Effects of notoginosides on proliferation and upregulation of GR nuclear transcription factor in hematopoietic cells1
Introduction
Notoginseng is a Chinese medicinal herb which belongs to the panax pseudo-ginseng plant of the Araliaceae family. It has been used in clinics for thousands of years as a treatment for “antithrombosis and vascular diseases”. The effective components of notoginseng are mainly panax notoginosides (PNS), which contains the notoginsenoside monomer of R1, Rg1, Rb1, Rg2, Rh1, and Rd, etc. Recently, investigators have found some pharmacological actions of PNS on the central nervous, cardiovascular, and immune systems[1,2], but little is known about its effects on human CD34+ hematopoietic stem/progenitor cells. The mature blood cells are derived from undifferentiated stem cells, progenitor cells, and precursor cells through a complex series of proliferation, differentiation, maturation, and apoptosis. The stem cell compartment is made up of rare primitive cells, which are multipotential and maintain the capacity to give rise to large numbers of progenitor cells; they also have a high self-renewal capacity. The progenitor cell compartment comprises mainly of cells with the capacity to differentiate along one lineage.
The glucocorticoid receptor (GR) is a member of the nuclear hormone receptor superfamily of ligand-activated transcription factors. The receptor molecules consist of three functional domains: hormone-binding domain, the DNA-binding domain, and the transactivation region on the amino-terminal side[3]. GR is expressed in a wide variety types of cell and tissues, but its expression level is different. Inactivated GR is bound to a large protein complex and localized in the cytoplasm. When glucocorticoid (GC) binds to GR, the protein complex dissociates and the GC/GR complex translocates to the nucleus where it binds to GC response elements resulting in the transcriptional upregulation of gene transcription[4,5].The influence on the nuclear transcription factor of the glucocorticoid receptor (GR-NTF) initiated by PNS has not been reported yet. In this study, the proliferation effects of PNS, extracted from the notoginseng herb on CD34+ hematopoietic stem cells, granulocytic, erythrocytic, and megakaryocytic progenitor cells of human bone marrow, were observed by colony forming assay of all lineage mixtures, granulocytic, erythrocytic, and megakaryocytic progenitor cells (CFU-GEMM, CFU-GM, CFU-E, and CFU-MK) in semisolid culture. The GR-NTF was detected to recognize the GC signaling pathway initiated by PNS and to elucidate its action related with proliferation of the human hematopoietic cells lines of granulocytic HL-60, erythrocytic K562, megakaryocytic CHRF-288, and Meg-01 cells.
Materials and methods
Preparation of bone marrow nuclear cells Bone marrow samples were obtained from human resected ribs during thoracotomy without hematology disorders, with the permission of the patients, and approval by The Human Ethics Committee at The First Affiliated Hospital of Zhejiang Chinese Medical University (Hangzhou, Zhejiang). The marrow smear showed a normal hematological cellular profile. The mononuclear cells were isolated by Ficoll-Paque gradient centrifugation (specific gravity 1.077 g/mL) from bone marrow, and were suspended in Iscove’s Modified Dulbecco’s medium (IMDM) after being washed 3 times in the medium. The number of mononuclear cells was counted before plating.
Purification of CD34+ stem/progenitor cells The method was described previously in our study[6] and modified in this paper. The CD34+ cells were isolated from mononuclear cells of bone marrow using immune beads of Dynal CD34 cell selection system (Dynal, Oslo, AS, Norway). The immuno-magnetic beads was washed 3 times and resuspended in 1 mL isolation buffer. The mononuclear cells were then added to the isolation buffer containing immunomagnetic beads and shaken gently for 30 min at 4 °C. The CD34+ cells, conjugated with the immunomagnetic beads, were obtained by putting them on a magnetic particle concentrator (Dynal, Norway) after co-culture. For the detachment of the immunomagnetic beads from the purified CD34+ cells, 0.1 mL detachment buffer was added to the mixture and incubated for 15 min at 37 °C in a water bath, and then 2 mL isolation buffer was added and thoroughly shaken with a vortex. Finally, the tube was placed on a magnetic particle concentrator to separate the beads from the CD34+ cells. The CD34+ cells in the isolation buffer were harvested by centrifugation and suspended in the medium; some of the cells were stained with trypan blue for their survival. The purity of the CD34+ cells was detected as 86%–93% by flow cytometry with the monoclonal antibody against CD34.
