5-HT1A/7 receptor agonist excites cardiac vagal neurons via inhibition of both GABAergic and glycinergic inputs1
Introduction
Heart rate and cardiac functions are under dual control of the sympathetic and the parasympathetic nerves, and the activity of the cardiac parasympathetic nerves dominates in this balance[1,2]. Anatomical and functional studies indicated that 5-hydroxytryptamine (5-HT) is involved in the reflex parasympathetic control of cardiac vagal tone. Immunochemical studies demonstrated that the nucleus ambiguus (NA) and the dorsal motor nucleus of the vagus (DMV), the sites at which cardiac vagal preganglionic neurons (CVPN) are primarily located[3–6], are densely innervated by 5-HT immunoreactive terminals[7,8], and terminal buttons containing 5-HT make synaptic contact with CVPN[9,10]. There are also binding sites for 5-HT1A/7 agonists in both the NA and the DMV in cats[11], rats[12,13], and humans[14]. The activation of central 5-HT1A/7 receptors caused a vagally-mediated bradycardia in cats[9,15–18] and rats[19,20]. The ionophoretic application of 8-hydroxy-2-(di-N-propylamino) tetralin (8-OH-DPAT), an agonist of 5-HT1A/7 receptors, activated CVPN at larger electrophoretic currents in cats, which was attenuated by the co-application of the 5-HT1A/7 receptor antagonist WAY-100635[21]. The blockade of central 5-HT1A/7 receptors attenuated the reflex activation of CVPN[21–24].
The synaptic mechanism that the 5-HT1A/7 receptor agonist activates CVPN and 5-HT1A/7 receptor antagonist attenuates the reflex control of cardiac vagal tone is not clear at the level of CVPN. Since the activation of 5-HT1A/7 receptors is generally known to be inhibitory via opening hyperpolarizing K+ channels[25] and the inhibition of Na+ channels[26], Wang and Ramage[21] assumed that the 5-HT1A/7 agonist might excite CVPN via “disinhibition” of their GABAergic inputs. CVPN receive both GABAergic and glycinergic inhibitory inputs[27]. At least some of the GABAergic inputs originate from the nucleus tractus solitarius (NTS) and can be activated by stimulation of this nucleus[28]; the stimulation-evoked GABAergic currents, compared with the spontaneous GABAergic synaptic currents, are commonly believed to be more closely related to the reflex control of these neurons. In a recent study, the 5-HT1A/7 agonist was found to inhibit the spontaneous GABAergic synaptic currents of CVPN[29], but its effect on the stimulation-evoked GABAergic currents has not been examined. In addition, whether the glycinergic inputs are also involved in the 5-HT receptor-mediated excitation of CVPN is not known. Thus, the purpose of the present study is to test the hypothesis that the 5-HT1A/7 receptor agonist excites CVPN via the inhibition of both the GABAergic and the glycinergic inputs.
Materials and methods
Retrograde fluorescent labeling of CVPN Inhalation agent halothane (0.5 mL) was dripped into a glass box (5×5×5 cm) with a lid and a cotton pad at the bottom of the box. Sprague–Dawley rats (Shanghai Institute for Family Planning, Shanghai, China) that were 3–4 d old were put in the box for 30 s with the lid closed. This procedure anesthetized the rats, but kept their breathing at a relatively normal state. When the rats had no response to a needle puncturing the limbs, the body was buried in ice-filled bags to decrease the body temperature and slow the heart rate. After automatic breathing ceased (usually within 2 min), the animals were placed on an ice-filled bag in a supine posture; a right thoracotomy was made to expose the heart. A glass pipette (tip diameter, 30 µm) filled with 1% rhodamine (XRITC, Molecular Probes, Carlsbad, CA, USA) was gently punctured into the fat pad at the base of the heart and 5 µL solution was injected in a 20 s period. The fat pad of the heart contains the major cardiac parasympathetic ganglions, and neurons in these ganglions receive innervations from the terminals of CVPN[30]. The application of fluorescent tracers at the terminals of CVPN has been a well-established method to retrogradely label CVPN[29]. Special care was taken to avoid rhodamine leaking into the cardiac sac, which could lead to the mislabeling of the brain stem neurons innervating the tissues near the cardiac sac[31]. The incision was sutured and the animals were heated with a thermo-pad to help recovery. During the whole surgery period (approximately 5 min), the body temperature of the animals was approximately 10 ℃, and the animals had no automatic breathing and did not struggle to breathe. After the surgery, the animals usually started automatic breathing within 3 min and started moving freely within a further 5 min. The animals were allowed 1–2 d to recover. The experiments were performed at when the animals were 4.4±0.2 d old (n=43). Rats at this age have been shown to have similar respiratory-related and reflex-related parasympathetic heart rate control as adult rats[32,33].
Slice preparation The animals were anesthetized deeply with halothane and decapitated at the supracollicular level. The hind brain was exposed, isolated, and immerged in cold (4 ℃) artificial cerebral spinal fluid (ACSF) of the following composition (in mmol/L): NaCl (124), KCl (3), KH2PO4 (1.2), CaCl2 (2.4), MgSO4 (1.3), NaHCO3 (26), D-glucose (10), and sucrose (10), and constantly bubbled with gas (95% O2, 5% CO2) at pH 7.4. The cerebellum was removed and the brain stem was dissected using a dissection microscope. The brain stem was then secured in the slicing chamber of a vibratome (Leica VT 1000 S), filled with the same ACSF, and sequentially sectioned at 400 µm thickness in the transverse plane. The slice was transferred into the recording chamber and submerged in the ACSF and maintained at a temperature of 20 ℃.
