Bufalin induces apoptosis in human osteosarcoma U-2OS and U-2OS methotrexate300-resistant cell lines1
Introduction
Osteosarcoma is a common primary malignant tumor of bones in children and adolescents, characterized by an extremely aggressive clinical course with rapid development of metastases[1–3]. The first choice of treatment for osteosarcoma is neoadjuvant chemotherapy. Many anticancer drugs, such as methotrexate (MTX), doxorubicin, cisplatin, etoposide, and cyclophosphamide are commonly used, either alone or in combination[4,5]. Although the adoption of adjuvant and neoadjuvant chemotherapy has significantly improved the prognosis, a considerable number of osteosarcoma patients develop drug resistance and die as a result of disease progression[1,4,5].
MTX, one of the most important drugs in osteosarcoma treatment, is a potent inhibitor of the dihydrofolate reductase (DHFR) enzyme. DHFR catalyzes the reduction of folate and 7,8-dihydrofolate to 5,6,7,8-tetrahydrofolate.
Resistance to MTX can arise through several mechanisms[6–8]. In osteosarcomas, these mechanisms include increased levels of the DHFR enzyme because of DHFR gene overexpression, and impaired intracellular transport of MTX, as a consequence of decreased levels of the reduced folate carrier in the cell membrane[9]. Enhanced levels of DHFR are frequently present in proliferating tumor cells, which can be associated with the amplification of the DHFR gene. Thus, new treatments that circumvent the mechanisms responsible for drug resistance could become the basis for innovative therapeutic regimens aimed at increasing the drug response rate and improving the clinical outcome of patients[10,11].
Bufalin is a major digoxin-like immunoreactive component of “Chan’Su”, a traditional Chinese medicine obtained from the skin and parotid venom glands of toads[12–14]. Bufalin has been reported to exhibit significant antitumor activity against human leukemia cells[15–17], endometriotic stromal cells[18], and prostate cancer cells[19]. Its activities are mediated by the induction of apoptosis and cell differentiation; the regulation of various genes and proteins is also involved in the process.
Although the antitumor activities of bufalin have been analyzed in several tumors, no data have been reported so far about its effectiveness on human osteosarcoma cells. Thus, we evaluated the effects of bufalin on the proliferation, cell cycle arrest, and apoptosis in human osteosarcoma U-2OS and U-2OS/MTX300 cell lines. Moreover, we assessed the effect of bufalin on the DHFR expression. The aim of this study was to determine the effects of this agent on human osteosarcoma cell lines in vitro to assess whether it might be useful for alternative chemotherapeutic regimens in osteosarcoma patients unresponsive (resistant) to MTX.
Materials and methods
Drugs and reagents Bufalin and MTX were purchased from Sigma Chemical Co (St Louis, MO, USA) Doxorubicin, cisplatin, and paclitaxel were purchased from the National Institute for the Control of Pharmaceutical and Biological Products (Beijing, China). Stock solutions of these drugs were stored at 4 °C. Working concentrations were then prepared by diluting stock solutions in culture medium immediately before use.
MTT (methyl thiazolyl tetrazolium) and Hoechst 33258 were purchased from Sigma Chemical Co (USA) The DNAzol and TRIzol reagents were purchased from Invitrogen Life Technologies (Paisley, Scotland, UK) and Gibco BRL (Grand Island, NY, USA), respectively. Reagents for RT-PCR were from Toyobo (Osaka, Japan). Antibodies to DHFR were purchased from Becton-Dickinson (Mountain View, CA, USA). Antibodies to Bax, Bcl-2, p53, p21, and β-actin were obtained from Santa Cruz Biotechnology (Santa Cruz, CA, USA).
Cell lines and culture The human osteosarcoma cell lines U-2OS and U-2OS/MTX300 were gifts from Dr M SERRA (Istituti Ortopedici Rizzoli, Bologna, Italy). Starting with the MTX-sensitive U-2OS osteosarcoma cell line, the MTX300-resistant variant was obtained by exposing the parental line in vitro to stepwise increased MTX concentrations[20]. Such continuous exposure to MTX resulted in cells resistant to 300 µg/L (U-2OS/MTX300). The MTX300-resistant variant was continuously cultured in the presence of 300 µg/L MTX. All of the cells were cultured in Iscove’s modified Dulbecco’s medium (IMDM, Gibco BRL, USA), supplemented with 10% fetal calf serum (Hyclone, Logan, UT, USA), penicillin (10 000 U/L), and streptomycin (100 mg/L) at 37 °C in a 5% CO2 humidified atmosphere.
MTT assay The effects of bufalin on cell proliferation and the viability of U-2OS and U-2OS/MTX300 cells were assessed using the MTT assay. U-2OS and U-2OS/MTX300 cells were harvested and seeded in 96-well plates at 6.0×103 cells/well in a final volume of 180 µL. After 24 h of incubation, drugs were added to duplicate plates at appropriate concentrations. After 92 h, MTT solution (10 µL) was added to each well. DMSO (100 µL) was added to each well 4 h later. The concentrations required to inhibit cell growth by 50% (IC50) were calculated from the cytotoxicity curves (Bliss’s software; Bliss Co, CA, USA). The degree of resistance was calculated by dividing the IC50 for the U-2OS/MTX300 cells by that of the parental MTX-sensitive cells. The IC50 values were calculated from at least 3 independent experiments.
Fluorescent staining The cells treated with the indicated concentrations of bufalin for 48 h were collected by centrifugation (1500×g, 5 min), washed twice with phosphate buffered saline (PBS), and stained with the DNA-specific dye Hoechst 33258 (10 mL, 10 mg/L). The cells were then incubated at 37 °C for 10 min and again washed in PBS for morphological observation using an Olympus photomicroscope with an epifluorescence attachment (Tokyo, Japan). Cells displaying condensed, fragmented DNA were deemed apoptotic.