CD34+ cell culture for the CFU-GEMM colony and cytological identification The semi-solid culture system of CFU-GEMM was similar to our previous report[6] and modified in this study, which was composed of 20% fetal bovine serum (Gibco, Grand Island, NY, USA), 1% bovine serum albumin (BSA, Sigma, St Louis, MO, USA), 1×10-5 mol/L 2-mercapto-ethanl, 0.3% agar, 10 ng/mL stem cell factor (SCF, Pepro Tech, Rehovot, Israel), 20 ng/mL interleukin-3 (IL-3, Strathmann, Hanover, Germany), and 50 ng/mL granulocyte-colony stimulating factor (G-CSF, Strathmann, Hanover, Germany). The CD34+ cells, from the bone marrow of 9 people, were expos-ed to PNS at a final concentration of 0, 10, 25, 50, and 100 mg/L, respectively. The culture system described above was mixed and plated into 24-well plate in triplicate for each sample with 5×103 CD34+ cells/ 0.5 mL per well, then incubated at 37 oC in a humidified atmosphere supplemented with 5% CO2. The number of CFU-GEMM colonies (≥100 cells) was counted under an inverted microscope on d 14 after initial plating. The CFU-GEMM colonies were made up of 3 lineage blood cells, including granulocytes, erythrocytes, and megakaryocytes. For the identification of these cells, granulocytes within the colonies were revealed as black with peroxidase staining, erythrocytes displayed as red wine colour with dimethoxybenzidine staining, and human megakaryocytes were identified with monoclonal antibodies.
Bone marrow culture for CFU-E and CFU-GM colonies The mononuclear cells from the bone marrow of 10 people were plated to semi-solid culture. The culture system of CFU-E was similar to the authors’ previous study[7] which contained 20% fetal bovine serum, 1×10-5 mol/L 2-mercapto-ethanal, 5% phytohemagglutinin-leukocyte conditioned medium, 2 U/mL recombinant human erythropoietin (EPO, Amgen, Thousand Oaks, CA, USA), and 0.3% agar of IMDM medium. The culture system described above was mixed and plated in triplicate wells for each sample with 105 mononuclear cells/0.5 mL per well, and subsequently incubated at 37 oC for 7 d within a humidified atmosphere supplemented with 5% CO2. The nuclear erythrocytes within the CFU-E colony were revealed as red with dimethoxybenzidine staining in situ[8], which was helpful for determining the erythro-cytes. The CFU-E (≥8 cells) colonies, which consisted of positive cells, were counted using an inverted microscope. The culture system of CFU-GM contained 20% fetal bovine serum, 10 ng/mL recombinant human granulocyte/macrophage-colony stimulating factor (GM-CSF, Sandoz, São Paulo, Brazil), and 0.3% agar, which was plated in triplicate with 1×105 bone marrow mononuclear cells/0.5 mL per well. The culture system was then incubated at 37 oC for 7 d. The colony numbers of CFU-GM (≥40 cells) were scored on an inverted microscope.
Bone marrow culture for the CFU-MK colony and identification of megakaryocytes The mononuclear cells from the bone marrow of 10 people were plated to semisolid culture. The culture system of CFU-MK, set up as described in another study with modifications[8], was composed of 20% fetal bovine serum, 1% BSA, 1×10-5 mol/L 2-mercaptoethanl, recombinant human thrombopoietin (rHu TPO, Pepro Tech, Rehovot, Israel) with final concentration of 30 ng/mL, and 0.8% methylcellulose as viscous support. The semisolid culture of CFU-MK was performed in triplicate wells for each sample with 2×105 nuclear cells/0.5 mL per well, and the colony numbers of CFU-MK (≥4 cells) were evaluated on d 14 after initial planting. The megakaryocytes of the CFU-MK colony could be identified directly according to their large size, and well demarcated translucent as well as hyaline cytoplasm under an inverted microscope of high optical quality. For the identification of the megakaryocytes, all cells of the colony were harvested for preparation slides by washing out methylcellulose on d 14 after plating. The megakaryocytes on the slides were identified with monoclonal antibodies against CD41 and CD42 by streptavidin-alkaline phosphatase (SAP) enzyme conjugation reaction. The positive cells with red were regarded as megakaryocytes.