Electrophysiological recording Individual CVPN in the NA were identified by the presence of the fluorescent tracer using an Olympus upright microscope through a 40× water immersion objective. The pipette resistance (2–5 MΩ) and capacitance were not compensated either before or after gaining intracellular access. To access the glutamatergic spontaneous excitatory postsynaptic currents (sEPSC) the patch pipettes were filled with a solution consisting of (in mmol/L): K+ gluconate (150), HEPES (10), EGTA (10), CaCl2 (1), and MgCl2 (1) at pH 7.3. The cells were clamped at –80 mV. With this pipette solution and the holding voltage, the Cl–-mediated inhibitory synaptic currents were minimized and only excitatory synaptic events were detectable. To access the GABAergic and the glycinergic synaptic currents, the patch pipettes were filled with a solution consisting of (in mmol/L): KCl (150), MgCl2 (2), EGTA (2), HEPES (10), and Mg-ATP (2) at pH 7.3. With this pipette solution, the Cl- current induced by the activation of the GABAergic receptors and the glycinergic receptors was recorded as an inward current. The osmolarity of the ACSF and pipette solutions was adjusted to 320 mosm/L before use. A 200 ms, 5 mV hyperpolarizing voltage was applied at an interval of 4 s for calculating the input resistance. In some experiments, a pair of bipolar tungsten electrodes (tip diameter, 5 μm; distance between tips, 30 µm) was placed in the dorsomedial subnucleus of the ipsilateral NTS. Single square wave currents (1–2 ms duration, 10–70 μA) were injected at an interval of 2 s to evoke GABAergic currents in CVPN. The patch-clamp signal was amplified with an Axopatch 200B amplifier (sampling frequency, 10 kHz; filter frequency, 1 kHz), digitized with 1322A Digidata, and collected with Clampex 9.0 software (Axon instruments, USA). All animal procedures were performed in compliance with the institutional guidelines at Fudan University (Shanghai, China), and were in accordance with the internationally accepted ethical standards for the experimental use of animals.
Drug application The drugs were either globally used in the bath or focally applied through a puffer pipette positioned within 10 µm of the patched neuron. Strychnine (1 µmol/L) and picrotoxin (10 µmol/L) were used to block glycine receptors and GABA receptors, respectively. 6-Cyano-7-nitroquinoxaline-2, 3-dione (CNQX; 50 µmol/L) and D-2-amino-5-phosphonovalerate (AP5; 50 µmol/L) were used to block non-NMDA and NMDA glutamate receptors, respectively. The GABAergic synaptic currents were isolated by the inclusion of AP5 (50 µmol/L), CNQX (50 µmol/L), and strychnine (1µmol/L) in the perfusate. The glycinergic synaptic currents were isolated by the inclusion of AP5 (50 µmol/L), CNQX (50 µmol/L), and picrotoxin (10 µmol/L) in the perfusate. In the experiments that examined miniature inhibitory postsynaptic currents (mIPSC), tetrodotoxin (1 µmol/L) was included in the bath, and the concentration of KCl was increased to 23 mmol/L to increase the frequency of mIPSC[27]. In some experiments, exogenous GABA (100 µmol/L) or glycine (100 µmol/L) was focally applied (100 ms duration) in the presence of TTX to evoke GABAergic or glycinergic currents, respectively. WAY-100635 (100 nmol/L and 1 µmol/L) was used to block 5-HT1A/7 receptors. 8-OH-DPAT (1 nmol/L–10 µmol/L), of which the affinity for 5-HT1A/7 receptors is over 100 times stronger than that of 5-HT[34], was used to activate the 5-HT1A/7 receptors. The duration of 8-OH-DPAT application was 2 min, and each slice was applied only once to avoid desensitization. All of the drugs were purchased from Sigma–Aldrich (St Louis, MO, USA).
Data analysis Spontaneous or miniature synaptic currents were analyzed with MiniAnalysis (version 4.3.1, Synaptosoft), with a minimally acceptable amplitude at 10 pA. The data from a 2 min period before 8-OH-DPAT application and the data from a 30 s period during the maximal response were averaged for comparison. The results are presented as mean±SEM, and statistically compared with paired Student’s t-test or ANOVA followed by post-test Bonferroni correction when appropriate. Significant difference was set at P<0.05.
Results
8-OH -DPAT inhibited the GABAergic synaptic currents At all of the concentrations tested (10 nmol/L, 100 nmol/L, 1 µmol/L, and 10 µmol/L), 8-OH-DPAT significantly decreased the frequency of the GABAergic spontaneous inhibitory postsynaptic currents (sIPSC; paired Student’s t-test) as shown in Figure 1A. The frequency decreases (measured in percentage of controls) caused by 100 nmol/L, 1 µmol/L, and 10 µmol/L 8-OH-DPAT were not significantly different, but the frequency decrease caused by 10 µmol/L 8-OH-DPAT was significantly larger compared with that caused by 10 nmol/L 8-OH-DPAT (ANOVA, Bonferroni), as shown in Figure 1B. The amplitude of the GABAergic sIPSC was not significantly changed by 8-OH-DPAT at 10 nmol/L, but was significantly decreased by 8-OH-DPAT at 100 nmol/L, 1 µmol/L, and 10 µmol/L (paired Student’s t-test), as shown in Figure 1C,1D. These results indicated that both the frequency inhibition and the amplitude inhibition of the GABAergic sIPSC by 8-OH-DPAT had dose-dependent tendencies. 8-OH-DPAT also caused an amplitude decrease of the GABAergic currents evoked by stimulation of the NTS. 8-OH-DPAT (1 µmol/L) inhibited the amplitude of the stimulation-evoked GABAergic synaptic currents by 55% (paired Student’s t-test; P<0.05, n=6). An experiment is shown in Figure 1E–1H, which shows that 8-OH-DPAT caused decreases in the frequency and the amplitude of the GABAergic sIPSC and caused a simultaneous amplitude decrease of the stimulation-evoked GABAergic synaptic currents. The responses of the GABAergic sIPSC to 8-OH-DPAT usually started within 15 s, reached the nadir within 30 s and 2 min, lasted for 10–40 min, and were reversible. At any concentration, 8-OH-DPAT did not alter the baseline current and did not cause a significant change in the input resistance. The input resistance measured before and after the application of 1 µmol/L 8-OH-DPAT was 227.61±12.63 and 230.12±15.34 MΩ, respectively (P>0.05, n=7; paired Student’s t-test). At the end of each experiment 10 µmol/L picrotoxin abolished the spontaneous or the evoked GABAergic synaptic currents.
