treatment options. Although, these treatments provide a limited success in reducing the mortality in PCa patients, the severe side effects of these treatments have also been reported. These include rectal complications, urinary incontinence, and impotence, loss of libido, weight gain, gynaecomastia, liver inflammation, and osteoporosis[11]. Because PCa is a complex process that involves different molecular events, usually occurring simultaneously, blocking or inhibiting only one event will not be sufficient to prevent or delay the onset of the disease. It is therefore necessary to intensify our efforts for a better understanding of PCa pathophysiology, and for the development of novel approaches for its prevention and treatment. Among many preventive approaches chemoprevention through the use of non toxic dietary substances is gaining popularity[12,13].
Many types of natural agents that act on various molecular targets have been reported to inhibit or delay various stage(s) of cancer[12,13]. Epidemiological studies have shown a correlation between populations with higher consumption of selenium and vitamin E, fruits and tomatoes, in lowering the risk of PCa[14,15]. Consistent with this notion, several single natural agents are currently being studied for their potential as preventive agents against PCa. Tea derived from the plant Camellia sinensis, has been studied extensively and shown to have anti-mutagenic and anti-cancer effects in animal tumor models[16,17]. In 1999, we initiated a program to assess whether tea consumption could afford chemopreventive effects against prostate carcinogenesis[18], which we continue to pursue. This research has also been extensively followed in many laboratories around the world. In this review we summarize the laboratory studies, clinical trials and epidemiological observations supporting the use of tea or its individual constituent polyphenols in prevention and/or better management of PCa.
Chemoprevention and prostate cancer
The magnitude of the problem of cancer and the failure of conventional strategies to effect a marked diminution in the total number of deaths from this disease now indicate that other preventive or therapeutic measures should be seriously explored. Many strategies are possible to reduce cancer-related deaths, four of which are noteworthy: (1) prevention; (2) early diagnosis and intervention; (3) successful treatment of localized cancer; and (4) improved management of non-localized cancer. Among these, prevention appears to be the most practical approach. One approach for preventing the occurrence of cancer(s) is through chemoprevention. Chemoprevention, by definition, is a means of cancer control in which the occurrence of the disease can be entirely prevented, slowed or reversed by the administration of one or more naturally occurring and/or synthetic compounds[19–23]. This approach is promising because therapy and surgery have not been fully effective against the high incidence and/or low survival rate of most cancer types. Furthermore, this approach with the use of dietary substances appears to have practical implications in reducing cancer risk because unlike the carcinogenic environmental factors that are difficult to control, individuals can make decisions to modify the food and beverages they consume.
Chemoprevention, especially through consumption of tea, appears to be a useful strategy for the management of PCa. This is well-supported by the epidemiological observation that the Japanese and Chinese populations, which are habitual drinkers of several cups of tea, have one of the lowest rates of PCa in the world[24]. PCa is believed to be an ideal candidate disease for chemoprevention because of its high latency period and because it is typically diagnosed in men over the age of 50. Thus, even a slight delay in the progression of this disease by chemoprevention could result in substantial reduction in the incidence of the disease and more importantly, improve the quality of life of the patients with the disease[25,26]. If chemoprevention delays the clinical course of PCa even by 5 years, the incidence of and deaths from this disease would decrease substantially[27]. The identification of promising agents (and their molecular targets) for PCa prevention is guided by data derived from a variety of sources. These evidence-based leads come from (1) epidemiological observations; (2) PCa clinical trials; (3) secondary analyses from large, randomized, controlled cancer clinical trials; (4) understanding the mechanism of prostate carcinogenesis; and (5) experimental animal models.
Tea: an overview
Tea, made from the leaves of Camellia sinensis, an evergreen shrub of the Theaceae family, is a beverage of choice in many countries around the world. The first instance of tea drinking dates back to 2737 BC. According to a Chinese legend, it was Emperor Shen Nung of China who accidently discovered the refreshing effects and fine taste of tea when he was out camping and a few leaves of a tea plant fell into his cup of boiling water. Currently tea plant is cultivated in approximately 30 countries. Based on the method of processing, tea is typically of three types: black, green and oolong. Black tea, predominantly consumed in Western and some Asian countries, shares a major part of world tea production, accounting for about 78% of total tea consump-tion. About 20% of the total tea manufactured is green tea, and is mainly consumed in China, Japan, India, and a few countries in North Africa and the Middle East. The remaining 2% of tea consumed is oolong tea, mainly produced and consumed in southeastern China.
Tea contains several polyphenolic components, which are antioxidative in nature, and many studies have shown that tea polyphenols possess the ability to prevent oxidant-induced cellular damage[28,29]. In recent years, studies from ours and many laboratories around the world, conducted in various organ-specific animal bioassay systems, have shown that tea and its polyphenolic constituents are capable of affording protection against a variety of cancer types[13,16,19,29]. Although most of the studies conducted have used green tea, a limited number of studies have also shown the anti-cancer efficacy of black tea.
Tea as a beverage is consumed worldwide at greatly varying levels. Not only does tea consumption vary from country to country, but also there is enormous variation within a given population. This ranges from no tea to as many as 20 or more cups per day. Although definite data are not available, it is generally accepted that next to water, tea is the most consumed beverage in the world; with a per capita worldwide consumption of approximately 120 mL per day[30].
The composition of tea-leaf varies with climate, season, horticultural practices, variety of the plant, and the position of the leaf on the harvested shoot (ie, its age). The composition of different types of teas varies according to the manufacturing process, which differs in the degree of ‘enzymatic oxidation’ or fermentation. Thus, green tea is unfermented, oolong tea is partially fermented, and black tea is fully fermented. Table 1 shows the principal polyphenolic components present in a typical green and black tea beverage, but variations may be considerable. Oolong tea composition in general falls between that of green and black teas[31].
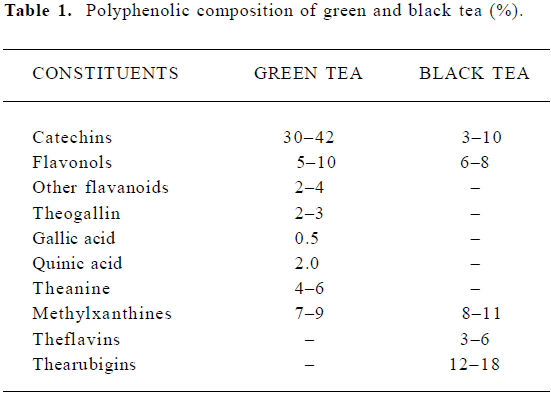
Full table
Polyphenols of tea
Tea leaves are quite unique in that they are a rich source of catechins, theanine and methylxanthines (Table 1). The chemical composition of green tea is more or less similar to that of the fresh leaf with regard to the major components. Green tea contains polyphenolic compounds, which include flavanols, flavandiols, flavonoids, and phenolic acids. These compounds account for up to 30% of the dry weight of green tea leaves. Most of the polyphenols present in green tea are flavanols, commonly known as catechins. Some major catechins present in green tea are (-)-epicatechin (EC), (-)-epicatechin-3-gallate (ECG), (-)-epigallocatechin (EGC), and (-)-epigallocatechin-3-gallate (EGCG). The chemical structures of these compounds are given in Figure 2. In addition, caffeine, theobromine, theophylline, and phenolic acids such as gallic acids are also present in green tea (Table 1). Green teas are generally produced in two different varieties; white tea and yellow tea, the latter is less fermented because of a process known as wilting. In the production of black and oolong tea, fresh leaves are allowed to wither until the moisture content of the leaves is reduced to about 55% of the original leaf-weight resulting in the concentration of polyphenols and the deterioration of leaf-structural integrity. The withered leaves are rolled and crushed, initiating fermentation of the polyphenols. This process is known as maceration. During these processes the catechins are converted to theaflavins (TF) and thearubigins. TF are astringent compounds contributing importantly to color and taste of black tea. The thearubigen fraction is a mixture of substances, with a molecular weight distribution of 1 000–40 000 and account for 15% of dry weight solids of black tea. Oolong teas are prepared by firing the leaves shortly after rolling to terminate the oxidation and drying the leaves. Normal oolong tea is considered to be about half fermented compared to black tea. Oolong tea extracts contain catechins at a level of 8%–20% of the total dry matter.
During the fermentation process involved in the manufacture of black tea, the monomeric flavanols undergo polyphenol oxidase-dependent oxidative polymerization leading to the formation of bisflavanols, TF, thearubigins, and some other oligomers. TF (1%–2%, on dry weight basis) contains benzotropolone rings with dihydroxy or trihydroxy substitution systems. About 10%–20% of the dry weight of black tea is due to thearubigens, which are even more extensively oxidized and polymerized. The structures of TF and thearubigins are shown in Figure 2. Oolong tea contains monomeric catechins, TF, and thearubigins. In addition, epigallocatechin esters, theasinensins, dimeric catechins, and dimeric proanthocyanidins are also the characteristic components of oolong tea.
Biochemical properties of tea and its poly-phenols
Tea is consumed worldwide for a variety of reasons from improving blood flow, eliminating toxins and improving resistance to various diseases and combating two of the greatest killers of human population: cancer and cardiovascular diseases. Supportive scientific evidence for these claims, based on the data generated in recent times have lead to an increase in consumption of green tea. The most widely recognized biological properties of tea polyphenols are the antioxidant properties[30,32]. Studies have shown that tea or EGCG can lower lipid absorption and plasma lipid levels in rats[33], increase high-density lipoprotein cholesterol in humans[34] and decrease blood glucose[35,36]. The health beneficial effects of tea and its polyphenols have been summarized recently in a review from our laboratory[30].