Culture of human hematopoietic cell lines Three lineage cell lines of human granulocytic HL-60, erythrocytic K562, megakaryocytic CHRF-288, and Meg-01 cells were incubated in IMDM supplemented with 10% new-born bovine serum without any growth factors. CHRF-288 and Meg-01 cells were generously gifted from Prof Beng Hock CHONG (St George Hospital, Sydney, Australia) who purchased the cells from ATCC (Manassas, VA, USA).
PNS stimulating test for hematopoietic progenitor cells PNS was extracted and purified from the notoginseng herb by Sanxi Zhengkang Pharmaceutical Company (Xi’an, China), which dissolves drastically in water. It is a clinical treatment drug, administered by muscle or vein injection, and contains 50 mg/mL notoginosides per vial (certification N
Preparation of nuclear extracts The nuclear extracts were similarly prepared to those of our previous study[9,10]. After washing with ice-cold phosphate-buffered saline, the cells were resuspended in ice-cold hypotonic buffer A [10 mmol/L Hepes (pH 7.9), 10 mmol/ L KCl, 1 mmol/L EDTA, 1 mmol/L dithiothreitol (DTT), 0.5 mmol/L phenymethyl-suphonyl fluoride (PMSF), 10 mg/ mL aprotinin, leupeptin, antipain, and pepstatin (Sigma, St Louis, MO, USA)] for 15 min. Subsequently, 0.6% Nonidet P-40 was added, and the sample was vortexed for 10 s. Nuclei were separated from the cytosol by centrifugation at 13 000 r/min for 15 min and resuspended in hypotonic buffer C (20 mmol/ L Hepes, 25% glycerol, 0.4 mmol/ L NaCl, 1 mmol/ L EDTA, 1 mmol/ L DTT, 0.5 mmol/ L PMSF, and 10mg/ mLleupeptin, antipain, aprotein, and pepstatin) and briefly sonicated on ice. Nuclear extracts were obtained by centrifugation at 13 000 r/min for 20 min at 4 oC. The supernatant fluid was the nuclear extracts which were measured by the Bradford method using the protein dye reagent (Bio Rad, Hercules, CA, USA) for the protein concentration of each sample.
Western blot analysis Western blotting was performed as our previous study[10,11]. 10 μg nuclear protein was loaded,with an equal volume of 2×electrophoresis sample buffer, and separated by SDS-PAGE with 7.5% acrylamide. The proteins were transferred to a nitrocellulose membrane (Amer-sham, Buckinghamshire, UK) using an electroblotting apparatus (Bio Rad, Hercules, CA, USA). The membranes were submerged in a blocking buffer containing 1.0% bovine serum albumin in TBS solution (150 mmol/ L NaCl, 50 mmol/L Tris, pH 7.5) for 1 h at room temperature Sub-sequently, the membranes were incubated in primary antibody (Santa Cruz, CA, USA) at a dilution of 1:1000 against the amino terminus (E-20) or carboxyl terminus (P-20) of GR-NTF for an additional 1 h at room temperature. After washed 3 times, the membranes were incubated with anti-rabbit horseradish peroxidase-conjugated secondary antibody (Santa Cruz, CA, USA) at a dilution of 1:2000 for 45 min at room temperature. After washed again, the membranes were visualized. The special bands from the conjugation reaction of the protein antigen and antibody were visualized by the ECL kit (Santa Cruz, CA, USA), and the density of the bands was analyzed after scanning image on X film (Kodak, Shanghai, China). The experiment was repeated 3 times.