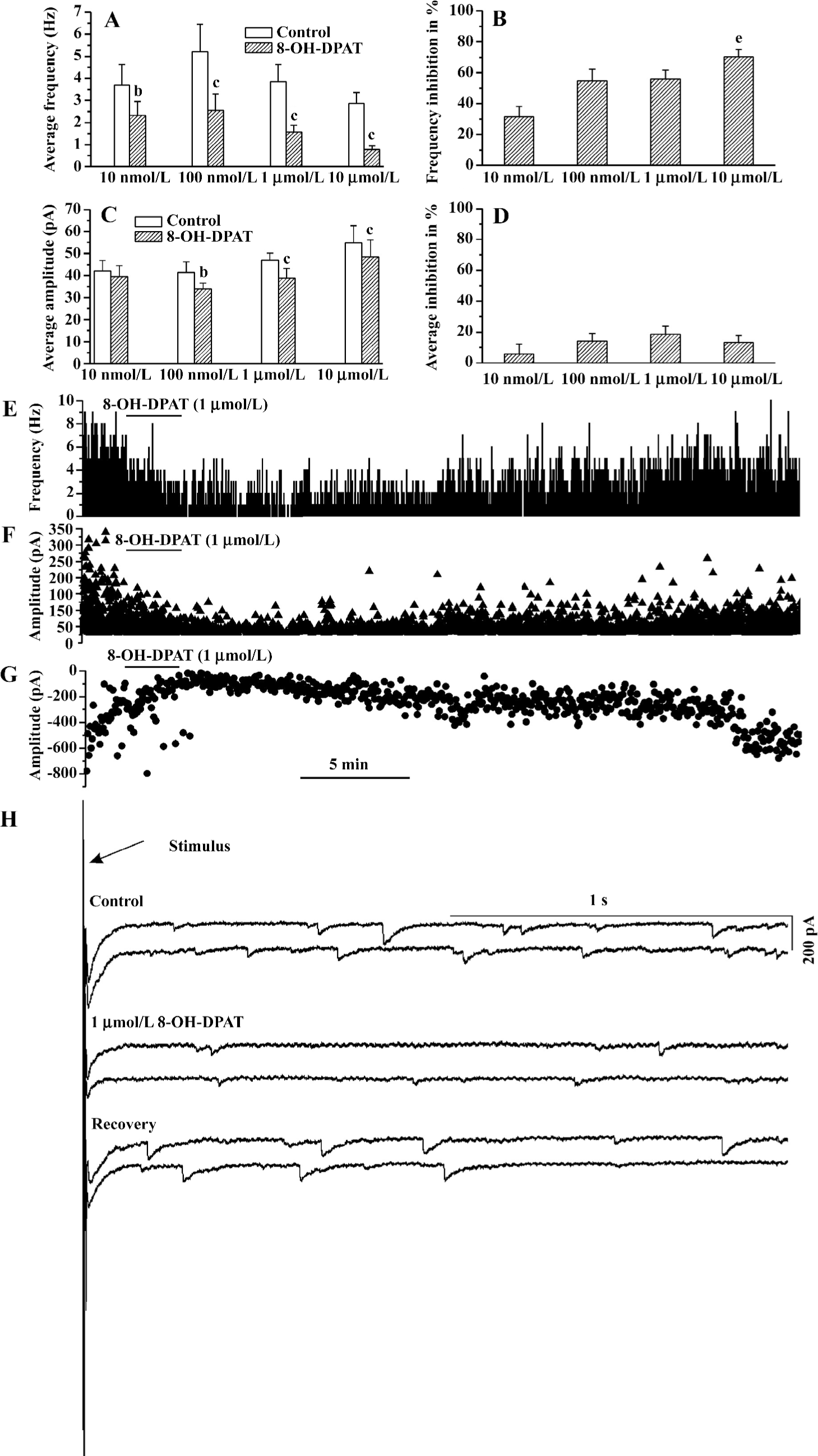
8-OH-DPAT inhibited glycinergic sIPSC The glycinergic sIPSC were more sensitive to 8-OH-DPAT. 8-OH-DPAT significantly decreased the frequency of the glycinergic sIPSC at a concentration as low as 1 nmol/L (Figure 2A). The frequency decrease (measured in percentage of controls) caused by 1 µmol/L 8-OH-DPAT was significantly larger compared with that caused by 1 nmol/L 8-OH-DPAT (ANOVA, Bonferroni), as shown in Figure 2B. The amplitude of the glycinergic sIPSC was not significant changed by 8-OH-DPAT at 1 nmol/L, but was slightly although significantly decreased by 8-OH-DPAT at 10 nmol/L, 100 nmol/L, and 1 µmol/L (paired Student’s t-test), and the amplitude decrease (measured in percentage of controls) caused by 1 μmol/L and 100 nmol/L 8-OH-DPAT was significantly larger compared with that caused by 10 nmol/L 8-OH-DPAT (ANOVA, Bonferroni), as shown in Figure 2C,2D. These results indicated that both the frequency inhibition and the amplitude inhibition of the glycinergic sIPSC by 8-OH-DPAT had dose-dependent tendencies. The time-course of responses of the glycinergic sIPSC to 8-OH-DPAT was similar to the responses of the GABAergic synaptic currents to 8-OH-DPAT. At the end of each experiment, 1 μmol/L strychnine abolished the glycinergic sIPSC.