Several studies have suggested that the polyphenols, present in tea possess high antioxidant activities, which in turn, protects cells against the adverse effects of damaging reactive oxygen species (ROS) such as superoxide radical, hydroxyl radical, singlet oxygen, hydrogen peroxide, peroxynitrite, and alkoxyradicals that are constantly produced in the body. These ROS damage lipids, proteins, nucleic acids, and cellular components such as ion channels, membranes, and chromatin leading to cellular injury and cellular dysfunctions and thus contribute to the etiology of many chronic health problems including inflammatory diseases, diabetes, obesity, cancer and cardiovascular diseases[29,30,37,38]. Studies in culture systems have shown that both green tea extract and ECGC are capable of inhibiting the growth of a variety of mouse and human cancer cell types[13,19,30]. Ahmad et al[39] initially reported these effects showing that green tea polyphenol (GTP) may protect against cancer by causing cell cycle arrest and inducing apoptosis in various carcinoma cells without affecting normal cells.
Some of the effects of tea polyphenols may also be due to the chelation of metal ions. Because of its chelating properties, tea may additionally protect against toxicity due to heavy metals. Tea manifests chelating activity in-vivo as indicated by the fact that tea consumption lowers absorption of dietary iron in controlled feeding studies and decre-ases body iron balance[40]. This study had also shown that EGCG may chelate cations, which contribute to its ability to inhibit angiotensin-converting enzyme. Tea polyphenols chelate copper ions and this mechanism has also been suggested to protect low-density lipoproteins from peroxi-dation[41]. Catechins may also affect signal transduction pathways, modulate many endocrine systems, and alter hormones and other physiological processes as a result of their binding these metals and enzyme co-factors[42]. Tea polyphenols have been shown to inhibit the activation of transcription factors AP-1 and NF-κB and synthesis of nitric oxide[43–46]. Inhibition of cell transformation and cell growth by purified catechins and TF has been reported [47]. These activities have been attributed to the inhibition of AP-1 activity, possibly due to the inhibition of several steps of signal transduction pathways [eg, mitogen-activated protein kinase (MAPK) activities by tea polyphenols][48]. It has been reported that tea and its polyphenolic constituents impart inhibitory effects on the activities of many enzymatic and metabolic pathways relevant to cancer development[49].
Tea and its constituents in the management of PCa
For many years the concept of management of PCa by means of dietary intervention has been the subject of increasing attention and discussion[50]. Epidemiological studies show that in Asian populations that consume tea, the incidence of all types of cancer including PCa is low compared to that in the West[3,8,24,51,52]. Thus, it has been suggested that the low occurrence of PCa in Asian countries may be due, in part, to the consumption of green tea by these populations.
In the year 1999, our laboratory initiated a program to assess the effect of tea consumption on prostate carcino-genesis. Because the role of the androgen-responsive gene, ornithine decarboxylase (ODC), the key regulatory enzyme in the biosynthesis of polyamine is well documented in carcinogenesis[53], we analyzed ODC activity in paired benign and cancer tissues obtained from same individual with PCa and found that ODC activity in cancer tissue was 2.7-fold higher than in the corresponding paired benign tissue. This exciting observation suggested that ODC could be extrapolated as a target for prevention and therapy of PCa. Extending these studies we investigated the effect of the polyphenolic fraction isolated from green tea (GTP) against testosterone-mediated induction of ODC in (1) LNCaP cells; (2) Cpb:WU rats; and (3) C57BL/6 mice; and found that GTP resulted in a significant reduction in testosterone-mediated induction of ODC activity in the prostate[18]. We then reasoned that these studies should be extrapolated and GTP should be able to inhibit PCa development in an appropriate animal model of PCa. Transgenic adenocarcinoma of the mouse prostate (TRAMP) is one such model for PCa that closely mimics progressive forms of the human disease[54]. In this model, we provided convincing evidence that oral infusion of GTPs (equivalent to 6 cups of green tea consumption in humans) inhibits prostate carcinogenesis[55,56]. The outcome of this study was later verified by Caporali and colleagues who demonstrated that GTC significantly inhibited PCa development in TRAMP mice[57]. This study further demonstrated that in TRAMP mice, the clusterin gene was dramatically downregulated during onset and progression of PCa. In the GTC-treated TRAMP mice in which tumor progression was chemoprevented, clusterin mRNA and protein progressively accumulated in the prostate gland. The clusterin levels returned to undetectable levels in animals in which GTC chemoprevention failed and PCa developed.
Below, we summarize the biological activities of tea and its constituents in relation to PCa.
Effect of tea polyphenols on androgen synthesis and androgen receptor Because androgens are capable of both stimulating proliferation as well as inhibiting the rate of glandular epithelial cell death within the prostate, androgen ablation therapy is commonly suggested for men with this non-organ confined disease[58]. Huggins et al for the first time demonstrated the use of androgen deprivation as a treatment for advanced PCa[59]. Since then removal of androgenic stimulation has been used to treat this disease. Kao et al[42] reported that treatment with EGCG (85 mg/kg body weight) for 7 d reduces the circulating testosterone levels by 70% in a murine model that can be associated with the lowering of PCa risk. Studies that followed have shown that tea polyphenols might block the pathway that leads to the synthesis of androgen. Populations with impaired androgen metabolism such as hereditary 5α-reductase deficiency do not develop PCa, while those with higher circulating levels of androgen are at a higher risk of the disease[60]. In tumor xenograft studies of PCa, the 5α-reductase inhibitors were found to slow the growth of previously established tumors[61]. It has been reported that green tea constituents, EGCG and ECG, inhibit the activity of type 1 rat 5α-reductase[62]. These polyphenols also inhibited types 1 and 2 human 5α-reductase in microsomes from rat cells that expressed the human enzyme. Several molecular mechanisms have been postulated to be accountable for the development of sporadic hormone-refractory tumors. Most of these mechanisms involve alterations in the function of the androgen receptor (AR) and its complex signaling pathways[63]. Several studies have demonstrated that AR is expressed in all of the stages of PCa, and at least one-third of advanced PCa contain amplified AR genes[64–66]. It is suggested that overexpres-sion or mutation of the AR in PCa cells may promote a growth advantage. Therefore, it has been of great interest to seek more effective means of minimizing or eliminating the function of the AR in order to achieve preventive and/or therapeutic treatments for prostatic neoplastic disease. One study reported that tea polyphenols downregulated the expression of the AR in androgen responsive to LNCaP prostate carcinoma cells[67]. They reported a significant reduction in AR mRNA by the EGCG treatment. The basal activity of the AR promoter is determined by an Sp1 binding site within the AR core promoter region[68,69]. Sp1 not only regulates the basal expression of the AR but also acts as its coregula-tor[70]. Sp1 is involved in the expression of genes related to cell proliferation[71]. Since Sp1 regulates the expression of many critical genes, the decrease in this protein by tea polyphenols could somewhat decrease the growth rates of prostatic cells. EGCG has been reported to significantly decrease the Sp1 DNA binding activity[67]. However additional work is necessary to authenticate the effect of tea constituents on androgen synthesis or its receptor status in a manner that could lead to protection against development of PCa.
Effect of tea polyphenols on prostate specific antigen Prostate specific antigen (PSA) is a glycoprotein secreted by the prostate gland. Functionally, PSA is a kallikrein-like serine protease produced by the epithelial cell lining of the acini and ducts of the prostate gland[72]. It circulates in the serum in both free (unbound) and complex forms. The most common cause for an elevated serum PSA is benign prostatic hyperplasia, the incidence of which increases with age, similar to PCa. Reduction in serum PSA levels has been proposed as an endpoint biomarker for staging hormone-insensitive human PCa intervention. At present, the measurement of serum PSA levels is the most commonly used biomarker for monitoring the progression of PCa and the response to therapy[66]. It has been suggested that serum PSA levels can be decreased by the agents that lower serum testosterone levels such as leutinizing hormone releasing hormone agonists and antagonists, anti-androgens such as flutamide and bicalutamide, and the 5α-reductase inhibitors such as finasteride[73,74]. We evaluated the effect of EGCG on the production of PSA in androgen-sensitive human prostate carcinoma LNCaP cells. This study demonstrated that EGCG treatment resulted in significant dose-dependent decrease in PSA level in the culture medium. Further, a significant time-dependent decrease in PSA production was also observed after EGCG treatment compared with the control. The validity of these cell culture observations to human PCa patients could have implications in reducing PCa body burden. Therefore, clinical data where PSA levels are being monitored after administration of green tea to PCa patients could be of interest.
Polyamine synthesis and tea polyphenols Polyamines are present in all living cells and are essential for cell differentiation, proliferation[75] and migration[76]. Depletion of polyamines results in the inhibition of cell proliferation and migration and the failure of embryonic development, whereas accumulation of polyamines causes apoptosis[77,78] or cell transformation[79,80]. Cells maintain intracellular polyamines at optimal levels by regulating synthesis or degradation and by uptake or release of polyamines from or to the exterior. Prostate tissue is known to contain some of the highest concentrations of polyamines and polyamine-metabolizing enzymes in the body[81,82]. ODC is a key regulatory enzyme for polyamine synthesis and the induction of its activity has been reported to be linked with various types of cancers including PCa[53,83–85] and thus, ODC has been used as a biomarker for the chemopreventive studies[86]. An induction in the ODC activity and ODC mRNA expression mediated by testosterone has been reported in prostate carcinoma cells[87,88]. Our laboratory reported that GTPs significantly reverse the induction of ODC activity as well ODC mRNA expression in LNCaP cells[18]. We also demonstrated that testosterone when administered to the C57 BL/6 mice, caused a 2-fold increase in ODC activity in the ventral prostrate while prior oral infusion of 0.2% (w/v) GTP in drinking water resulted in 40% inhibition in this induction. In a recent study EGCG was shown to decrease both tumor number and total tumor burden compared with untreated ODC/Ras mice without decreasing the elevated polyamine levels present in the ODC/Ras mice[89].