DNA fragmentation assay The integrity of the cells’ genomic DNA was assessed by agarose gel electrophoresis. The cells (1×106) were added to DNAZOL (genomic DNA isolation) reagent (0.5 mL) and lysed by agitation. The lysate was gently pipetted into an assay tube, and the homogenate was cleared by centrifugation (10 000×g, 10 min). Following centrifugation, the resulting viscous supernatant was transferred to a fresh tube, and DNA from the lysate was precipitated by the addition of 100% ethanol (0.25 mL). The precipitate was washed twice with 75% ethanol (0.8–1.0 mL). After removing the ethanol, the DNA precipitate was dissolved in NaOH solution (8 mmol/L). The DNA samples were loaded on 2% agarose gels for electrophoresis. The gels were stained with ethidium bromide (0.5 mg/L) and photographed under UV illumination.
Western blot analyses The cells were washed in cold PBS, then lysed and harvested by scraping with a protein extraction reagent (Pierce Biotechnology, Rockford, USA). Protein concentrations were determined using the Bradford assay. For each sample, the protein extract (30 µg) was separated on 15% SDS-PAGE and electroblotted onto polyvinylidene difluoride membranes. The membranes were incubated with primary antibodies for 12 h at 4 °C followed by 3 washes in Tris-buffered saline with Tween-20 (TBST) for 5 min, and were then incubated with horseradish peroxidase-conjugated secondary antibody for 1 h at room temperature. Western blot analyses were performed using anti-DHFR (Becton-Dickinson, USA), antihuman Bax, antihuman p53, antihuman p21, and antihuman Bcl-2 (Santa Cruz Biotechnology, USA) monoclonal antibodies. Protein bands were visualized on X-ray film using an enhanced chemiluminescence detection system. For each sample, the amount of protein was determined by densitometric analysis.
RT-PCR analysis The total RNA was extracted by using TRIzol reagent, according to the manufacturer’s protocol. The total RNA (1 μg) was denatured at 65 °C for 10 min and then reverse transcribed at 42 °C for 40 min in a reaction mix (20 µL) containing 5×RT buffer, 10 mmol/L deoxyribonucleotide triphosphate (dNTP) mix, 10 U/µL RNase inhibitor, and 10 µmol/L oligo (dT; ReverTra Ace, Toyobo, Japan). Part (2 µL) of each RT reaction was amplified using ReverTra Dash (Toyobo, Japan) in a total volume of 100 µL. Transcripts of the gene for glyceraldehydes-3-phosphate dehydrogenase (G3PDH) were used as internal controls.
After incubating the mix at 94 °C for 2 min, 30 PCR cycles were conducted, consisting of 94 °C for 1 min, annealing at 60 °C for 1 min, and elongation at 72 °C for 1 min with a 5 min extension at 72 °C following the last cycle. For DHFR, the primers were 5'-GTAGAAGGTAAACAGAATCTG-3' (forward intron-spanning) and 5'-AGAACACCTGGG-TATTCTGG-3' (reverse intron-spanning), and for G3PDH, the primers were 5'-ACCACAGTCCATGCCATCAC-3' (forward) and 5'-TCCA-CCACCCTGTTGCTGTA-3' (reverse). The amplified samples were then electrophoresed on 1.5% agarose gels containing ethidium bromide (0.5 mg/L).
Statistical analysis All data were derived from at least 3 independent experiments and results are expressed as mean±standard error. Differences were assessed using the Student’s t-test or the Kruskal-Wallis test. A P value of <0.05 was deemed statistically significant.
Results
Effects of bufalin on the viability of the U-2OS and U-2OS/MTX300 cell lines The effects of bufalin and other drugs on the viability of U-2OS and U-2OS/MTX300 cells were assessed using the MTT assay (Table 1). To investigate the impact of MTX resistance, we first made a comparison between U-2OS and U-2OS/MTX300 cells. U-2OS/MTX300 were approximately 120-fold more resistant to MTX than U-2OS. Bufalin showed a potent cytotoxic effect on both U2OS and U-2OS/MTX300 cells. The IC50 values of bufalin for U-2OS and U-2OS/MTX300 cells were 8.49±2.1 µg/L and 10.19±1.7 µg/L, respectively; these values were not statistically significantly different (P>0.05). The IC50 value of bufalin was similar to that of doxorubicin, although significantly lower than that of cisplatin or paclitaxel. U-2OS/MTX-300 cells showed no cross-resistance with bufalin. Similar results were obtained with doxorubicin, cisplatin, and paclitaxel.
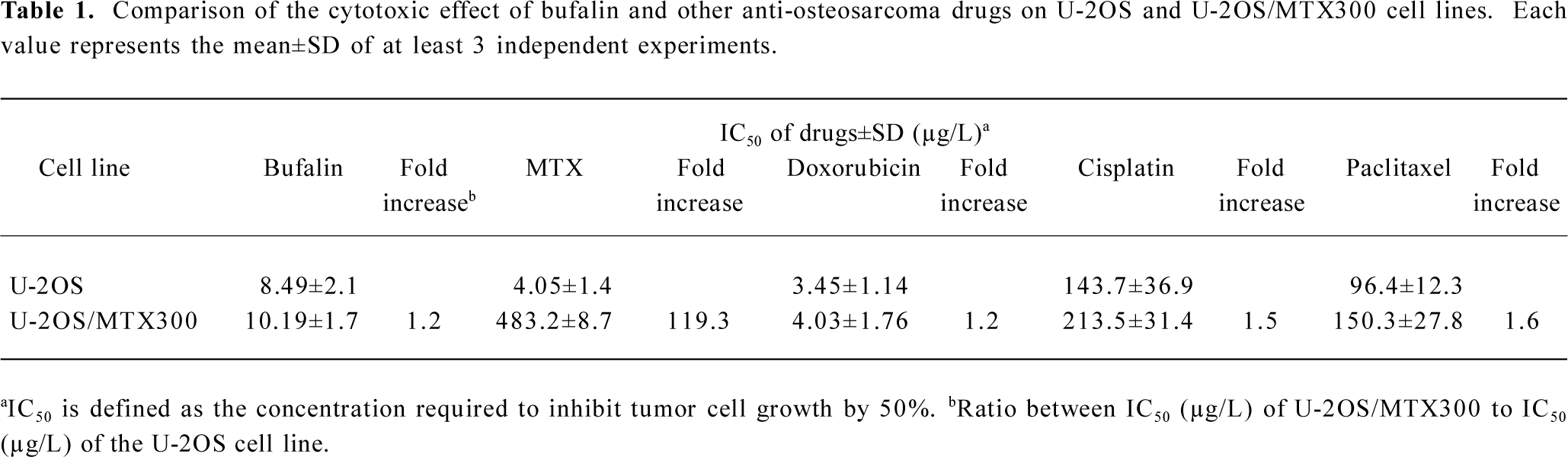
Full table
The loss of viability could be the result of inducing apoptosis, blocking the cell cycle at a specific point, such as G2/M, or more generally, inhibiting growth. Thus, we next investigated whether bufalin could induce apoptosis or block the cell cycle in human osteosarcoma cells.