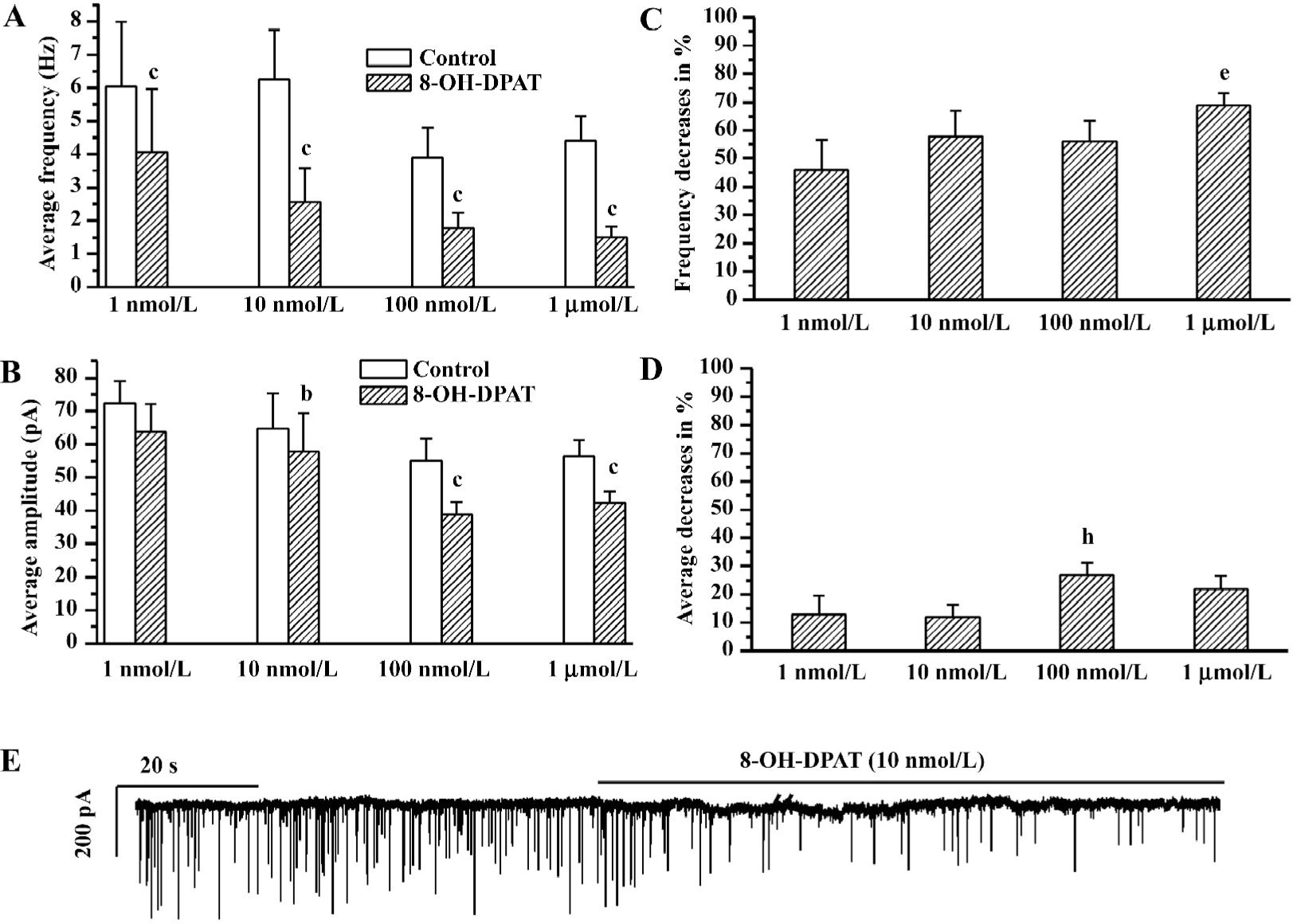
WAY-100635 blocked the inhibition of the GABAergic and glycinergic sIPSC by 8-OH-DPAT During the slow recovery process of the GABAergic or the glycinergic sIPSC from their responses to the 8-OH-DPAT applications, the addition of WAY-100635 (at the same doses as 8-OH-DPAT) abolished the responses within several seconds (tested in 3 CVPN of which the GABAergic sIPSC were recorded, and in 4 CVPN of which the glycinergic sIPSC were recorded). An experiment is shown in Figure 3A. In 15 CVPN pretreated with WAY-100635 (100 nmol/L in 8 CVPN and 1 µmol/L in 7 CVPN) the baseline frequency and the baseline amplitude of the GABAergic sIPSC were unaltered, and further application of 8-OH-DPAT at the same doses did not cause changes in the frequency and the amplitude. These 15 CVPN were analyzed as 1 group. The data from a representative experiment are shown in Figure 3B, 3C, and the data from the 15 experiments are summarized in Figure 3D,3E. In 9 CVPN pretreated with WAY-100635 (100 nmol/L in 5 CVPN and 1 µmol/L in 4 CVPN) the baseline frequency and the baseline amplitude of the glycinergic sIPSC were unaltered, and further application of 8-OH-DPAT at the same doses did not cause changes in the frequency and the amplitude. These 9 CVPN were also analyzed as 1 group. The data from a representative experiment are shown in Figure 3F, 3G, and the data from the 9 experiments are summarized in Figure 3H, 3I.
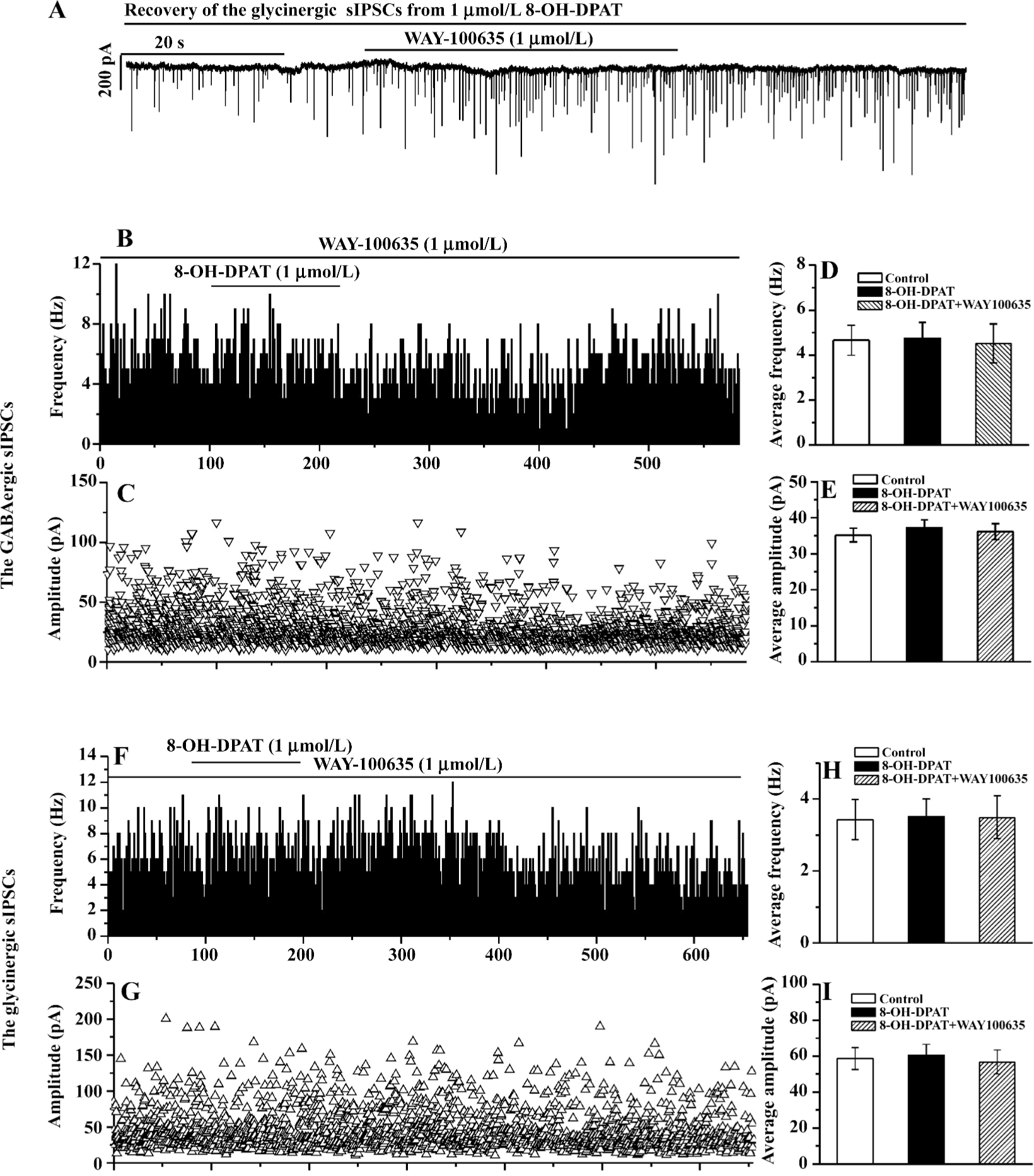