Electrophoretic mobility shift assay (EMSA) EMSA was performed as our previous study[9,11]. For the binding reaction, 10 mg nuclear extracts from each sample were incubated in a 25 µL total reaction volume containing 20 mmol/ L Hepes (pH 7.9), 50 mmol/ L NaCl, 0.1 mmol/ L EDTA, 1 mmol/ L DTT, 5% glycerol, 200 mg/mL BSA, and 2.5 mg poly (dI/dC) (Boehringer Ingelheim, Germany) for 15 min at 4 oC. The probe used in this study was the double-stranded nuclear transcription factor of the GR (Santa Cruz, CA, USA) consensus oligonucleotide containing the binding site for GR-NTF: 5'-AGA GGA TCT GTA CAG GAT GTT CTA GAT-3' GR-NTF consensus ds-oligonucleotide was radiolabeled with the polynucleotide kinase (Boehringer Ingelheim, Ingelheim, Germany) and [α-32P]ATP (Amersham, Buckinghamshire, England). 50 000 cpm GR-NTF oligonucleotide was added to the reaction mixture and incubated for 30 min at room temperature. The reaction products were analyzed by electrophoresis in 6% polyacrylamide gel with 0.25-fold TBE running buffer (22.3 mmol/ L Tris, 22.2 mmol/ L borate, and 0.5 mmol/ L EDTA). The gel was dried and the complexes of GR-NTF with DNA were visualized by autoradiography. The experiment was repeated 3 times.
Statistical analysis Triplicate experimental results were pooled and expressed as mean±SD. Student’s t-test was used to determine statistical difference between the experimental groups and control groups. P<0.01 or P<0.05 were considered statistically significant.
Results
Effects of PNS on the proliferation of CD34+ cells The CFU-GEMM colonies, derived from the culture of CD34+ cells, were made up of 3 lineage blood cells, including granulocytes, erythrocytes, and megakaryocytes. The colony growth pattern was seen (≥100 cells) in vitro as shown in Figure 1A. Table 1 shows a