Effects of bufalin on apoptosis in the U-2OS and U-2OS/MTX300 cell lines To examine whether bufalin could induce apoptosis in human osteosarcoma U2OS cells and the MTX-resistant variant, we first used fluorescent staining with Hoechst 33258 (Figure 1). U-2OS and U-2OS/MTX300 cells were treated with bufalin (0, 10, 50, or 100 µg/L) for 48 h. Fluorescent observation shown that bufalin could induce apoptosis in both cell lines. The cells showed typical apoptotic morphology characterized by volume reduction, chromatin condensation, nuclear fragmentation, and the appearance of apoptotic bodies.
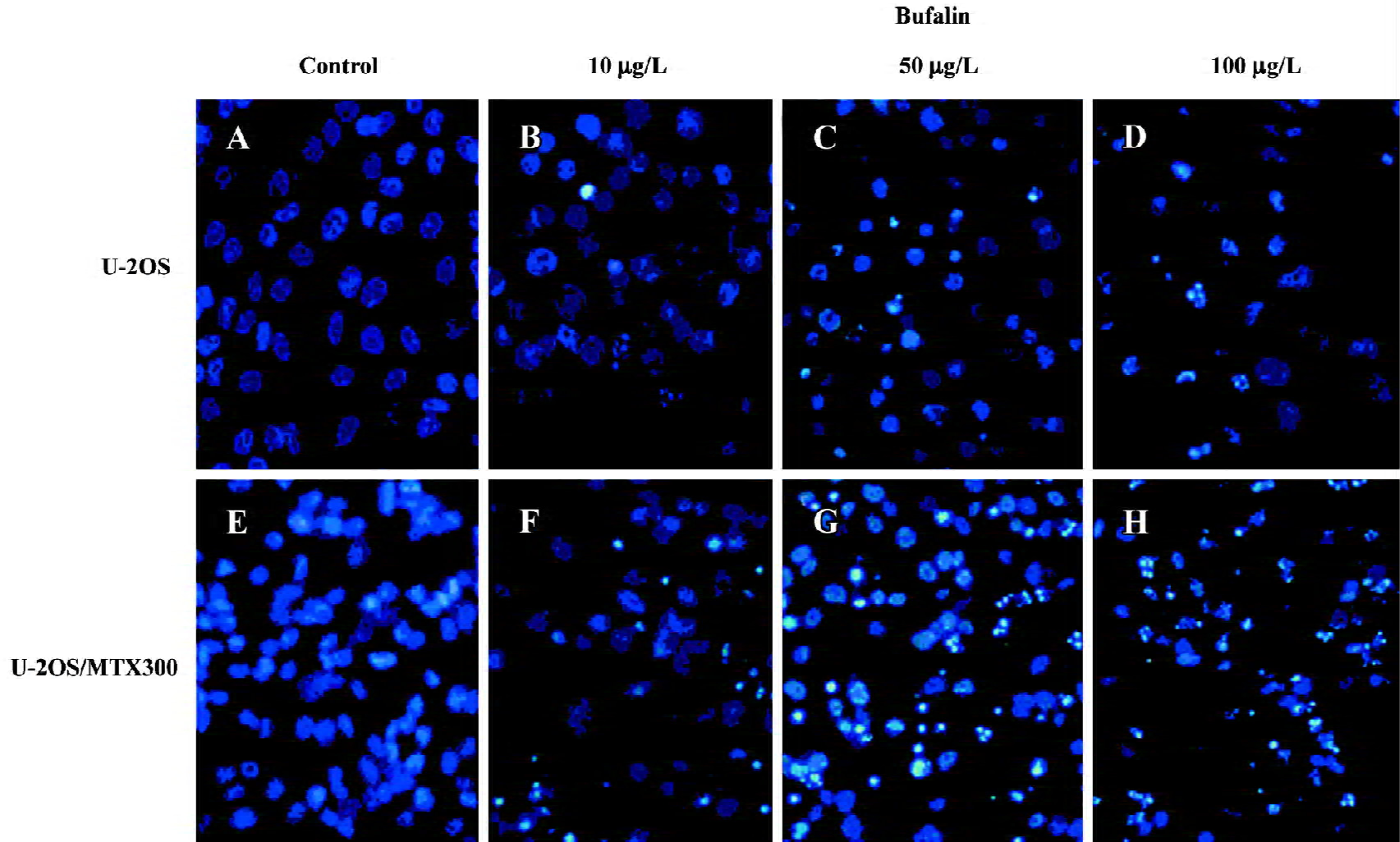
Next, we used flow cytometry to assess the degree of bufalin-induced apoptosis in U2OS cells and in U-2OS/MTX300 cells. After incubation for 48 h, bufalin induced apoptosis in U-2OS and U-2OS/MTX300 cells in a dose-dependent manner (Figure 2). The sub-G1 peak proportion was assessed and the difference was significant between the bufalin-treated (10, 50, and 100 µg/L) and the control cells.