8-OH-DPAT had no effect on the GABAergic or the glycinergic mIPSC, and had no effect on the currents evoked by exogenous GABA or glycine The decreases in sIPSC frequency could be caused by the action of 8-OH-DPAT on the GABAergic or the glycinergic terminals, or preterminally on the dendrites, somas, or axons. The decreases in sIPSC amplitude could be caused by weakened summation of synaptic events or alterations in the activity of the GABAergic or the glycinergic neurons, such as changes in their action potential waveform or reduced recruitment of neurons. Another possibility is that the decreases in sIPSC amplitude are due to post-synaptic alterations in the responses to the released GABA or glycine. To distinguish between these possibilities, we examined the effect of 8-OH-DPAT on the GABAergic and the glycinergic mIPSC and examined the effect of 8-OH-DPAT on the currents evoked by exogenous GABA or glycine. The application of 1 µmol/L 8-OH-DPAT did not cause significant changes in the GABAergic or the glycinergic mIPSC in either the frequency or the amplitude (Figure 4). The GABAergic or the glycinergic currents evoked by exogenous GABA or glycine did not show any change upon application of 8-OH-DPAT (1 µmol/L), as shown in Figure 5.
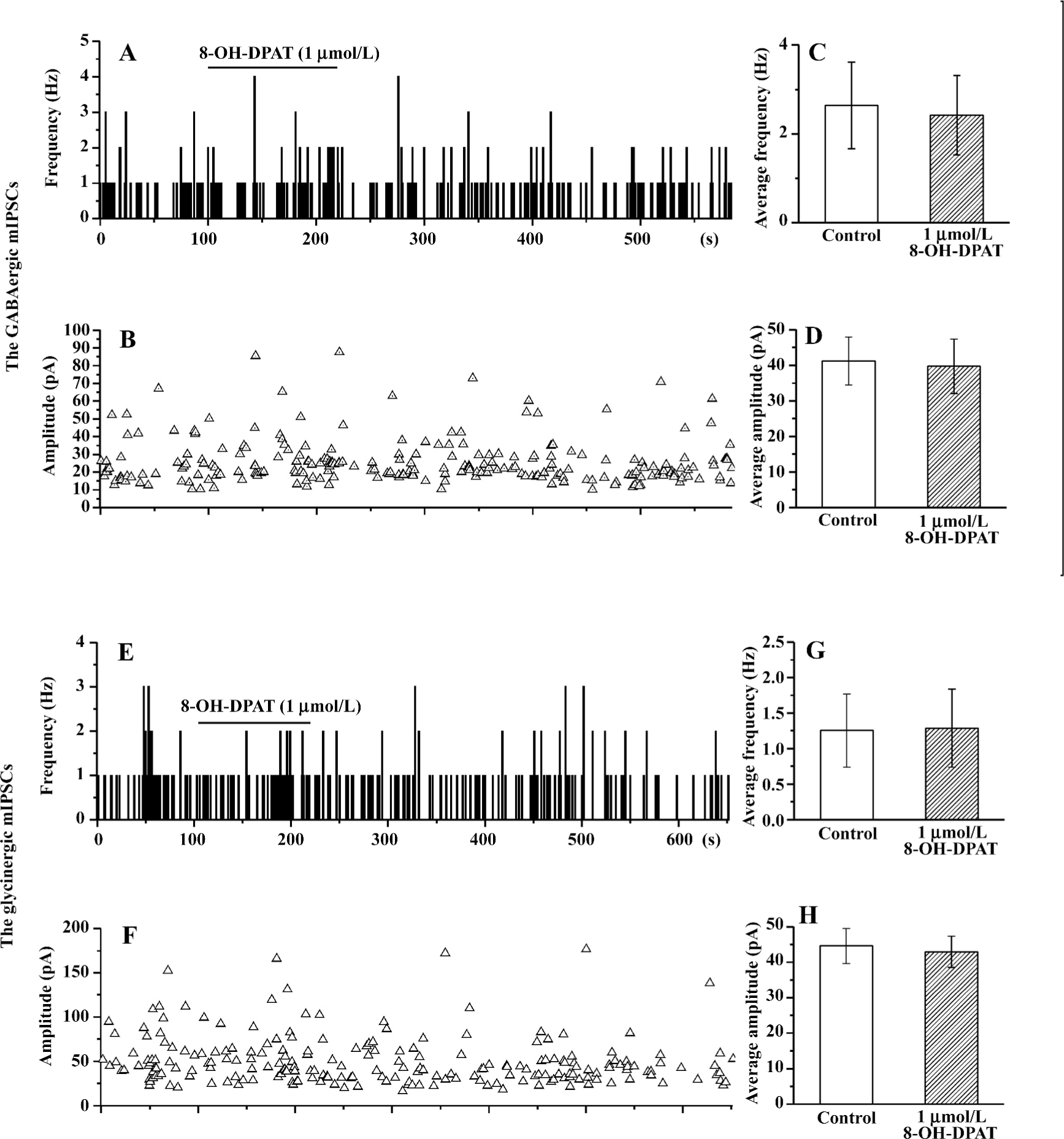
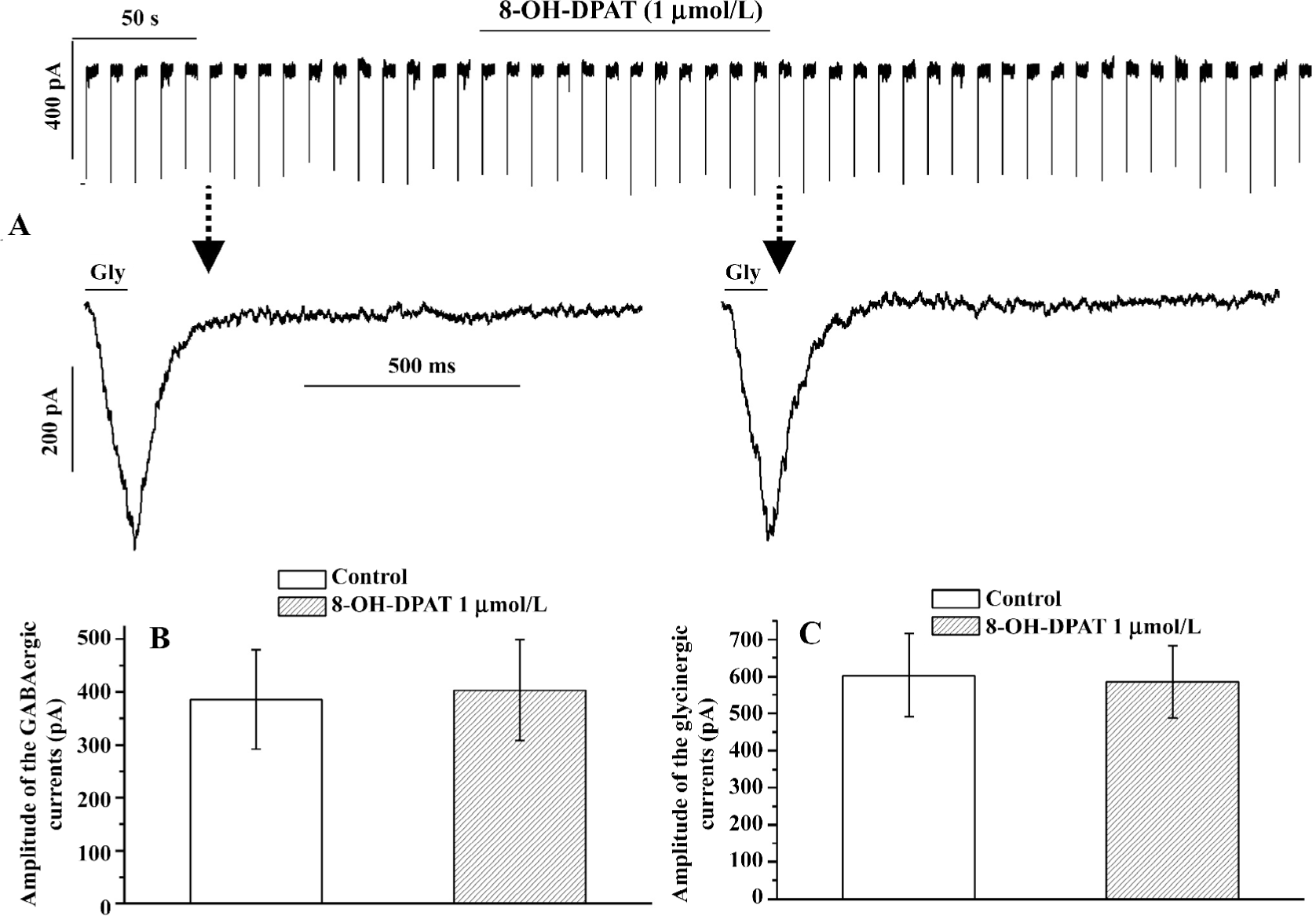
8-OH-DPAT had no effect on the glutamatergic sEPSC In the initial attempt to isolate the glutamatergic sEPSC, we added picrotoxin (10 µmol/L) and strychnine (10 µmol/L) in the perfusate. Unfortunately, this protocol evoked intermittent excitatory inward currents in CVPN mediated by NMDA and non-NMDA