control of non-PNS treatment; CFU-GEMM colony numbers were 47.8±11.1/5×103 CD34+ cells on d 14 after initial planting, while the CD34+ cells from the 9 samples were exposed to PNS at the concentrations of 10, 25, and 50 mg/L, the frequencies of CFU-GEMM colony were significantly more than those of the non-PNS control (P<0.01 and 0.05). The highest colony formation was noticed at 25 mg/ L PNS with an increasing rate of 34.7±16.0% (P<0.01). The results indicated that PNS could enhance the proliferation of CD34+ hematopoietic stem cells to increase the formation of CFU-GEMM colony in vitro.
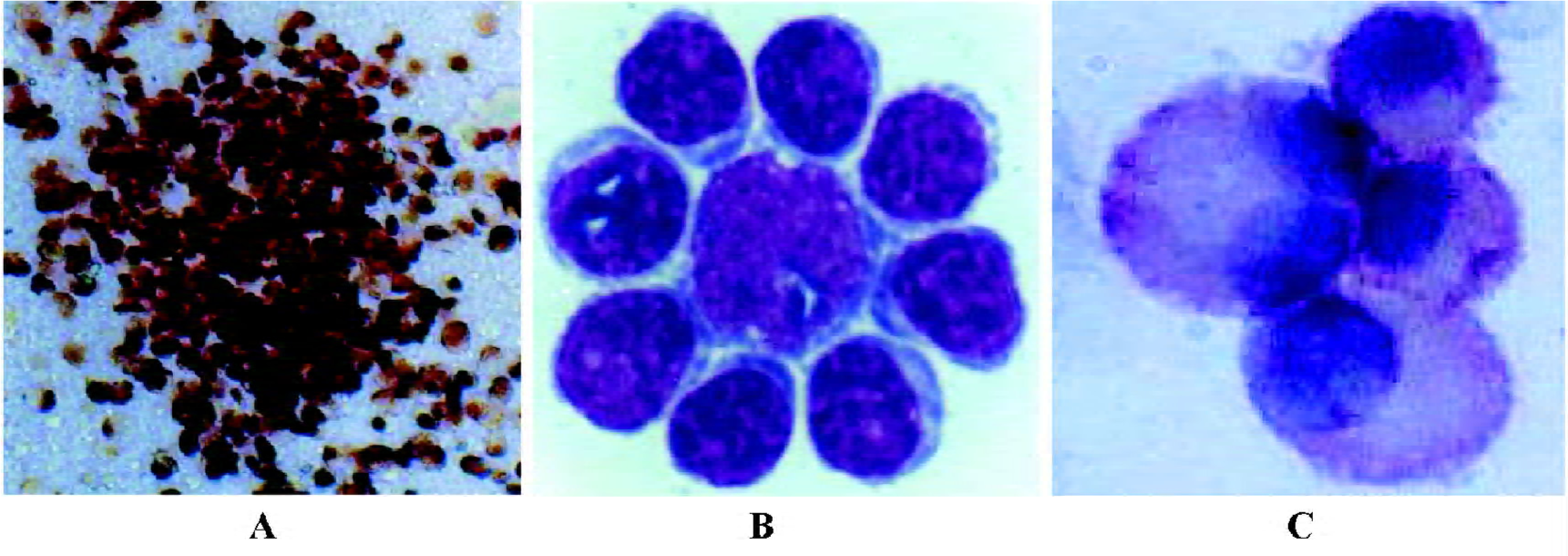
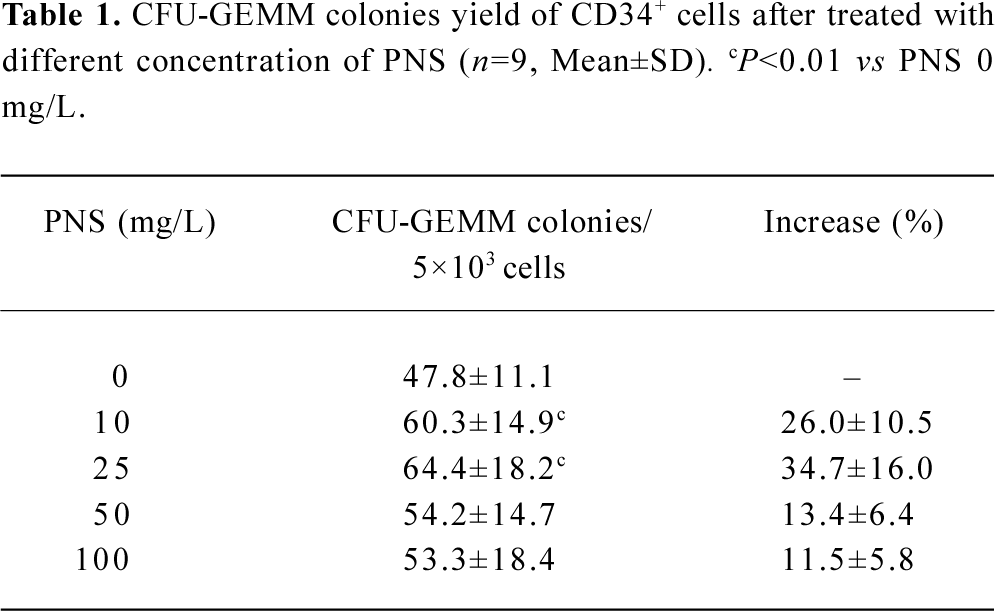
Full table
Effects of PNS on the proliferation of CFU-GM and CFU-E The results of CFU-GM and CFU-E colony assay in the semisolid culture system from 10 samples exposed to PNS at concentrations of 0, 10, 25, 50, and 100 mg/L on d 7 after initial planting. The colony formation of CFU-GM was 49.4±4.2/1×105 mononuclear cells in the non-PNS control, while the frequencies of CFU-GM colony in 3 groups of PNS 10, 25, and 50 mg/ L were significantly more than those of the no-PNS control, respectively (P<0.01), with an increasing rate of 22.5%, 37.4%, and 39.3% over the non-PNS control, respectively. Meanwhile, CFU-E colony (shown as Figure 1B with Wright staining) numbers from bone marrow culture were 35.7±3.0/1×105 mononuclear cells in the non-PNS control, while in the presence of PNS (20 and 50 mg/ L), the colony numbers of CFU-E were significantly more than those of the non-PNS control (P<0.01), respectively. These results indicated that PNS could enhance the proliferation of human granulocytic and erythrocytic progenitor cells in a dose-dependent manner to increase the formation of CFU-GM and CFU-E colonies in vitro.