Tea polyphenols and gene expression Cancer is believed not to be a single disease but rather a conglomeration of several diseases and several genes are known to play imperative roles at various stages and at different levels in it. These include the genes that regulate growth, cell signaling, differentiation, cell death, cell division and cell migration. There is always an upregulation or suppression of such genes in the process of cancer development. Tea polyphenols have been shown to modulate the function of various genes at various levels. We identified nine genes, including six kinases and three phosphatases, whose expression was found to be downregulated by EGCG[90]. The genes repressed by EGCG mostly belonged to the G-protein signaling network and are responsible for cell proliferation, and include adenosine kinase, protein kinase C alpha (PKC-α) and type I β cGMP-dependent protein kinase. Interestingly, the PKC-α form, whose inhibition of expression has been shown to inhibit cell growth in some cancer cells, was selectively repressed by EGCG while the expression of six other PKC isoforms (β, δ, ε, μ, η and ζ) was unaffected[90]. PKC is known to be involved in several diverse cellular functions including cell differentiation, growth control, tumor promotion and progression and cell death. It is also a regulator of cell cycle events during G1 progression and G2/M transition. Inhibition of PKC-α gene expression is believed to inhibit cell proliferation in the animal tumor model and in some human cancer cell lines. We have shown that EGCG inhibits the expression of the PKC-α gene, adenosine kinase and type I β cGMP-dependent protein kinase in LNCaP cells and hence is able to block the intracellular cyclic-nucleotide signaling cas-cade[90].
The loss of tumor suppressor genes and the genes producing anti-growth factors is an important event in cancer development. Studies have shown that EGCG induces the expression of 16 kinases and phosphatase genes in prostate cells. These include tumor suppressor gene SHP-1 and the genes that produce pyrroline-5-carboxylate and prostatic acid phosphatase. The p53 tumor suppressor gene is the most frequently mutated gene found in human malignancies, including cancer of the prostate gland. Generally, no correlation between p53 mutation and early stage PCa has been noticed but p53 mutations are shown to be associated with 10%–20% of advanced PCa patients with high Gleason grade and distant site metastasis[91,92]. In a study from our laboratory, we have shown that EGCG upregulated the protein levels of p53 in LNCaP cells (with wild type p53) but not in DU 145 cells (with mutant p53)[93]. Further, we have also shown that EGCG-induced apoptosis in LNCaP cells occurs through the stabilization of p53 protein via phosphorylation on critical serine residues and p14ARF- mediated downregula-tion of MDM2 protein[94].
Role of tea polyphenol on programmed cell death (apoptosis) in PCa Morphologically, apoptosis is characterized by a temporal sequence of events consisting of chromatin aggregation, nuclear and cytoplasmic condensation, and eventual fragmentation of the dying cell into a cluster of membrane bound segments that often contain morphologically intact organelles[95]. These apoptotic bodies are rapidly recognized, phagocitized, and digested by either macrophages or adjacent epithelial cells[96]. The hallmark of apoptosis is the fragmentation of genomic DNA, an irreversible event that commits the cell to die and occurs before changes in plasma and internal membrane permeability[97]. In addition, apoptosis is a discrete way of cell death that is different from necrotic cell death and regarded to be an ideal method of cell elimination[98]. Under normal cellular situations a balance between cell growth and cell death is main-tained, this balance is lost in favor of cell growth in the case of cancers including cancer of the prostate gland. Correction of this imbalance could lead to the prevention and even ablation of prostatic cancer[99,100] as apoptosis is closely involved in the initiation, progression, and metastasis of human PCa. Since human PCa represents a heterogeneous mixture of androgen-dependent and androgen-independent mixture of cells, the surgery and chemotherapy have failed to address this problem. Hence, one potential strategy to eradicate this mixture of cells is to modulate the apoptotic machinery by chemopreventive agents. Chemopreventive agents, which can modulate apoptosis, may be able to affect the steady-state cell population that can be useful in the management and therapy of cancer. Many cancer chemopre-ventive agents have been shown to induce apoptosis and conversely several tumor promoters have also been shown to inhibit apoptosis[101–103]. Therefore, it is reasonable to assume that the chemopreventive agents that have proven effects in animal tumor bioassay systems and/or human epidemiology on one hand and cause induction of apoptosis of cancer cells on the other hand may have wider implications for cancer control. Studies from this and other laboratories have shown that EGCG results in an induction of apoptosis in several human carcinoma cells[39,93,104]. A study from our laboratory has demonstrated that green tea constituent EGCG results in an induction of apoptosis in human PCa cells LNCaP and DU145[93]. This observation was subsequently verified by the studies from another labratory[105]. We recently demonstrated that EGCG-induced apoptosis in human prostate carcinoma LNCaP cells is mediated via modulation of two related pathways: (1) stabilization of p53 and down regulation of MDM2 protein; and (2) negative regulation of NK-κB activity, thereby, decreasing the expression of the pro-apoptotic protein Bcl-2[94].
Role of tea polyphenols on angiogenesis in PCa Cancer cells move from their original sites and invade surrounding tissues, a phenomenon known as ‘metastasis’. Metastasis is subsequently followed by the formation of new blood vessels, a phenomenon known as ‘angiogenesis’, a major event of later stages of carcinogenesis. Some hydrolases and matrix metalloproteases (MMPs) have been reported to be overexpressed during the invasion of cancer cells and angiogenesis[106,107]. Once tumors become aggressive and start to metastasize to other organs, even systemic chemotherapy may be futile. Garbisa et al[108] reported that EGCG is a potent inhibitor of gelatinases and an orally available pharmacologic agent that may confer the antiangiogenic and antimetastatic activity associated with green tea. EGCG was also found to inhibit tumor cell invasion and directly suppress the activity of two matrix metalloproteases, MMP2 and MMP9, most frequently overexpressed in cancer and angiogenesis and essential in penetrating the basement membrane barriers[109–111]. Recently we showed that green tea infusion in the TRAMP mice resulted in marked inhibition of the markers of angiogenesis and metastasis most notably vascular endothelial growth factor (VEGF), urokinase plasminogen activator (uPA), and MMPs 2 and 9[112]. It has been shown that tea components slow the progression of LNCaP human prostate tumors in SCID mice, partly by inhibiting the formation of new blood vessels[113]. A recent study investigated the effect of EGCG on the tube formation of human umbilical vein endothelial cells (HUVEC) on matrigel. Tube formation was inhibited by treatment both prior to plating and after plating endothelial cells on matrigel with EGCG. EGCG treatment also was found to reduce the migration of endothelial cells in a matrigel plug model. These findings suggest that EGCG also acts as an angiogenesis inhibitor by modulating protease activity during endothelial morphogenesis[110].
Role of tea polyphenols on Insulin like growth factor in PCa Studies established that elevated circulating levels of Insulin-like growth factor 1 (IGF-1) are associated with increased risk of several common cancers, including those of the breast, prostate, lung and colorectum[114]. IGF-1 has mitogenic and anti-apoptotic effects on prostate epithelial cells in vitro and has been strongly implicated in the etiology of human PCa. The level of IGF-binding protein 3 (IGFBP-3), a major IGF-1 binding protein in serum that, in most situations, suppresses the mitogenic action of IGF-1, has been shown to be inversely associated with the risk of different cancers[115]. The elevated level of IGF-1 with concomitant lowering of IGFBP-3 in serum is an excellent predictor of PCa progression in humans. The identification of agents that can inhibit the IGF-1 signaling pathway could lead to the development of highly successful prevention strategies for PCa. IGF-1 has been implicated as an important factor in the initiation and progression of PCa in a TRAMP model[116], and we have shown that oral infusion of GTP significantly lowers IGF-1 levels and restores the depleted levels of IGFBP-3 in the serum of TRAMP mice[55]. In a study, we demonstrated that the IGF-1/IGFBP-3 signaling pathway is a prime pathway for GTP-mediated inhibition of PCa that limits the progression of cancer through inhibition of angiogenesis and metastasis[112].
Regulation of cell cycle in prostate cancer cells by tea polyphenols The mammalian cell division cycle is a set of events that governs the self-replication of cells. Normal cell growth and division depends on precise regulation of cell cycle; the loss of which can result in unrestrained cell division. The consequence of this includes cell proliferation, tumorigenesis and cancer. A controlled cell cycle progression is important and essential for normal tissue homeostasis[98]. Cell cycle progression is monitored by surveillance mechanisms, or cell cycle checkpoints, that ensure initiation of a later event is coupled to the completion of an early cell cycle event. One or more cell-cycle checkpoint defects are involved in most of the cancer types including PCa[93,117,118]. A normal cell cycle progression is dependent on the ability of the cell to translate extracellular signals, such as mitogenic stimuli and intact extracellular matrices in order to efficiently replicate DNA and divide. The cell cycle progression is controlled by kinase complexes consisting of a cyclin-dependent kinase (Cdk) subunit and a cyclin subunit. Cdk respond to the extracellular signals and push cells through the cell cycle. Transitions between different phases in the cell cycle are driven by sequential activation and inactivation of various Cdk in complexes with cyclins. Abnormal Cdk activity is accomplished by cyclin amplification, cdk or substrate mutation as well as inactivation of inhibitors such as WAF1/p21, INK4a/p16, and INK4c/p18[120]. The selective growth advantage of cancer cells also stems from the amplification of positive growth signals and mutation of checkpoints. The loss of cell cycle checkpoints results in the selection of cells that have a growth advantage that may result in drug resistance, invasion and metastasis[121]. Also, inhibition of the cell cycle has been appreciated as a target for the management of cancer[122]. A few reviews are available that contain information about the dysregulation of cell cycle in prostate carcinogenesis[118,119]. Tea polyphenols have been shown to impart cell cycle arrest in cancer cells via inducing the expression of cdk inhibitors[123]. Earlier, our laboratory showed that EGCG exhibited a dose-dependent arrest of cells in G0/G1 phase of the cell cycle, thereby slowing the growth of human PCa cells[93]. Recently, we elucidated the molecular mechanism involved in the cell cycle arrest in human PCa cells[123]. Our data demonstrated that EGCG treatment of LNCaP and DU-145 cells resulted in significant dose- and time-dependent (1) upregulation of the protein levels of WAF1/p21, INK4a/p16, and INK4c/p18; (2) down-modulation of the protein levels of cyclin D1, cyclin E, cdk2, cdk4, and cdk6, but not of cyclin D2; and (3) increase in the binding of cyclin E toward cdk2. This resulted in a blockade of G1 to S transition, causing a G0/G1 phase arrest of the cell cycle. The effect of EGCG on the interbinding between the different components of the cki-cyclin-cdk network, however, needs further investigation.