glutamatergic inputs, as we reported elsewhere[35]. We then omitted picrotoxin and strychnine in the recording of glutamatergic sEPSC. The application of 1 μmol/L 8-OH-DPAT did not cause any detectable change in the baseline currents and did not cause significant changes in either the frequency (1.15±0.40 vs 1.05±0.37 Hz; P>0.05, n=7; paired Student’s t-test) or the amplitude (11.19±1.23 vs 10.85±1.28 pA; P>0.05; n=7; paired Student’s t-test) of the glutamatergic sEPSC.
Discussion
There are 3 major findings in the present study. First, in CVPN the 5-HT1A/7 receptor agonist 8-OH-DPAT caused significant decreases in both the frequency and the amplitude of the GABAergic sIPSC, and also caused significant amplitude decrease of the GABAergic currents evoked by stimulation of the NTS, but had no effect on the GABAergic mIPSC and the GABAergic currents evoked by exogenous GABA. Both the frequency inhibition and the amplitude inhibition of the GABAergic sIPSC by 8-OH-DPAT had dose-dependent tendencies and could be reversed by the 5-HT1A/7 receptor antagonist WAY-100635. Second, in CVPN 8-OH-DPAT caused significant decreases in both the frequency and the amplitude of the glycinergic sIPSC, but had no effect on the glycinergic mIPSC and the glycinergic currents evoked by exogenous glycine. Both the frequency inhibition and the amplitude inhibition of the glycinergic sIPSC by 8-OH-DPAT had dose-dependent tendencies and could be reversed by the 5-HT1A/7 receptor antagonist WAY-100635. Third, 8-OH-DPAT had no effect on the glutamatergic sEPSC of CVPN.