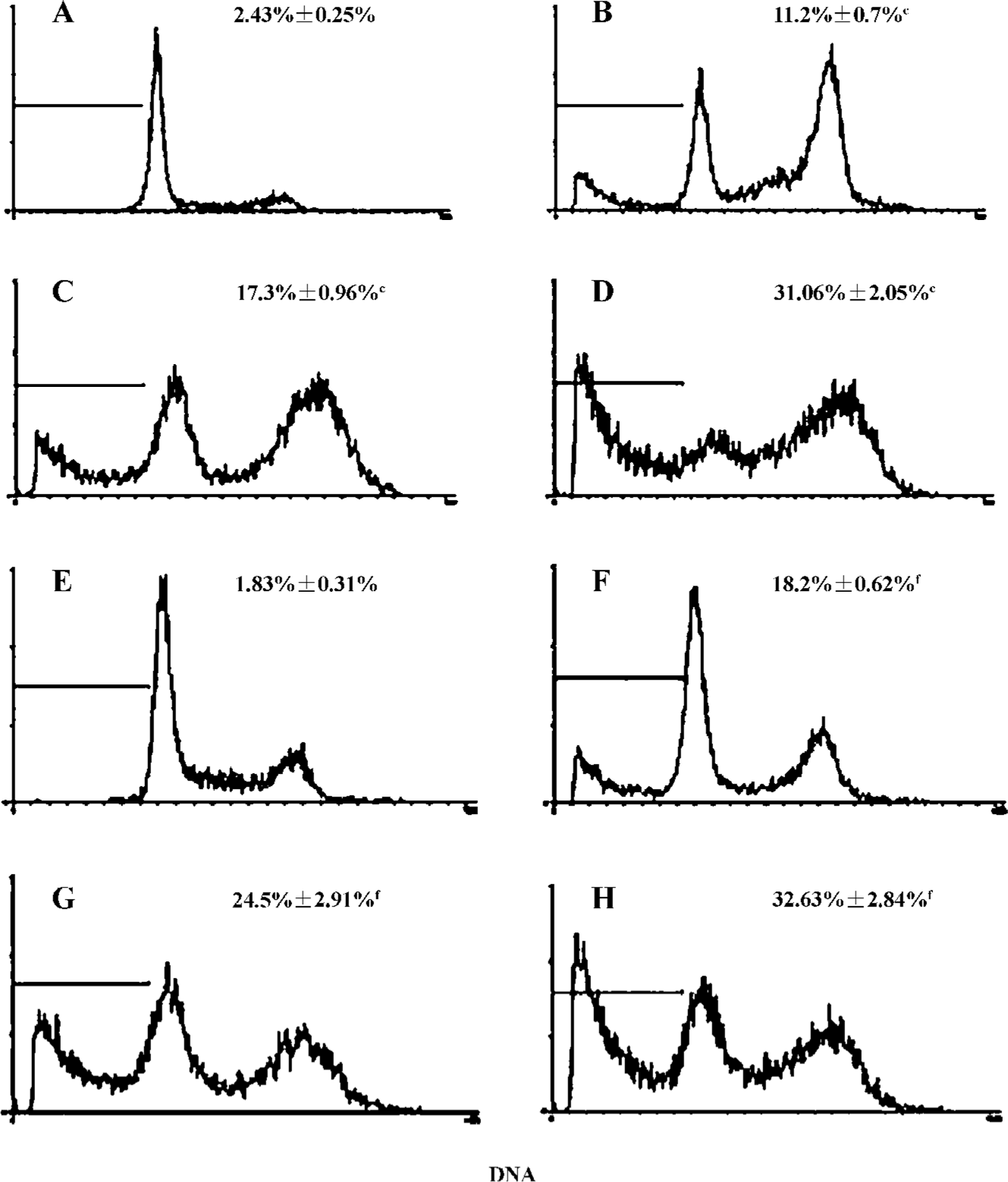
To further assess the apoptotic effect of bufalin, we examined genomic DNA fragmentation using agarose gel electrophoresis and DNA staining. After incubating the cells with bufalin (0, 10, 50, and 100 µg/L) for 48 h, the characteristic “ladder” of the fragmented DNA was evident (Figure 3).
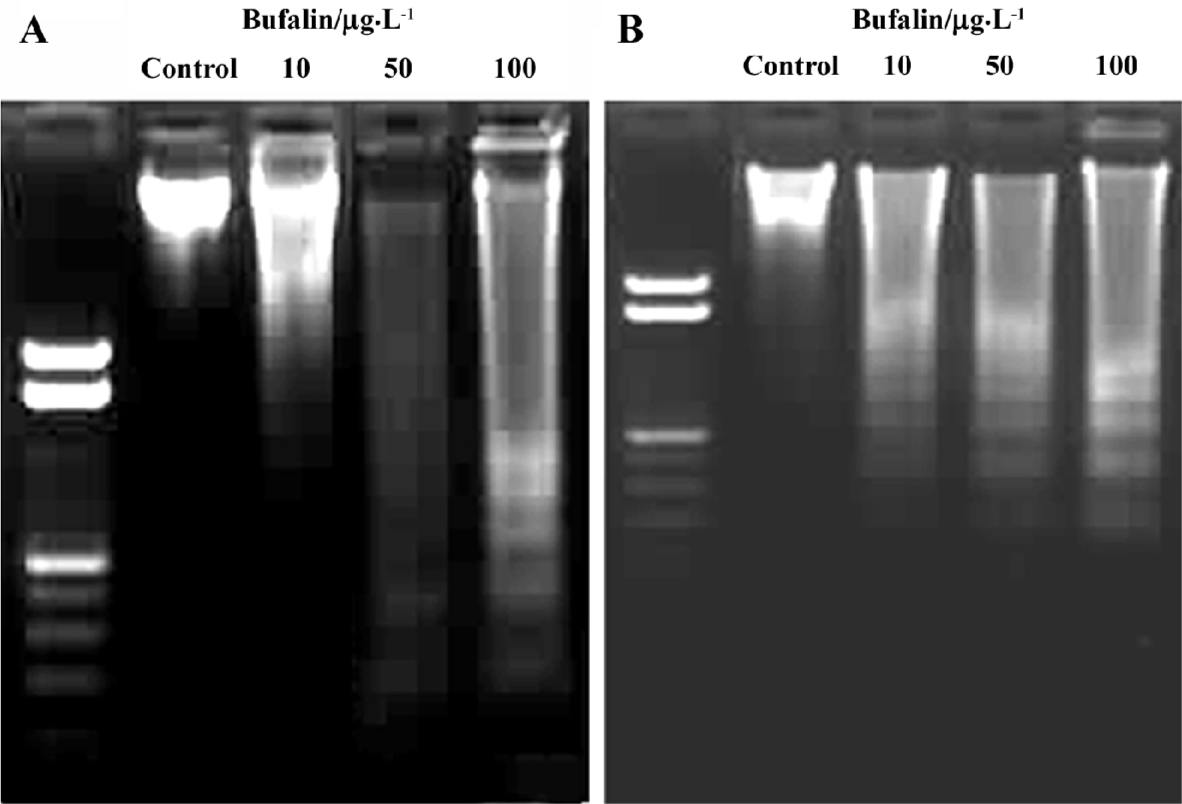
Effects of bufalin on the cell cycle in the U-2OS and U-2OS/MTX300 cell lines The effects of bufalin on the cell cycle in U-2OS and U-2OS/MTX300 cells were examined by flow cytometry. After culture for 48 h in the presence of bufalin (0, 10, 50, and 100 µg/L), accumulation of cells in the G2/M phase was observed in both U-2OS and U-2OS/MTX300 cells in a dose-dependent manner (Figure 4). A concomitant decrease in the proportion of cells in the G0/G1 phase was also seen in U-2OS cells, while no such decrease was obvious in U-2OS/MTX300 cells. Similar results were obtained in repeated experiments, suggesting that bufalin leads to the accumulation of cells in the G2/M phase in U-2OS and U-2OS/MTX300 cells.