Modulation of phosphatidylinositol-3-kinase/protein kinase B and mitogen activated protein kinases by tea Phosphatidylinositol-3-kinase (PI3K)/protein kinase B (PKB) and MAPK signaling pathways are regarded to play a critical role in cellular proliferation, cell cycle regulation and apoptosis. Defects in these signaling pathways have been found to result in the development of various cancer types[124]. PI3K catalyzes the formation of the 3’-phosphoinositides, phosphatidylinositol 3,4-diphosphate and phosphatidylinositol 3,4,5-triphosphate. An increase in 3’-phosphoino-sitides leads to membrane translocation of downstream effectors such as the serine/threonine protein kinase Akt, resulting in increased cellular proliferation and protection from apoptosis[125]. The MAPK family such as extracellular signal regulated protein kinase (Erk) 1 and 2 are shown to be constitutively active in PCa in humans, and possibly play a causative role in the progression of this malignancy from an androgen-sensitive phenotype to an advanced and androgen-insensitive metastatic disease. It has been shown that the activation of MAPK by green tea might be a potential mechanism in the regulation of antioxidant-responsive element mediated phase II enzyme gene expression[126]. In one report, the authors found that GTP causes a significant activation of extracellular signal-regulated kinase 2 (ERK2) and c-Jun N-terminal kinase 1 (JNK1)[126]. GTP treatment also resulted in increased mRNA levels of the immediate-early genes c-jun and c-fos, as determined by reverse transcriptase-coupled polymerase chain reaction[126]. Exposure of normal human epidermal keratinocytes (NHEK) to UVB radiation induces oxidative stress and phosphorylation of MAPK. Studies from this laboratory have shown that pretreatment of normal NHEK with EGCG inhibits UVB-induced H2O2 production and H2O2-mediated phosphorylation of MAPK[127].
We recently evaluated the effects of EGCG and TF, on PI3K- and MAPK-pathways in DU145 and LNCaP cells. Both EGCG and TF treatment were found to (1) decrease the levels of PI3K and phospho-Akt; and (2) increase Erk1/2 in both DU145 and LNCaP cells. This study, for the first time, demonstrated the modulation of the constitutive activation of PI3K/Akt and Erk1/2 pathways by EGCG as well as TF[124]. More recently an in vivo study revealed that oral infusion of GTP resulted in significant decrease in (1) the protein levels of PI3K; (2) phosphorylation of Akt; and (3) phosphorylation of ERK1/2 in TRAMP[112].
Modulation of nuclear factor κB by EGCG The nuclear transcription factor NF-κB plays an important role in inflammation, autoimmune response, cell proliferation and apoptosis by regulating the expression of genes involved in these processes and thus, is widely recognized as a critical mediator of immune and inflammatory responses. Constitutive activation of the transcription factor NF-κB has been observed in a high proportion of androgen-independent PCa[128–130]. In addition to suppressing apoptosis, NF-κB promotes malignant behavior in other ways like stimulating transcription of cell cycle progression factors (c-myc, cyclin D1), proteolytic enzymes (MMP-9, uPA), and angiogenic factors VEGF and IL-8[130,131]. Thus, it is not surprising that nuclear localization of NF-κB in PCa biopsies has been shown to correlate with poor clinical prognosis[132]. A mystifying array of natural products, some of which have putative chemo-preventive activity, are reported to inhibit constitutive and/or stimulated NF-κB activity in human PCa. In a study, we demonstrated that EGCG treatment to normal NHEK keratinocytes resulted in a significant dose- and time-dependent inhibition of UVB-mediated activation and nuclear translocation of a NF-κB/p65, suggesting that that EGCG protects against the adverse effects of UV radiation via modulations in the NF-κB pathway[46]. In another study from our laboratory, it was demonstrated that EGCG had a concurrent effect on p53 and NF-κB, causing a change in Bax/Bcl-2 ratio in a way that favors apoptosis in prostate carcinoma LNCaP cells[94].
Effects of tea and its polyphenols on prostate tumor xenografts Athymic nude mice implanted with tumor xenografts have been widely used as an established pre-clinical in vivo model to assess the effect of drugs and/or synthetic agents and dietary substances on the development of a variety of cancer types. Liao and colleagues[133] studied the effects of tea polyphenols on growth inhibition and regression of prostate tumors in athymic nude mice. They found that intraperitoneal injection of EGCG but not structurally related catechins, such as ECG, inhibited the growth and rapidly reduced the size of human prostate PC-3 (androgen-insensitive) and LNCaP 104-R (androgen-repressed) tumors. In a recent study[134], we demonstrated that GTP, black tea extract (BTE), EGCG and TF resulted in significant inhibition in the growth of androgen responsive CWR22Rν1 implanted tumors in athymic nude mice. Further, treatment of mice with all tea preparations resulted in significantly reduced PSA secretion in serum measured at 3 and 4 weeks post implantation of CWR22Rν1 cells. The immuno-blot analyses of the tumors revealed that the prostate tumor growth inhibitory response of all tea preparations was accompanied with (1) decrease in Bcl-2 (anti-apoptotic) with a concomitant increase in Bax (pro-apoptotic); (2) activation of Caspase-3; (3) cleavage of PARP; and (4) decrease in VEGF. This study suggested that GTP and BTE as well as their major polyphenolic constituents EGCG and TF significantly inhibited the development of PCa via (1) induction of apoptosis; and (2) inhibition of angiogenesis in tumors. Furthermore, in an independent experiment, we found that GTP, at very low doses (0.1% and 0.05% in water) resulted in a significant regression of tumors in athymic nude mice when given after CWR22Rν1 tumors were established to a volume of approximately 400 mm3. These data point to a potential therapeutic benefit of black as well as green tea against PCa.
Custom tailoring of chemopreventive regimen for prostate cancer
Cancer is the result of several genetic mutations, which in turn results in defects in multiple signaling pathways being affected. It is therefore unlikely that any single agent may prove to be totally effective and/or beneficial for PCa prevention or treatment in all populations on a long-term basis. We recently advocated the idea of combining naturally occurring agents in a manner that could be more beneficial and at the same time would not exhibit toxicity. It is of interest that several dietary agents that are effective chemopreventive agents in one experimental setting can enhance or have no effects on carcinogenesis in another experimental setting. Thus it is likely that we can custom tailor or personalize the chemopreventive regimen through a cocktail of agents with agents that have known mechanisms targeted for different individual needs. This concept of combining chemopreventive agents to achieve greater benefits is being increasingly appreciated and studies are being conducted in our and other laboratories using a combination of natural agents and chemotherapeutic drugs. To achieve the objective, there is also a need to understand genetic, environmental, and lifestyle factors that influence various populations for whom the chemoprevention intervention is considered. This information could be critical in the selection of an appropriate cancer chemopreventive regimen for individuals with varying risk for PCa development. A combination of different nutraceuticals with varied mechanisms of action could be more effective as they could attack process of carcinogenesis at more than one site or pathway(s) and result in the treatment having an additive or synergistic effect against cancer growth and development. We recently demonstrated the combined inhibitory effects of green tea polyphenols and COX-2 inhibitor on growth of PCa cells in in-vivo and in-vitro situations[135]. This study reported an increased efficacy of selective COX-2 inhibitors in combination with polyphenols from green tea for inhibition of growth of human prostate cancer cells both in vitro and in vivo. A recent study demonstrated that combined treatment with lycopene and vitamin E, at 5 mg/kg BW each, suppressed orthotropic growth of PC-346C prostate tumors by 73% and increased median survival time by 40%[136]. In another study, Venkateswaran et al. demonstrated that selenium potentiates vitamin E-induced inhibition of LNCaP cells and this inhibition was demonstrated by a reduction of cells in the S-phase of cell cycle[137]. A study by Tokar et al[138] examined the effects of synthetic retinoid N-(4-hydroxyphenyl)retinamide (4-HPR) in combination with cholecalciferol (vitamin D3) on growth, and on the expression of vimentin, matrix metalloproteinase-2 (MMP-2), and retinoid and vitamin D receptor expression, using the non-tumorigenic, human prostate epithelial cell line RWPE-1. These results suggested that combined treatment with 4-HPR and cholecalciferol, at doses lower than what might be effective with single agents, increase anti-cancer efficacy.
In light of the observations suggesting more beneficial effects with a combination of chemopreventive agents at low doses, we advocate to design experiments containing a mixture of substances that target multiple signaling pathways.