The functional roles of the synaptic inputs identified in CVPN are poorly understood with regard to the reflex control of cardiac vagal tone. Both the present study and a recent study by others[29] demonstrated that 8-OH-DPAT inhibited the GABAergic sIPSC of CVPN. The present study demonstrated that 8-OH-DPAT also inhibited the GABAergic currents of CVPN evoked by stimulation of the NTS. These results strongly suggested that 5-HT1A/7 receptor-mediated inhibition of the GABAergic inputs are involved in the excitation of CVPN during cardiopulmonary reflex since the cardiopulmonary reflex control of CVPN is known to involve 5-HT1A/7 receptor activation in vivo[21]. In addition, the present study has proved that 8-OH-DPAT also inhibited the glycinergic inputs of CVPN, suggesting that the 5-HT1A/7 receptor-mediated inhibition of these inputs is also involved in the excitation of CVPN during cardiopulmonary reflex.
It is quite surprising that the present study, as well as the study of Wang and Ramage[21], does not indicate any role of the glutamatergic inputs to CVPN in the 5-HT1A/7 receptor-mediated reflex control of CVPN. One possibility is that the glutamatergic inputs of CVPN just account for their tonic control and on which the 5-HT1A/7 receptor-mediated inhibition of the GABAergic and the glycinergic inputs are superimposed and are responsible for their reflex control. The other possibility is that 5-HT might modulate the glutamatergic inputs of CVPN via other subtypes of 5-HT receptors. This second possibility is supported by a previous study that found 5-HT, an endogenous non-selective agonist of 5-HT receptors, caused either an increase or a decrease of the sEPSC of DMV preganglionic neurons controlling the gastrointestinal tract[36]. It has also been indicated by Wang et al[29] that other subtype of 5-HT receptors, such as 5-HT4α receptors, are involved in the control of the synaptic inputs of CVPN.
WAY-100635 had no effect on the baseline frequency and the baseline amplitude of the GABAergic or the glycinergic sIPSC of CVPN. These results suggested that the 5-hydroxytryptaminergic mechanism in the cardiopulmonary reflex pathway is not spontaneously active and its primary function is reflex related. This suggestion is also supported by the study of Wang and Ramage[21] that demonstrated that the ionophoretic application of WAY-100635 had no effect on the baseline firing of CVPN.
8-OH-DPAT caused significant frequency decreases of both the GABAergic and the glycinergic sIPSC of CVPN, but did not cause significant frequency changes of either the GABAergic or the glycinergic mIPSC. These results suggested that the modulation of the GABAergic and the glycinergic inputs by 8-OH-DPAT are action potential-dependent, and that 5-HT1A/7 receptors are located on the preterminal sites other than on the terminals. These results are consistent with the report that the 5-HT1A/7 agonist inhibits Na+ channels[26]. It is easy to imagine that in our experiments TTX, via the blockade of the Na+ channels on the GABAergic or the glycinergic neurons preceding CVPN, abolished the effect of 8-OH-DPAT. Of course it is also possible that the inhibition the GABAergic and the glycinergic sIPSC of CVPN by 8-OH-DPAT is just the consequence of polysynaptic actions.
8-OH-DPAT also caused significant amplitude decreases of both the GABAergic and the glycinergic sIPSC of CVPN. However, 8-OH-DPAT did not cause significant changes in the amplitude of the GABAergic or the glycinergic mIPSC, or in the amplitude of the GABAergic, or the glycinergic currents evoked by exogenous GABA or glycine. In addition, 8-OH-DPAT did not change the baseline currents and the input resistance of CVPN. These results suggested that the amplitude responses of the GABAergic and the glycinergic sIPSC to 8-OH-DPAT are presynaptic and are in part due to the decreased summation of sIPSC.
In summary, the present study demonstrated that the activation of 5-HT1A/7 receptors inhibited the GABAergic and the glycinergic inputs to CVPN. We conclude that the 5-HT1A/7 receptor-mediated reflex control of CVPN is via the inhibition of both the GABAergic and the glycinergic inputs to CVPN. Our findings have at least in part revealed the synaptic mechanisms involved in the 5-HT1A/7 receptor-mediated reflex control of cardiac vagal nerves in intact animals.
References
- Loewy AD, Spyer KM. Central regulation of autonomic function. NY: Oxford University Press; 1990.
- Mendelowitz D. Advances in parasympathetic control of heart rate and cardiac function. News Physiol Sci 1999;14:155-61.
- Nosaka S, Yasunaga K, Tamai S. Vagal cardiac preganglionic neurons: distribution, cell types, and reflex discharges. Am J Physiol 1982;243:R92-8.
- Stuesse SL. Origins of cardiac vagal preganglionic fibers: a retrograde transport study. Brain Res 1982;236:15-25.
- Standish A, Enquist LW, Escardo JA, Schwaber JS. Central neuronal circuit innervating the rat heart defined by transneuronal transport of pseudorabies virus. J Neurosci 1995;15:1998-2012.
- Mendelowitz D. Firing properties of identified parasympathetic cardiac neurons in nucleus ambiguus. Am J Physiol 1996;271:H2609-14.
- Steinbusch HWM. Distribution of serotonin immunoreactivity in the central nervous system of the rat – cell bodies and terminals. Neuroscience 1981;6:557-618.
- Sykes RM, Spyer KM, Izzo PN. Central distribution of substance P, calcitonin gene-related peptide, 5-hydroxytryptamine in vagal sensory afferents in the rat dorsal medulla. Neuroscience 1994;59:195-210.
- Izzo PN, Jordan D, Ramage AG. Anatomical and pharmacological evidence supporting the involvement of serotonin in the central control of cardiac vagal motoneurones in the anaesthetized cat. J Physiol (London) 1988;406:19.
- Izzo PN, Deuchars J, Spyer KM. Localization of cardiac vagal preganglionic motoneurones in the rat: Immunocytochemical evidence of synaptic inputs containing 5-hydroxytryptamine. J Comp Neurol 1993;327:572-83.