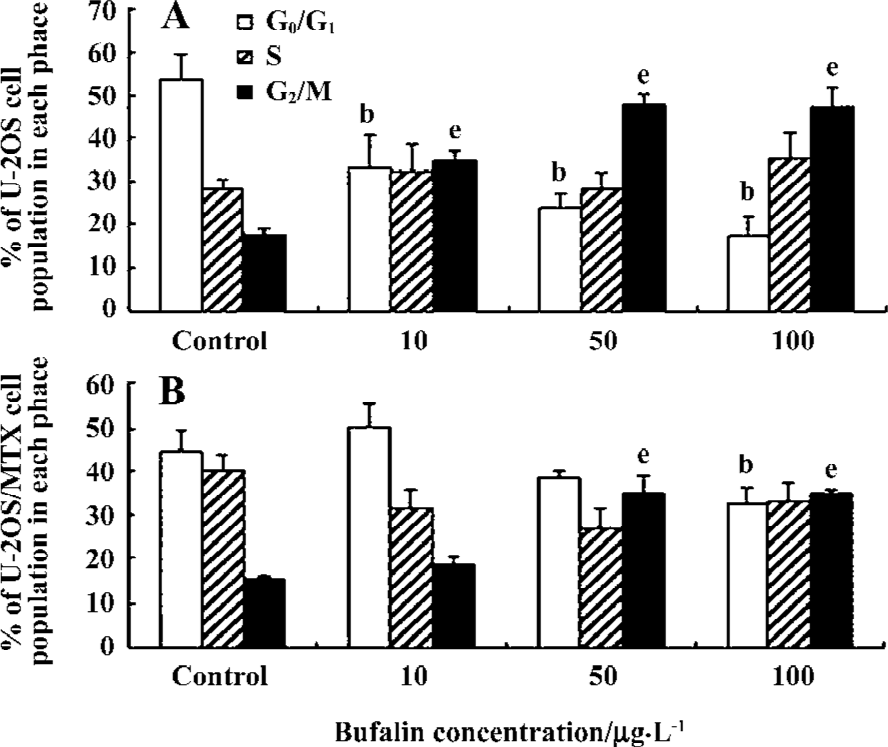
Effects of bufalin on the expression of apoptosis-related proteins in the U-2OS and U-2OS/MTX300 cell lines Based on the apoptotic analysis, we next examined the expression of apoptosis-related proteins. We confirmed intracellular apoptotic events biochemically by examining the expression levels of p53, p21, Bcl-2, and Bax. Bufalin had obvious effects on p53, Bcl-2, and Bax in both cell lines (Figure 5). The expression of Bcl-2 was downregulated in bufalin-treated cells, while p53, p21, and Bax were upregulated with an increase in the bufalin concentration from 10 to 100 µg/L. The ratio of Bax/Bcl-2 increased dose dependently after bufalin treatment. These results suggest that bufalin can induce apoptosis in U-2OS and U-2OS/MTX300 cells by affecting these apoptosis-related proteins. With regard to the expression levels of p21, there was some difference between U-2OS and U-2OS/MTX300 cells (Figure 5). In U-2OS/MTX300 cells, the induction of p21 was evident; however, in U-2OS cells, there was no such difference between the bufalin-treated and control cells.