Epidemiological studies
In recent years, the availability of increasingly sophisticated biochemical approaches of molecular epidemiology has made the process of cancer formation easier to understand and comprehend. Such understanding has offered opportunities for defining the risk to individuals and for modulating this risk by means of agents that can alter critical steps in the multistage process of carcinogenesis. As of yet, only a few case control studies have been conducted to evaluate the effect of consumption of tea for human PCa. All published data seeking an association between tea consumption and the risk of PCa considered undefined tea preparations, mostly black tea. The possible harmful effects of excessive tea consumption, tea consumption at very high temperature, or the consumption of salted tea had not been yet ruled out. Two epidemiological studies have shown that people who regularly consume tea have a lower incidence of PCa[139,140]. Heilbrun et al[139], in a prospective cohort study of 7833 men living in Hawaii with Japanese ancestry, observed a weak but significant negative association between black tea intake (more than one cup per day) and PCa incidence (P= 0.02). In a case-control study conducted in 3 geographical areas of Canada, Jain et al[140] observed a decrease in PCa risk with an intake of more than 2 cups of tea per day. Other epidemiological studies conducted in Italy[141], Utah[142] and Canada[143] did not find any difference of risk for PCa between tea drinkers and non-drinkers. It should be noted that most of these studies lacked appropriate controls for comparison in categorization of tea consumption, the type of tea consumed and the ethnicity of the subjects, which weakens the overall effect of the study.
Recently, to investigate whether green tea consumption has an etiological association with PCa, a case-control study was conducted in Hangzhou, southeast China during 2001–2002[144]. In this study, 130 incident patients with histologically confirmed adenocarcinoma of the prostate and 274 hospital inpatients without PCa or any other malignant diseases and matched to the age of cases were monitored. Information on duration, quantity and frequency of usual tea consumption, as well as the number of new batches brewed per day, were collected by face-to-face interview using a structured questionnaire. The risk of PCa for tea consumption was assessed using multivariate logistic regression adjusting for age, locality, education, income, body mass index, physical activity, alcohol consumption, tobacco smoking, total fat intake, marital status, age at marriage, number of children, history of vasectomy and family history of PCa. Among the cases, 55.4% were tea drinkers compared to 79.9% for the controls and almost all the tea consumed was green tea. The PCa risk was found to be declined with increasing frequency, duration and quantity of green tea consumption. The dose response relationships were also significant, suggesting that green tea imparts chemopreventive effects against PCa in humans[144].
Clinical trials with green tea
A phase I trial of green tea extract in patients with solid tumors was conducted to ascertain the maximum tolerated dose, toxicity, and pharmacology of oral green tea extract. This study concluded that 1.0 g/m2 of green tea extract three times a day [equivalent to 7 to 8 Japanese cups (120 mL) of green tea] is well tolerated and is not associated with any side effects[145]. One recent phase II clinical trial explored the anti-neoplastic effects of green tea in patients with metastatic androgen-independent PCa[146]. In this study, forty-eight patients were instructed to take 6 g of green tea per day orally in 6 divided doses. Results from this study showed that only one patient within this 42-patient cohort manifested a 50% decline in PSA values from baseline, but this reduction was not sustained beyond 2 months. Although green tea was tolerated well, a notable percentage of patients experienced adverse effects such as insomnia, fatigue etc., presumably from caffeine present in the tea. It should however, be noted that this study was conducted in patients with metastatic androgen-independent PCa and therefore, in principle, is not a representation of the chemopreventive effects of green tea. Furthermore, the median time on this study was only one month, whereas, prior pre-clinical data have been suggesting that green tea requires prolonged exposure to exert its antitumor activity[110,112]. Taken into consideration the drawbacks of this trial, the negative findings of this study do not contradict the results of earlier epidemiological studies, which suggest that green tea may confer antitumor effects in a relatively healthy population. Thus, for an ideal prospective study, a population with high risk for PCa development should be considered and the length of exposure of green tea to the subjects should be taken into consideration.
Bettuzzi et al[147] recently carried out a one-year proof-of-principle clinical trial to assess the safety and efficacy of GTCs for the chemoprevention of CaP in high-grade prostate intraepithelial neoplasia (HG-PIN) volunteers. Sixty volunteers with HG-PIN, were enrolled in this double-blind, placebo-controlled study. Daily treatment consisted of three GTCs capsules, 200 mg each (total 600 mg/d). After one year, only one tumor was diagnosed among the 30 GTC-treated men (incidence, approximately 3%), whereas nine cancers were found among the 30 placebo-treated men (incidence, 30%). Total prostate-specific antigen did not change significantly between the two arms, but GTCs-treated men showed values constantly lower with respect to placebo-treated ones. International Prostate Symptom Score and quality of life scores of GTC-treated men with coexistent benign prostate hyperplasia (BPH) improved, reaching statistical significance in the case of the International Prostate Symptom Scores. No significant side effects or adverse effects were documented. This is the first study showing that GTCs are safe and very effective for treating premalignant lesions before PCa develops. As a secondary observation, administration of GTCs was also found to reduce lower urinary tract symptoms, suggesting that these compounds might also be of help for treating the symptoms of BPH. Confirmation of these findings in another group of patients is awaited.
Conclusion and future perspectives
Prostate cancer exists in either a latent, clinically insignificant form or in an aggressive form that progresses to various other body sites. Moreover, it is a complicated malignancy with tremendous heterogenicity of androgen-dependent and androgen-independent forms and because of the co-existence of multiple pathological entities, it has not been possible to provide satisfactory treatment options for this disease. Thus, chemoprevention with a naturally occurring non-toxic agent such as green tea could be useful in the management of this disease.
Recent pre-clinical studies have shown that tea and its constituents inhibit cell cycle, induce apoptosis and modulate multiple signaling pathways in a fashion that encourages the elimination of precancerous and cancerous cells. The uniqueness of the action of tea perhaps lies in its specificity of killing cancer cells without affecting the growth of normal cells. Although studies on tea polyphenols demonstrate its efficacy as a potent chemopreventive agent against PCa, there are still many gaps in our current awareness. There are certain factors such as bio-availability, tissue level of tea constituents, tea type, drinking habit, race, etc. that need to be taken care of before a recommendation could be made for tea polyphenols as preventive or therapeutic agent for human use. The bio-availability of the active polyphenolic constituents after tea consumption by laboratory animals and humans is poorly defined. Yang et al and others[148–150] have measured the concentration of tea polyphenols in human plasma, saliva, feces and urine after consuming decaf-feinated green tea and found that EGCG was in lesser concentration than EGC. Therefore, a great deal of laboratory research and many more epidemiological studies are needed for obtaining conclusive evidence. The increase or decrease of PSA and IGF-1 and IGFBP3 in relation to tea consumption and levels of tea polyphenols in urine samples may be used as biomarkers in PCa chemoprevention. This type of study could answer the question of how much tea should be consumed by humans for PCa chemoprevention and explain the mechanism involved.
Prostate cancer is a disease of many etiological factors and involves several mechanisms in its progression. There-fore, the modulation of a single mechanism alone may not completely impede the progression of PCa. Therefore, a strategy of acting on multiple signaling pathways using a combination of chemopreventive agents should be explored for PCa chemoprevention in pre-clinical studies. For such a type of combination chemoprevention strategy, the agents should be selected based on their mechanism of action in such a way that two or more signaling pathways are attacked with two or more agents to impart a synergistic response against PCa. Because of the promising effects of tea consumption on tumor induction in animals and in a case control study, we recommend that this effect should be examined in a large population.
References
- Jemal A, Siegel R, Ward E, Murray T, Xu J, Thun MJ. Cancer statistics, 2007. CA Cancer J Clin 2007;57:43-66.
- Parkin DM, Bray F, Ferlay J, Pisani P. Global cancer statistics, 2002. CA Cancer J Clin 2005;55:74-108.
- Sim HG, Cheng CW. Changing demography of prostate cancer in Asia. Eur J Cancer 2005;41:834-45.
- Platz EA, Giovannucci E. The epidemiology of sex steroid hormones and their signaling and metabolic pathways in the etiology of prostate cancer. J Steroid Biochem Mol Biol 2004;92:237-53.
- Lowe JF, Frazee LA. Update on prostate cancer chemoprevention. Pharmacotherapy 2006;26:353-9.
- Sikka SC. Role of oxidative stress response elements and antioxidants in prostate cancer pathobiology and chemoprevention – a mechanistic approach. Curr Med Chem 2003;10:2679-92.
- Bostwick DG, Burke HB, Djakiew D, Euling S, Ho SM, Landolph J, et al. Human prostate cancer risk factors. Cancer 2004;101:2371-490.
- Hsing AW, Tsao L, Devesa SS. International trends and patterns of prostate cancer incidence and mortality. Int J Cancer 2000;85:60-7.
- Terry PD, Rohan TE, Wolk A. Intakes of fish and marine fatty acids and the risks of cancers of the breast and prostate and of other hormone-related cancers: a review of the epidemiologic evidence. Am J Clin Nutr 2003;77:532-43.
- Rohrmann S, Platz EA, Kavanaugh CJ, Thuita L, Hoffman SC, Helzlsouer KJ. Meat and dairy consumption and subsequent risk of prostate cancer in a US cohort study. Cancer Causes Control 2007;18:41-50.
- Jani AB, Hellman S, Jani AB, Hellman S. Early prostate cancer: clinical decision-making. Lancet 2003;361:1045-53.
- Lamson DW, Brignall MS. Natural agents in the prevention of cancer, part two: preclinical data and chemoprevention for common cancers. Altern Med Rev 2001;6:167-87.
- Surh YJ. Cancer chemoprevention with dietary phytochemicals. Nat Rev Cancer 2003;3:768-80.
- Schuurman AG, Goldbohm RA, Brants HA, van den Brandt PA. A prospective cohort study on intake of retinol, vitamins C and E, and carotenoids and prostate cancer risk Cancer Causes Control 2002;13:573-82. [Netherlands].