- Dashwood MR, Gilbey MP, Jordan D, Ramage AG. Autoradiographic localization of 5-HT1A binding sites in the brainstem of the cat. Br J Pharmacol 1988;94:386.
- Pazos A, Palacios JM. Quantitative autoradiographic mapping of serotonin receptors in the rat brain. I. Serotonin-1 receptors. Brain Res 1985;346:205-30.
- Thor KB, Blitz-siebert A, Helke CJ. Autoradiographic localization of 5HT1 binding sites in autonomic areas of the rat dorsomedial medulla oblongata. Synapse 1992;10:217-27.
- Pazos A, Probst A, Palacios JM. Serotonin receptors in the human brain. III. Autoradiographic mapping of serotonin-1 receptors. Neuroscience 1987;21:97-122.
- Ramage AG, Fozard JR. Evidence that the putative 5-HT1A receptor agonists, 8-OH-DPAT, ipsapirone, have a central hypotensive action that differs from that of clonidine in anaesthetised cats. Eur J Pharmacol 1987;138:179-91.
- McCall RB, Escandon NA, Harris LT, Clement ME. Tolerance development to the vagal-mediated bradycardia produced by 5-HT1A receptor agonists. J Pharmacol Exp Ther 1994;271:777-81.
- McCall RB, Escandon NA, Harris LT, Clement ME, Shepheard SL, Jordan D, et al. Comparison of the effects of IVth ventricular administration of some tryptamine analogues with those of 8-OH-DPAT on autonomic outflow in the anaesthetized cat. Br J Pharmacol 1994;111:616-24.
- Shepheard SL, Jordan D, Ramage AG. Comparison of the effects of IVth ventricular administration of some tryptamine analogues with those of 8-OH-DPAT on autonomic outflow in the anaesthetized cat. Br J Pharmacol 1994;111:616-24.
- Sporton SCE, Shepheard SL, Jordan D, Ramage AG. Microinjections of 5-HT1A agonists into the dorsal motor vagal nucleus produce a bradycardia in the atenolol-pretreated anaesthetized rat. Br J Pharmacol 1991;104:467-70.
- Chitravanshi VC, Calaresu FR. Additive effects of dopamine and 8-OH-DPAT microinjected into the nucleus ambiguus in eliciting vagal bradycardia in rats. J Auto Nerv Syst 1992;41:121-8.
- Wang Y, Ramage AG. The role of central 5-HT (1A) receptors in the control of B-fibre cardiac and bronchoconstrictor vagal preganglionic neurones in anaesthetized cats. J Physiol (London) 2001;536:753-67.
- Bogle RG, Pires JGP, Ramage AG. Evidence that central 5-HT1A receptors play a role in the von Bezold-Jarisch reflex in the rat. Br J Pharmacol 1990;100:757-60.
- Skinner MR, Ramage AG, Jordan D. Effect of antagonism of central 5-HT1A receptors on baroreceptor and cardiopulmonary receptor reflexes in anaesthetized rabbits. J Physiol (London) 1998;513P:85.
- Dando SB, Skinner MR, Jordan D, Ramage AG. Modulation of the vagal bradycardia evoked by stimulation of upper airway receptors by central 5-HT1 receptors in anaesthetized rabbits. Br J Pharmacol 1998;125:409-17.
- Colino A, Halliwell JV. Differential modulation of three separate K-conductances in hippocampal CA1 neurons by serotonin. Nature 1987;328:73-7.
- Melena J, Chidlow G, Osborne NN. Blockade of voltage-sensitive Na(+) channels by the 5-HT(1A) receptor agonist 8-OH-DPAT: possible significance for neuroprotection. Eur J Pharmacol 2000;406:319-24.
- Wang J, Wang X, Irnaten M, Venkatesan P, Evans C, Baxi S, et al. Endogenous acetylcholine and nicotine activation enhances GABAergic and glycinergic inputs to cardiac vagal neurons. J Neurophysiol 2003;89:2473-81.
- Wang J, Irnaten M, Mendelowitz D. Characteristics of spontaneous and evoked GABAergic synaptic currents in cardiac vagal neurons in rats. Brain Res 2001;889:78-83.
- Wang X, Dergacheva O, Kamendi H, Gorini C, Mendelowitz D. 5-Hydroxytryptamine 1A/7 and 4α receptors differentially prevent opioid-induced inhibition of brain stem cardiorespiratory function. Hypertension 2007. [Crossref]
- Gatti PJ, Johnson TA, Phan P, Jordan IK, Coleman W, Massari VJ. The physiological and anatomical demonstration of functionally selective parasympathetic ganglia located in discrete fat pads on the feline myocardium. J Auton Nerv Syst 1995;51:255-99.
- Grkovic I, Fernandez K, McAllen RM, Anderson CR. Misidentification of cardiac vagal pre-ganglionic neurons after injections of retrograde tracer into the pericardial space in the rat. Cell Tissue Res 2005;321:335-40.
- Irnaten M, Neff RA, Wang J, Loewy AD, Mettenleiter TC, Mendelowitz D. Activity of cardiorespiratory networks revealed by transsynaptic virus expressing GFP. J Neurophysiol 2001;85:435-8.
- Neff RA, Wang J, Baxi S, Evans C, Mendelowitz D. Respiratory sinus arrhythmia: endogenous activation of nicotinic receptors mediates respiratory modulation of brainstem cardioinhibitory parasympathetic neurons. Circ Res 2003;93:565-72.
- Bobker DH, Williams JT. Serotonin-mediated inhibitory postsynaptic potential in guinea-pig prepositus hypoglossi and feedback inhibition by serotonin. J Physiol 1990;422:447-62.
- Wang J, Chen Y, Li K, Hou L. Blockade of inhibitory neurotransmission evoked seizure-like firing of cardiac parasympathetic neurons in brainstem slices of newborn rats: implications for sudden deaths in patients of epilepsy. Epilepsy Res 2006;70:172-83.
- Browning KN, Travagli RA. Characterization of the in vitro effects of 5-hydroxytryptamine (5-HT) on identified neurons of the rat dorsal motor nucleus of the vagus (DMV). Br J Pharmacol 1999;128:1307-15.