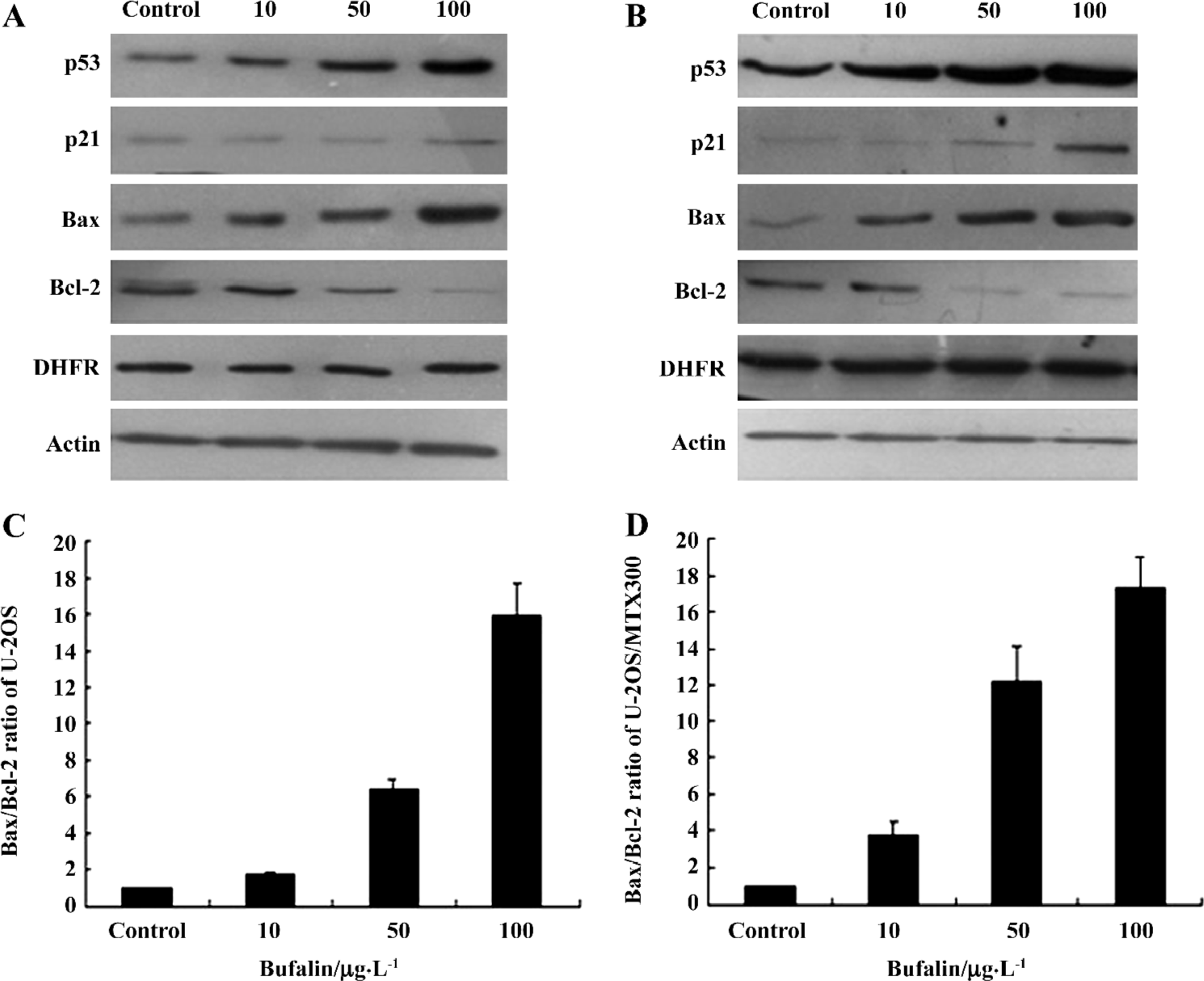
Effects of bufalin on the gene expression and protein levels of DHFR in the U-2OS and U-2OS/MTX300 cell lines To assess whether bufalin could affect DHFR expression, RT-PCR and Western blot analysis were conducted. After treatment with bufalin (0, 10, 50, and 100 µg/L) for 48 h, there was no obvious difference in DHFR at either the mRNA or protein level (Figures 5, 6), suggesting that bufalin does not affect DHFR expression in U-2OS and U-2OS/MTX300 cells.
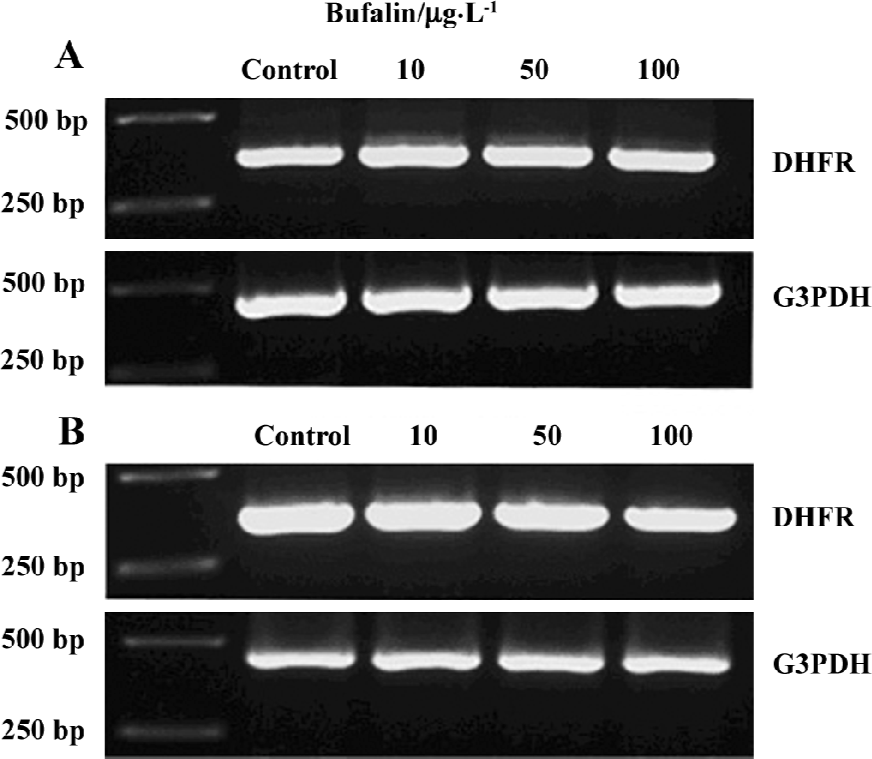
Discussion
Despite significant advances in the treatment of osteosarcoma, the development of drug resistance is a common clinical problem that significantly decreases the effectiveness of chemotherapy in high-grade osteosarcoma patients. Responsiveness to chemotherapy is a critical factor that dramatically influences clinical outcome[1,3–5]. A strategy that has been used is the search for new anticancer agents in traditional medicine[21].
Bufalin is a major component of Chan Su, a traditional Chinese medicine obtained from the skin and parotid venom glands of toads. Bufalin has been shown to exhibit antitumor activity by inhibiting the growth of and inducing apoptosis in several tumor cells. The mechanism of bufalin-induced apoptosis has been examined and includes the activation of AP-1[16], Rac1[17], cdc2 kinase and casein kinase II[22], the induction of Tiam1 expression[17], and the elevation of intracellular calcium concentrations[19]. Apoptosis-related proteins have also been examined; bufalin downregulates the expression of cyclin A, Bcl-2, and Bcl-X(L) and upregu-lates the expression of p21 and Bax[15,18]. Jing et al reported that apoptosis was not induced by bufalin in normal mononuclear or polymorphonuclear cells[23]. Nasu et al also indicated that bufalin induced apoptosis in endometriotic cyst stromal cells; in contrast, only marginal effects were observed in normal endometrial stromal cells[18]. The induction of cell cycle arrest by bufalin also differed among cells[18,23,24]; thus, the effects of bufalin may be cell-type specific.