- Klein EA. Selenium: epidemiology and basic science. J Urol 2004; 171: S50–3; discussion S53.
- Siddiqui IA, Adhami VM, Saleem M, Mukhtar H. Beneficial effects of tea and its polyphenols against prostate cancer. Mol Nutr Food Res 2006;50:130-43.
- Khan N, Afaq F, Mukhtar H. Apoptosis by dietary factors: the suicide solution for delaying cancer growth. Carcinogenesis 2007;28:233-9.
- Gupta S, Ahmad N, Mohan RR, Husain MM, Mukhtar H. Prostate cancer chemoprevention by green tea: in vitro and in vivo inhibition of testosterone-mediated induction of ornithine decarboxylase. Cancer Res 1999;59:2115-20.
- Siddiqui IA, Afaq F, Adhami VM, Mukhtar H. Prevention of prostate cancer through custom tailoring of chemopreventive regimen. Chem Biol Interact 2007. Epub ahead of print.
- Lee KW, Lee HJ. The roles of polyphenols in cancer chemo-prevention. Biofactors 2006;26:105-21.
- Aggarwal BB, Shishodia S. Molecular targets of dietary agents for prevention and therapy of cancer. Biochem Pharmacol 2006;71:1397-421.
- Tsao AS, Kim ES, Hong WK. Chemoprevention of cancer. CA Cancer J Clin 2004;54:150-80.
- Sarkar FH, Li Y. The role of isoflavones in cancer chemopreven-tion. Front Biosci 2004;9:2714-24.
- Jian L, Xie LP, Lee AH, Binns CW. Protective effect of green tea against prostate cancer: a case-control study in southeast China. Int J Cancer 2004;108:130-5.
- Klein EA, Thompson IM. Update on chemoprevention of prostate cancer. Curr Opin Urol 2004;14:143-9.
- Thompson IM, Ankerst DP, Chi C, Goodman PJ, Tangen CM, Lucia MS, et al. Assessing prostate cancer risk: results from the Prostate Cancer Prevention Trial. J Natl Cancer Inst 2006;98:529-34.
- Lieberman R. Evidence-based medical perspectives: the evolving role of PSA for early detection, monitoring of treatment response, and as a surrogate end point of efficacy for interventions in men with different clinical risk states for the prevention and progression of prostate cancer. Am J Ther 2004;11:501-6.
- Yamamoto T, Hsu S, Lewis J, Wataha J, Dickinson D, Singh B, et al. Green tea polyphenol causes differential oxidative environments in tumor versus normal epithelial cells. J Pharmacol Exp Ther 2003;307:230-6.
- Chung FL, Schwartz J, Herzog CR, Yang YM. Tea and cancer prevention: studies in animals and humans. J Nutr 2003;133:3268S-3274S.
- Siddiqui IA, Afaq F, Adhami VM, Ahmad N, Mukhtar H. Antioxidants of the beverage tea in promotion of human health. Antioxid Redox Signal 2004;6:571-82.
- Rumpler W, Seale J, Clevidence B, Judd J, Wiley E, Yamamoto S, et al. Oolong tea increases metabolic rate and fat oxidation in men. J Nutr 2001;131:2848-52.
- Yang CS. Tea and health Nutrition 1999;15:946-9.
- Raederstorff DG, Schlachter MF, Elste V, Weber P. Effect of EGCG on lipid absorption and plasma lipid levels in rats. J Nutr Biochem 2003;14:326-32.
- Tokunaga S, White IR, Frost C, Tanaka K, Kono S, Tokudome S, et al. Green tea consumption and serum lipids and lipoproteins in a population of healthy workers in Japan. Ann Epidemiol 2002;12:157-65.
- Babu PV, Sabitha KE, Srinivasan P, Shyamaladevi CS. Green tea attenuates diabetes induced Maillard-type fluorescence and collagen cross-linking in the heart of streptozotocin diabetic rats. Pharmacol Res 2007;55:433-40.
- Tsuneki H, Ishizuka M, Terasawa M, Wu JB, Sasaoka T, Kimura I. Effect of green tea on blood glucose levels and serum proteomic patterns in diabetic (db/db) mice and on glucose metabolism in healthy humans. BMC Pharmacol 2004;4:18.
- Yang CS, Wang ZY. Tea and cancer. Journal of National Cancer Institute 1993;85:1038-49.
- Dufresne CJ, Farnworth ER. A review of latest research findings on the health promotion properties of tea. J Nutr Biochem 2001;12:404-21.
- Ahmad N, Feyes DK, Nieminen AL, Agarwal R, Mukhtar H. Green tea constituent epigallocatechin-3-gallate and induction of apoptosis and cell cycle arrest in human carcinoma cells. J Natl Cancer Inst 1997;89:1881-6.
- Liao S, Kao YH, Hiipakka RA. Green tea: biochemical and biological basis for health benefits. Vitam Horm 2001;62:1-94.
- Yokozawa T, Dong E. Influence of green tea and its three major components upon low-density lipoprotein oxidation. Exp Toxicol Pathol 1997;49:329-35.
- Kao YH, Hiipakka RA, Liao S. Modulation of endocrine systems and food intake by green tea epigallocatechin gallate. Endocrinology 2000;141:980-7.
- Beltz LA, Bayer DK, Moss AL, Simet IM. Mechanisms of cancer prevention by green and black tea polyphenols. Anticancer Agents Med Chem 2006;6:389-406.
- Liang YC, Lin-Shiau SY, Chen CF, Lin JK. Inhibition of cyclin-dependent kinases 2 and 4 activities as well as induction of Cdk inhibitors p21 and p27 during growth arrest of human breast carcinoma cells by (-)-epigallocatechin-3-gallate. J Cell Biochem 1999;75:1-12.
- Ahmad N, Adhami VM, Gupta S, Cheng P, Mukhtar H. Role of the retinoblastoma (pRb)-E2F/DP pathway in cancer chemo-preventive effects of green tea polyphenol epigallocatechin-3-gallate. Arch Biochem Biophys 2002;398:125-31.
- Afaq F, Adhami VM, Ahmad N, Mukhtar H. Inhibition of ultraviolet B-mediated activation of nuclear factor kappaB in normal human epidermal keratinocytes by green tea Constituent (-)-epigallocatechin-3-gallate. Oncogene 2003;22:1035-44.
- Yang GY, Liao J, Kim K, Yurkow EJ, Yang CS. Inhibition of growth and induction of apoptosis in human cancer cell lines by tea polyphenols. Carcinogenesis 1998;19:611-16.
- Chung JY, Huang C, Meng X, Dong Z, Yang CS. Inhibition of activator protein 1 activity and cell growth by purified green tea and black tea polyphenols in H-ras-transformed cells: structure-activity relationship and mechanisms involved. Cancer Res 1999;59:4610-17.
- Yang CS, Maliakal P, Meng X. Inhibition of carcinogenesis by tea. Annu Rev Pharmacol Toxicol 2002;42:25-54.
- Kranse R, Dagnelie PC, van Kemenade MC, de Jong FH, Blom JH, Tijburg LB, et al. Dietary intervention in prostate cancer patients: PSA response in a randomized double-blind placebo-controlled study. Int J Cancer 2005;113:835-40.
- Pu YS, Chiang HS, Lin CC, Huang CY, Huang KH, Chen J. Changing trends of prostate cancer in Asia. Aging Male 2004;7:120-32.
- Hsing AW, Tsao L, Devesa SS. International trends and patterns of prostate cancer incidence and mortality. Int J Cancer 2000;85:60-7.
- Mohan RR, Challa A, Gupta S, Bostwick DG, Ahmad N, Agarwal R, et al. Overexpression of ornithine decarboxylase in prostate cancer and prostatic fluid in humans. Clin Cancer Res 1999;5:143-7.
- Gingrich JR, Barrios RJ, Morton RA, Boyce BF, DeMayo FJ, Finegold MJ, et al. Metastatic prostate cancer in a transgenic mouse. Cancer Res 1996;56:4096-102.
- Gupta S, Hastak K, Ahmad N, Lewin JS, Mukhtar H. Inhibition of prostate carcinogenesis in TRAMP mice by oral infusion of green tea polyphenols. Proc Natl Acad Sci USA 2001;98:10350-5.
- Adhami VM, Ahmad N, Mukhtar H. Molecular targets for green tea in prostate cancer prevention. J Nutr 2003;133:2417S-24S.
- Caporali A, Davalli P, Astancolle S, D’Arca D, Bettuzzi S, et al. The chemopreventive action of catechins in the TRAMP mouse model of prostate carcinogenesis is accompanied by clusterin over-expression. Carcinogenesis 2004;25:2217-24.
- Debruyne F. Hormonal therapy of prostate cancer. Semin Urol Oncol 2002;20:4-9.
- Huggins C, Hodges CV. Studies on prostatic cancer. I. The effect of castration, of estrogen and of androgen injection on serum phosphatases in metastatic carcinoma of the prostate, 1941. J Urol 2002;168:9-12.
- Fluchter SH, Weiser R, Gamper C. The role of hormonal treatment in prostate cancer. Recent Results Cancer Res 2007;175:211-37.
- Long BJ, Grigoryev DN, Nnane IP, Liu Y, Ling YZ, Brodie AM. Antiandrogenic effects of novel androgen synthesis inhibitors on hormone-dependent prostate cancer. Cancer Res 2000;60:6630-40.
- Hiipakka RA, Zhang HZ, Dai W, Dai Q, Liao S. Structure-activity relationships for inhibition of human 5alpha-reductases by polyphenols. Biochem Pharmacol 2002;63:1165-76.