To date, no data have been reported regarding the activity of bufalin in osteosarcoma cells. To gain insight into the effects of bufalin, we investigated its cytotoxicity and efficacy in the human U-2OS cell line and its variant U-2OS/MTX300. Our cell proliferation assays showed that bufalin strongly inhibited the proliferation of U-2OS and U-2OS/MTX300 in a dose-dependent manner. Based on these results, we examined the morphological changes in the cells by Hoechst 33258 staining (Figure 1). Flow cytometry analysis was also used to examine the apoptosis-inducing effects of bufalin (Figure 2). We also performed a DNA fragmentation assay to examine a late marker of apoptosis. After the U2OS cells were treated with bufalin at different concentrations for 48 h, significant DNA fragmentation was detected (Figure 3). In the cell cycle analysis, a significant increase in the cell population in the G2/M phase was observed after 48 h treatment with bufalin (Figure 4), which was consistent with previous studies[22,24].
To further examine the biological mechanism of bufalin-induced apoptosis, we assessed the levels of the p53, p21, Bcl-2, and Bax proteins by Western blot analysis. It is known that the tumor suppressor protein p53 induces apoptosis through several pathways and one of these involves pro-apoptotic and anti-apoptotic proteins of the Bcl-2 family. Members of the Bcl-2 family elicit opposing effects on mitochondria. Bax is a target of p53 and is a member of the Bcl-2 family that regulates apoptosis and cell prolifera-tion[25,26]. Translocation of Bax to the mitochondria in response to cell damage induces the mitochondrial permeability transition, an event that releases cytochrome c and culminates in the death of the cells[27]. Bcl-2 is transcriptionally suppressed by p53, helping to preserve the integrity of the mitochondria[28]. p21, a potent inhibitor of cyclin-dependent kinases, is also a downstream effector of the p53 pathway of growth control[29].
We observed that bufalin increased the expression of p53 and Bax, while it reduced the expression of Bcl-2 in both U-2OS and U-2OS/MTX300 cells. Thus, the induction of apoptosis in human osteosarcoma cells by bufalin was associated with the p53 pathway and Bcl-2 family proteins. Previous studies showed that the ratio of Bax to Bcl-2 (rather than anti-apoptotic Bcl-2 alone) is important in cell survival or apoptotic death in response to death stimuli[30]. In our study, the average ratio of Bax/Bcl-2 in p53-containing U2OS cells treated with bufalin was significantly higher than in the control cells. These results further suggest that the mechanism of bufalin-induced apoptosis in U2OS cells includes the modulation of the ratio of Bax/Bcl-2 by p53.
In this study, we found that bufalin leads to the accumulation of cells in the G2/M phase in both U-2OS and U-2OS/MTX300 cells. A concomitant decrease in the proportion of cells in the G0/G1 phase was also seen in U-2OS cells, while no such decrease was obvious in U-2OS/MTX300 cells. The extent of p21 induction was also different between U-2OS and U-2OS/MTX300 cells; it was almost absent in the U-2OS cell line. There are some differences between U-2OS and U-2OS/MTX300 cells; specifically, U-2OS/MTX300 cells showed a higher doubling time, a lower cloning efficiency, and a lower metastatic ability after iv injection than the corresponding parental cell line[31]. Thus, the differences in the cell cycle and p21 induction between U-2OS and U-2OS/MTX300 after treatment with bufalin may be due to these different characteristics and should be studied further.
In our study, we were particularly interested in the findings that bufalin retained its efficacy in MTX-resistant cells, which have an increased level of DHFR[10,11]. Studies have shown that an increase in DHFR levels is an important mechanism responsible for the degree of clinical resistance to MTX in osteosarcoma patients[9,10]. Thus, we sought to evaluate DHFR gene expression and protein levels in bufalin-treated U-2OS and U-2OS/MTX300 human osteosarcoma cells. After treatment with bufalin for 48 h, there was no difference in DHFR at the mRNA or protein level (Figures 5, 6), suggesting that bufalin does not affect DHFR expression in U-2OS or U-2OS/MTX300 cells. Furthermore, the apoptosis-inducing effects of bufalin were apparently not affected by the presence of high levels of DHFR protein. Indeed, bufalin was able to induce apoptosis equally in both MTX-sensitive and MTX-resistant cell lines without regard to and without altering the DHFR expression. These data indicate the lack of cross-resistance mechanisms between bufalin and MTX, and that bufalin may be useful as an alternative chemotherapeutic agent in osteosarcoma patients unresponsive (resistant) to MTX.
In conclusion, we demonstrated that bufalin induced apoptosis and G2/M phase cell cycle arrest in human osteo-sarcoma U-2OS and U-2OS/MTX300 cells. The induction of apoptosis is apparently mediated through a tumor suppressor protein p53-dependent pathway, which involves proteins of the Bcl-2 family. These results suggest that bufalin may be applicable as a medical treatment for osteosarcoma. In addition, data from our study indicate that bufalin has strong activity, not only in MTX-sensitive U-2OS cells, but also in MTX-resistant U-2OS/MTX300 cells, without being affected by or affecting DHFR expression. These findings indicate that bufalin may be useful as an alternative chemotherapeutic agent in osteosarcoma patients unresponsive to MTX.
Acknowledgement
We thank Dr Massimo SERRA (Laboratorio di Ricerca Oncologica, Istituti Ortopedici Rizzoli, Bologna, Italy) for providing the U-2OS and U-2OS MTX300-resistant cell lines.
References
- Ferguson WS, Goorin AM. Current treatment of osteosarcoma. Cancer Invest 2001;19:292-315.
- Bramwell VH. Osteosarcomas and other cancers of bone. Curr Opin Oncology 2000;12:330-6.
- Davis AM, Bell RS, Goodwin PJ. Prognostic factors in osteosarcoma: a critical review. J Clin Oncol 1994;12:423-31.
- Uchida A, Myoui A, Araki N, Yoshikawa H, Shinto Y, Ueda T. Neoadjuvant chemotherapy for pediatric osteosarcoma patients. Cancer 1997;79:411-5.
- Link MP, Goorin AM, Horowitz M, Meyer WH, Belasco J, Baker A, et al. Adjuvant chemotherapy of high-grade osteosarcoma of the extremity: updated results of the Multi-Institutional Osteosarcoma Study. Clin Orthop Relat Res 1991.8-14.
- Bertino JR, Goker E, Gorlick R, Li WW, Banerjee D. Resistance mechanisms to methotrexate in tumors. Stem Cells 1996;14:5-9.
- Slansky JE, Li Y, Kaelin WG, Farnham PJ. A protein synthesis-dependent increase in E2F1 mRNA correlates with growth regulation of the dihydrofolate reductase promoter. Mol Cell Biol 1993;13:1610-8.
- Banerjee D, Mayer-Kuckuk P, Capiaux G, Budak-Alpdogan T, Gorlick R, Bertino JR. Novel aspects of resistance to drugs targeted to dihydrofolate reductase and thymidylate synthase. Biochim Biophys Acta 2002;1587:164-73.
- Guo W, Healey JH, Meyers PA, Ladanyi M, Huvos AG, Bertino JR, et al. Mechanisms of methotrexate resistance in osteo-sarcoma. Clin Cancer Res 1999;5:621-7.
- Serra M, Reverter-Branchat G, Maurici D, Benini S, Shen JN, Chano T, et al. Analysis of dihydrofolate reductase and reduced folate carrier gene status in relation to methotrexate resistance in osteosarcoma cells. Ann Oncol 2004;15:151-60.
- Hattinger CM, Reverter-Branchat G, Remondini D, Castellani GC, Benini S, Pasello M, et al. Genomic imbalances associated with methotrexate resistance in human osteosarcoma cell lines detected by comparative genomic hybridization-based techniques. Eur J Cell Biol 2003;82:483-93.
- Panesar NS. Bufalin radioimmunoassays: in search of the endogenous digitalis-like substance. J Immunoassay 1994;15:371-91.
- Hong Z, Chan K, Yeung HW. Simultaneous determination of bufadienolides in the traditional Chinese medicine preparation, Liu-Shen-Wan, by liquid chromatography. J Pharm Pharmacol 1992;44:1023-6.
- Krenn L, Kopp B. Bufadienolides from animal and plant sources. Phytochemistry 1998;48:1-29.
- Watabe M, Kawazoe N, Masuda Y, Nakajo S, Nakaya K. Bcl-2 protein inhibits bufalin-induced apoptosis through inhibition of mitogen-activated protein kinase activation in human leukemia U937 cells. Cancer Res 1997;57:3097-100.
- Watabe M, Ito K, Masuda Y, Nakajo S, Nakaya K. Activation of AP-1 is required for bufalin-induced apoptosis in human leukemia U937 cells. Oncogene 1998;16:779-87.
- Kawazoe N, Watabe M, Masuda Y, Nakajo S, Nakaya K. Tiam1 is involved in the regulation of bufalin-induced apoptosis in human leukemia cells. Oncogene 1999;18:2413-21.
- Nasu K, Nishida M, Ueda T, Takai N, Bing S, Narahara H, et al. Bufalin induces apoptosis and the G0/G1 cell cycle arrest of endometriotic stromal cells: a promising agent for the treatment of endometriosis. Mol Hum Reprod 2005;11:817-23.
- Yeh JY, Huang WJ, Kan SF, Wang PS. Effects of bufalin and cinobufagin on the proliferation of androgen dependent and independent prostate cancer cells. Prostate 2003;54:112-24.
- Serra M, Scotlandi K, Manara MC, Maurici D, Lollini PL, De Giovanni C, et al. Establishment and characterization of multidrug-resistant human osteosarcoma cell lines. Anticancer Res 1993;13:323-9.
- Rui H. Research and development of cancer chemopreventive agents in China. J Cell Biochem Suppl 1997;27:7-11.
- Numazawa S, Shinoki M, Ito H, Yoshida T, Kuroiwa Y. Involvement of Na+,K+-ATPase inhibition in K562 cell differentiation induced by bufalin. J Cell Physiol 1994;160:113-20.
- Jing Y, Ohizumi H, Kawazoe N, Hashimoto S, Masuda Y, Nakajo S, et al. Selective inhibitory effect of bufalin on growth of human tumor cells in vitro: association with the induction of apoptosis in leukemia HL-60 cells. Jpn J Cancer Res 1994;85:645-51.
- Jing Y, Watabe M, Hashimoto S, Nakajo S, Nakaya K. Cell cycle arrest and protein kinase modulating effect of bufalin on human leukemia ML1 cells. Anticancer Res 1994;14:1193-98.
- Borner C. The Bcl-2 protein family: sensors and checkpoints for life-or-death decisions. Mol Immunol 2003;39:615-47.
- Miyashita T, Reed JC. Tumor suppressor p53 is a direct transcriptional activator of the human bax gene. Cell 1995;80:293-9.
- Karpinich NO, Tafani M, Rothman RJ, Russo MA, Farber JL. The course of etoposide-induced apoptosis from damage to DNA and p53 activation to mitochondrial release of cytochrome c. J Biol Chem 2002; 277: 16 547–52.
- Ryan KM, Phillips AC, Vousden KH. Regulation and function of the p53 tumor suppressor protein. Curr Opin Cell Biol 2001;13:332-7.
- el-Deiry WS, Tokino T, Velculescu VE, Levy DB, Parsons R, Trent JM, et al. WAF1, a potential mediator of p53 tumor suppression. Cell 1993;75:817-25.
- Salomons GS, Brady HJ, Verwijs-Janssen M, Van Den Berg JD, Hart AA, Van Den Berg H, et al. The Bax alpha: Bcl-2 ratio modulates the response to dexamethasone in leukaemic cells and is highly variable in childhood acute leukaemia. Int J Cancer 1997;71:959-65.