- Koivisto P, Kolmer M, Visakorpi T, Kallioniemi OP. Androgen receptor gene and hormonal therapy failure of prostate cancer. Am J Pathol 1998;152:1-9.
- Ettinger SL, Sobel R, Whitmore TG, Akbari M, Bradley DR, Gleave ME, et al. Dysregulation of sterol response element-binding proteins and downstream effectors in prostate cancer during progression to androgen independence. Cancer Res 2004;64:2212-21.
- Haapala K, Kuukasjarvi T, Hyytinen E, Rantala I, Helin HJ, Koivisto PA. Androgen receptor amplification is associated with increased cell proliferation in prostate cancer. Hum Pathol 2007;38:474-8.
- Palmberg C, Koivisto P, Kakkola L, Tammela TL, Kallioniemi OP, Visakorpi T. Androgen receptor gene amplification at primary progression predicts response to combined androgen blockade as second line therapy for advanced prostate cancer. J Urol 2000;164:1992-5.
- Ren F, Zhang S, Mitchell SH, Butler R, Young CY. Tea polyphenols down-regulate the expression of the androgen receptor in LNCaP prostate cancer cells. Oncogene. 2000;9:1924-32.
- Takane KK, McPhaul MJ. Functional analysis of the human androgen receptor promoter. Mol Cell Endocrinol 1996;119:83-93.
- Chen S, Supakar PC, Vellanoweth RL, Song CS, Chatterjee B, Roy AK. Functional role of a conformationally flexible homopurine/homopyrimidine domain of the androgen receptor gene promoter interacting with Sp1 and a pyrimidine single strand DNA-binding protein. Mol Endocrinol 1997;11:3-15.
- Yuan H, Gong A, Young CY. Involvement of transcription factor Sp1 in quercetin-mediated inhibitory effect on the androgen receptor in human prostate cancer cells. Carcinogenesis 2005;26:793-801.
- Philipsen S, Suske G. A tale of three fingers: the family of mammalian Sp/XKLF transcription factors. Nucleic Acids Res 1999;27:2991-3000.
- Peracaula R, Tabares G, Royle L, Harvey DJ, Dwek RA, Rudd PM, et al. Altered glycosylation pattern allows the distinction between prostate-specific antigen (PSA) from normal and tumor origins. Glycobiology 2003;13:457-70.
- Miller N, Smolkin ME, Bissonette E, Theodorescu D. Undetectable prostate specific antigen at 6–12 months: a new marker for early success in hormonally treated patients after prostate brachytherapy. Cancer 2005;103:2499-506.
- Grossfeld GD, Carroll PR. Prostate cancer early detection: a clinical perspective. Epidemiol Rev 2001;23:173-80.
- Yuan Q, Ray RM, Viar MJ, Johnson LR. Polyamine regulation of ornithine decarboxylase and its antizyme in intestinal epithelial cells. Am J Physiol Gastrointest Liver Physiol 2001;280:G130-8.
- Ray RM, McCormack SA, Covington C, Viar MJ, Zheng Y, Johnson LR. The requirement for polyamines for intestinal epithelial cell migration is mediated through Rac1. J Biol Chem 2003;278:13039-46.
- Poulin R, Pelletier G, Pegg AE. Induction of apoptosis by excessive polyamine accumulation in ornithine decarboxylase-overproducing L1210 cells. Biochem J 1995;311:723-7.
- Tobias KE, Kahana C. Exposure to ornithine results in excessive accumulation of putrescine and apoptotic cell death in ornithine decarboxylase-overproducing mouse myeloma cells. Cell Growth Differ 1995;6:1279-85.
- Auvinen M, Laine A, Paasinen-Sohns A, Kangas A, Kangas L, Saksela O, et al. Human Ornithine decarboxylase-overproducing NIH3T3 cells induce rapidly growing, highly vascularized tumors in nude mice. Cancer Res 1997;57:3016-25.
- Kubota S, Kiyosawa H, Nomura Y, Yamada T, Seyama Y. Ornithine decarboxylase overexpression in mouse 10T1/2 fibroblasts: cellular transformation and invasion. J Natl Cancer Inst 1997;89:567-71.
- Palavan-Unsal N, Aloglu-Senturk SM, Arsan D. The function of polyamine metabolism in prostate cancer. Exp Oncol 2006;28:178-86.
- Zeegers MP, Ostrer H. Genes in the polyamine biosynthesis pathway may be involved in prostate cancer susceptibility. Future Oncol 2005;1:683-8.
- Ahmad N, Gilliam AC, Katiyar SK, O’Brien TG, Mukhtar H. A definitive role of ornithine decarboxylase in photocarcino-genesis. Am J Pathol 2001;159:885-92.
- Kadmon D. Chemoprevention in prostate cancer: the role of difluoromethylornithine J Cell Biochem 1992;16H:122-7. [DFMO].
- Heston WD. Prostatic polyamines and polyamine targeting as a new approach to therapy of prostatic cancer. Cancer Surv 1991;11:217-38.
- Bachrach U, Wang YC. Cancer therapy and prevention by green tea: role of ornithine decarboxylase. Amino Acids 2002;22:1-13.
- Bai G, Kasper S, Matusik RJ, Rennie PS, Moshier JA, Krongrad A. Androgen regulation of the human ornithine decarboxylase promoter in prostate cancer cells. J Androl 1998;19:127-35.
- Asadi F, Faraj M, Malakouti S, Kukreja SC. Effect of parathyroid hormone related protein, and dihydrotestosterone on proliferation and ornithine decarboxylase mRNA in human prostate cancer cell lines. Int Urol Nephrol 2001;33:417-22.
- Paul B, Hayes CS, Kim A, Athar M, Gilmour SK. Elevated polyamines lead to selective induction of apoptosis and inhibition of tumorigenesis by (-)-epigallocatechin-3-gallate (EGCG) in ODC/Ras transgenic mice. Carcinogenesis. 2005;26:119-24.
- Wang SI, Mukhtar H. Gene expression profile in human prostate LNCaP cancer cells by (—)epigallocatechin-3-gallate. Cancer Lett 2002;182:43-51.
- Meyers FJ, Gumerlock PH, Chi SG, Borchers H, Deitch AD, deVere White RW. Very frequent p53 mutations in metastatic prostate carcinoma and in matched primary tumors. Cancer 1998;83:2534-9.
- Navone NM, Troncoso P, Pisters LL, Goodrow TL, Palmer JL, Nichols WW, et al. p53 protein accumulation and gene mutation in the progression of human prostate carcinoma. J Natl Cancer Inst 1993;85:1657-69.
- Gupta S, Ahmad N, Nieminen AL, Mukhtar H. Growth inhibition, cell-cycle dysregulation, and induction of apoptosis by green tea constituent [-]-epigallocatechin-3-gallate in androgen-sensitive and androgen-insensitive human prostate carcinoma cells. Toxicol Appl Pharmacol 2000;164:82-90.
- Hastak K, Gupta S, Ahmad N, Agarwal MK, Agarwal ML, Mukhtar H. Role of p53 and NF-kappaB in epigallocatechin-3-gallate induced apoptosis of LNCaP cells. Oncogene 2003;22:4851-9.
- Van Cruchten S, Van Den Broeck W. Morphological and biochemical aspects of apoptosis, oncosis and necrosis. Anat Histol Embryol 2002;31:214-23.
- Proskuryakov SY, Konoplyannikov AG, Gabai VL. Necrosis: a specific form of programmed cell death? Exp Cell Res 2003;283:1-16.
- Kroemer G, Petit P, Zamzami N, Vayssiere JL, Mignotte B. The biochemistry of programmed cell death. FASEB J 1995;9:1277-87.
- Evan GI, Vousden KH. Proliferation, cell cycle and apoptosis in cancer. Nature 2001;411:342-8.
- Denmeade SR, Lin XS, Isaacs JT. Role of programmed (apoptotic) cell death during the progression and therapy for prostate cancer. Prostate 1996;28:251-65.
- Tang DG, Porter AT. Target to apoptosis: a hopeful weapon for prostate cancer. Prostate 1997;32:284-93.
- Hussain M, Banerjee M, Sarkar FH, Djuric Z, Pollak MN, Doerge D, et al. Soy isoflavones in the treatment of prostate cancer. Nutr Cancer 2003;47:111-7.
- Chiao JW, Wu H, Ramaswamy G, Conaway CC, Chung FL, Wang L, et al. Ingestion of an isothiocyanate metabolite from cruciferous vegetables inhibits growth of human prostate cancer cell xenografts by apoptosis and cell cycle arrest. Carcinogenesis 2004;25:1403-8.
- Saleem M, Kweon MH, Yun JM, Adhami VM, Khan N, Syed DN, et al. A novel dietary triterpene Lupeol induces fas-mediated apoptotic death of androgen-sensitive prostate cancer cells and inhibits tumor growth in a xenograft model. Cancer Res 2005;65:11203-13.
- Stuart EC, Scandlyn MJ, Rosengren RJ. Role of epigallocatechin gallate (EGCG) in the treatment of breast and prostate cancer. Life Sci 2006;79:2329-36.
- Chung LY, Cheung TC, Kong SK, Fung KP, Choy YM, Chan ZY, et al. Induction of apoptosis by green tea catechins in human prostate cancer DU145 cells. Life Sci 2001;68:1207-14.
- Huang S, New L, Pan Z, Han J, Nemerow GR. Urokinase plasminogen activator/urokinase-specific surface receptor expression and matrix invasion by breast cancer cells requires constitutive p38alpha mitogen-activated protein kinase activity. J Biol Chem 2000;275:12266-72.
- Liotta LA, Tryggvason K, Garbisa S, Hart I, Foltz CM, Shafie S. Metastatic potential correlates with enzymatic degradation of basement membrane collagen. Nature 1980;284:67-8.
- Garbisa S, Sartor L, Biggin S, Salvato B, Benelli R, Albini A. Tumor gelatinases and invasion inhibited by the green tea flavanol epigallocatechin-3-gallate. Cancer 2001;91:822-32.
- Jung YD, Ellis LM. Inhibition of tumour invasion and angiogenesis by epigallocatechin gallate (EGCG), a major component of green tea. Int J Exp Pathol 2001;82:309-16.
- Singh AK, Seth P, Anthony P, Husain MM, Madhavan S, Mukhtar H, et al. Green tea constituent epigallocatechin-3-gallate inhibits angiogenic differentiation of human endothelial cells. Arch Biochem Biophys 2002;401:29-37.
- Rodriguez SK, Guo W, Liu L, Band MA, Paulson EK, Meydani M. Green tea catechin, epigallocatechin-3-gallate, inhibits vascular endothelial growth factor angiogenic signaling by disrupting the formation of a receptor complex. Int J Cancer 2006;118:1635-44.
- Adhami VM, Siddiqui IA, Ahmad N, Gupta S, Mukhtar H. Oral consumption of green tea polyphenols inhibits insulin-like growth factor-I-induced signaling in an autochthonous mouse model of prostate cancer. Cancer Res 2004;64:8715-22.
- Zhou JR, Yu L, Zhong Y, Blackburn GL. Soy phytochemicals and tea bioactive components synergistically inhibit androgen-sensitive human prostate tumors in mice. J Nutr 2003;133:516-21.
- Furstenberger G, Senn HJ. Insulin-like growth factors and cancer. Lancet Oncol 2002;3:298-302.
- Yu H, Rohan T. Role of the insulin-like growth factor family in cancer development and progression. J Natl Cancer Inst 2000;92:1472-89.
- Kaplan PJ, Mohan S, Cohen P, Foster BA, Greenberg NM. The insulin-like growth factor axis and prostate cancer: lessons from the transgenic adenocarcinoma of mouse prostate (TRAMP) model. Cancer Res 1999;59:2203-9.
- Fernandez PL, Hernandez L, Farre X, Campo E, Cardesa A. Alterations of cell cycle-regulatory genes in prostate cancer. Pathobiology 2002;70:1-10.
- Davis JN, Day ML. The convergence of hormone regulation and cell cycle in prostate physiology and prostate tumorigenesis. Mol Biotechnol 2002;22:129-38.
- Lee MH, Yang HY. Negative regulators of cyclin-dependent kinases and their roles in cancers. Cell Mol Life Sci 2001;58:1907-22.
- Vermeulen K, Van Bockstaele DR, Berneman ZN. The cell cycle: a review of regulation, deregulation and therapeutic targets in cancer. Cell Prolif 2003;36:131-49.
- Toogood PL. Cyclin-dependent kinase inhibitors for treating cancer. Med Res Rev 2001;21:487-98.
- Lin JK, Liang YC, Lin-Shiau SY. Cancer chemoprevention by tea polyphenols through mitotic signal transduction blockade. Biochem Pharmacol 1999;58:911-5.
- Gupta S, Hussain T, Mukhtar H. Molecular pathway for (-)-epigallocatechin-3-gallate-induced cell cycle arrest and apoptosis of human prostate carcinoma cells. Arch Biochem Biophys 2003;410:177-85.
- Siddiqui IA, Adhami VM, Afaq F, Ahmad N, Mukhtar H. Modulation of phosphatidylinositol-3-kinase/protein kinase B- and mitogen-activated protein kinase-pathways by tea polyphenols in human prostate cancer cells. J Cell Biochem 2004;91:232-42.
- Van de Sande T, De Schrijver E, Heyns W, Verhoeven G, Swinnen JV. Role of the phosphatidylinositol 3'-kinase/PTEN/Akt kinase pathway in the overexpression of fatty acid synthase in LNCaP prostate cancer cells. Cancer Res 2002;62:642-6.
- Yu R, Jiao JJ, Duh JL, Gudehithlu K, Tan TH, Kong AN. Activation of mitogen-activated protein kinases by green tea polyphenols: potential signaling pathways in the regulation of antioxidant-responsive element-mediated phase II enzyme gene expression. Carcinogenesis 1997;18:451-6.
- Katiyar SK, Afaq F, Azizuddin K, Mukhtar H. Inhibition of UVB-induced oxidative stress-mediated phosphorylation of mitogen-activated protein kinase signaling pathways in cultured human epidermal keratinocytes by green tea polyphenol (-)-epigallocatechin-3-gallate. Toxicol Appl Pharmacol 2001;176:110-7.
- Gasparian AV, Yao YJ, Kowalczyk D, Lyakh LA, Karseladze A, Slaga TJ, et al. The role of IKK in constitutive activation of NF-kappaB transcription factor in prostate carcinoma cells. J Cell Sci 2002;115:141-51.
- Ismail HA, Lessard L, Mes-Masson AM, Saad F. Expression of NF-kappaB in prostate cancer lymph node metastases. Prostate 2004;58:308-13.
- Suh J, Rabson AB. NF-kappaB activation in human prostate cancer: important mediator or epiphenomenon? J Cell Biochem 2004;91:100-17.
- Hodge JC, Bub J, Kaul S, Kajdacsy-Balla A, Lindholm PF. Requirement of RhoA activity for increased nuclear factor kappaB activity and PC-3 human prostate cancer cell invasion. Cancer Res 2003;63:1359-64.
- Lessard L, Mes-Masson AM, Lamarre L, Wall L, Lattouf JB, Saad F. NF-kappa B nuclear localization and its prognostic significance in prostate cancer. BJU Int 2003;91:417-20.
- Liao S, Umekita Y, Guo J, Kokontis JM, Hiipakka RA. Growth inhibition and regression of human prostate and breast tumors in athymic mice by tea epigallocatechin gallate. Cancer Lett 1995;96:239-43.
- Siddiqui IA, Zaman N, Aziz MH, Reagan-Shaw SR, Sarfaraz S, Adhami VM, et al. Inhibition of CWR22Rnu1 tumor growth and PSA secretion in athymic nude mice by green and black teas. Carcinogenesis 2006;27:833-9.
- Adhami VM, Malik A, Zaman N, Sarfaraz S, Siddiqui IA, Syed DN, et al. Combined inhibitory effects of green tea polyphenols and selective cyclooxygenase-2 inhibitors on the growth of human prostate cancer cells both in vitro and in vivo. Clin Cancer Res 2007;13:1611-9.
- Limpens J, Schroder FH, de Ridder CM, Bolder CA, Wildhagen MF, Obermuller-Jevic UC, et al. Combined lycopene and vitamin E treatment suppresses the growth of PC-346C human prostate cancer cells in nude mice J Nutr 2006;136:1287-93.
- Venkateswaran V, Fleshner NE, Klotz LH. Synergistic effect of vitamin E and selenium in human prostate cancer cell lines. Prostate Cancer Prostatic Dis 2004;7:54-6.
- Tokar EJ, Ancrile BB, Ablin RJ, Webber MM. Cholecalciferol (vitamin D3) and the retinoid N-(4-hydroxyphenyl)retinamide (4-HPR) are synergistic for chemoprevention of prostate cancer. J Exp Ther Oncol 2006;5:323-33.
- Heilbrun LK, Nomura A, Stemmermann GN. Black tea consumption and cancer risk: a prospective study. Br J Cancer 1986;54:677-83.
- Jain MG, Hislop GT, Howe GR, Burch JD, Ghadirian P. Alcohol and other beverage use and prostate cancer risk among Canadian men. Int J Cancer 1998;78:707-11.
- La Vecchia C, Negri E, Franceschi S, D’Avanzo B, Boyle P. Tea consumption and cancer risk. Nutr Cancer 1992;17:27-31.
- Slattery ML, West DW. Smoking, alcohol, coffee, tea, caffeine, and theobromine: risk of prostate cancer in Utah (United States). Cancer Causes Control 1993;4:559-63.
- Ellison LF. Tea and other beverage consumption and prostate cancer risk: a Canadian retrospective cohort study. Eur J Cancer Prev 2000;9:125-30.
- Jian L, Xie LP, Lee AH, Binns CW. Protective effect of green tea against CaP: a case-control study in southeast China. Int J Cancer 2004;108:130-5.
- Pisters KM, Newman RA, Coldman B, Shin DM, Khuri FR, Hong WK, et al. Phase I trial of oral green tea extract in adult patients with solid tumors. J Clin Oncol 2001;19:1830-8.
- Jatoi A, Ellison N, Burch AP, Sloan JA, Dakhil RS, Novotny P, et al. A phase II trial of green tea in the treatment of patients with androgen independent metastatic prostate carcinoma. Cancer 2003;97:1441-6.
- Bettuzzi S, Brausi M, Rizzi F, Castagnetti G, Peracchia G, Corti A. Chemoprevention of human prostate cancer by oral administration of green tea catechins in volunteers with high-grade prostate intraepithelial neoplasia: a preliminary report from a one-year proof-of-principle study. Cancer Res 2006;66:1234-40.
- Yang CS, Chen L, Lee MJ, Balentine D, Kuo MC, Schantz SP. Blood and urine levels of tea catechins after ingestion of different amounts of green tea by human volunteers. Cancer Epidemiol Biomarkers Prev 1998;7:351-4.
- Yang CS, Lee MJ, Chen L. Human salivary tea catechin levels and catechin esterase activities: implications in human cancer prevention studies. Cancer Epidemiol Biomarkers Prev 1999;8:83-9.
- He YH, Kies C. Green and black tea consumption by humans: impact on polyphenol concentrations in feces, blood, and urine. Plant Foods Hum Nutr 1994;46:221-9.