Effects of PNS on the proliferation of CFU-MK The results of CFU-MK colony assay in the semi-solid culture system from the 10 samples exposed to PNS at concentrations of 0, 10, 25, 50, and 100 mg/ L on d 14 after initial planting. Megakaryocytic colonies could be divided into 2 kinds according to their growth pattern under the inverted microscope: loose aggregates of 3–10 cells without additional hematopoietic cell lineages present, and megakaryocytes within mixed colonies which contained the other lineages of erythrocytic and granulocytic cells. The cells in red were regarded as megakaryocytes labeled with monoclonal antibody against CD41 or CD42 by SAP enzyme conjugation reaction (shown as Figure 1C with CD41 labeling). Table 2 shows that the CFU-MK colony numbers from 10 samples were 42.8±5.2/1×105 mononuclear cells in the non-PNS control, while in response to PNS (25 and 50 mg/ L), the colony numbers of CFU-MK were significantly more than those of the non-PNS control (P<0.01), respectively. The results suggest that PNS could enhance the proliferation of megakaryocytic progenitor cells to increase the formation of CFU-MK colony in vitro.
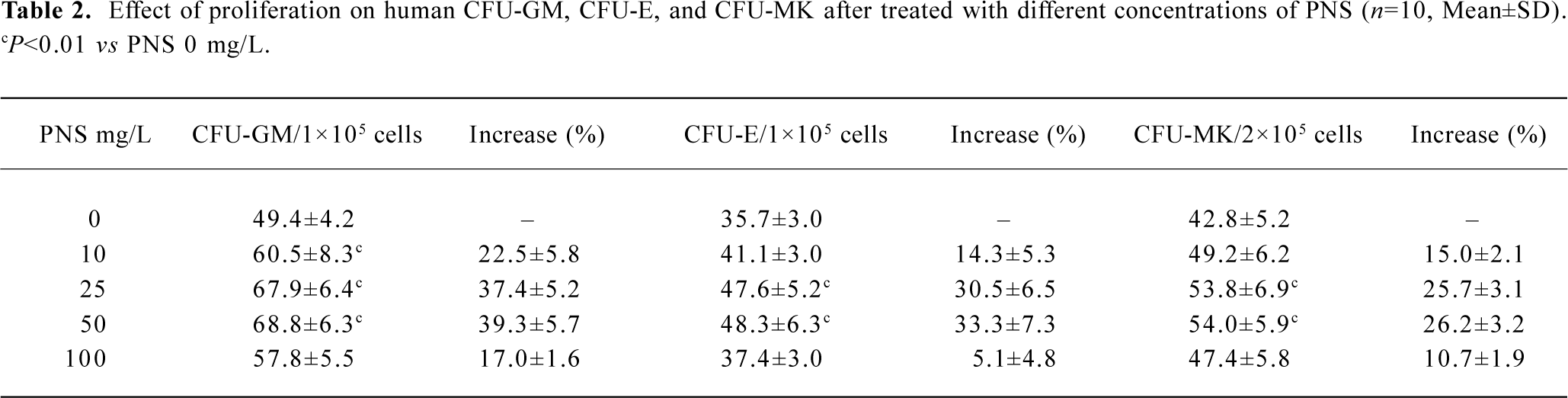
Full table
Upregulated expression of the GR-NTF protein induced by PNS The expression level of the GR-NTF protein was determined by Western blotting in 4 cell lines of HL-60, K562, CHRF-288, and Meg-01 after treated with PNS, respectively (Figure 2). By using the antibodies against the amino terminus and carboxyl terminus, respectively, the specific bands of 95 kDa that appeared were derived from the conjugation reaction of the GR-NTF protein with the antibodies againstthe amino terminus and carboxyl terminus, respectively. The band density of the GR-NTF protein (amino terminus) initiated by PNS was 2.6-, 2.8-, and 2.4-fold (Figure 2, lanes 4, 6, and 8), respectively higher than those of the non-PNS control (Figure 2, lanes 3, 5, and 7) in 3 cell lines of K562, CHRF-288, and Meg-01. The band density of the GR-NTF protein (carboxyl terminus) initiated by PNS was 1.3-, 3.9-, and 2.7-fold (Figure 2, lanes 4, 6, and 8), respectively, higher than those of the non-PNS control in 3 cell lines of K562, CHRF-288, and Meg-01. However, there was no obvious difference of the expression level of the GR-NTF protein (both the amino terminus and carboxyl terminus) between the PNS-treated and untreated HL-60 cells. Meanwhile, the positive control of Dex also raised the expression of the GR-NTF protein (both the amino terminus and carboxyl terminus) compared with the untreated K562 and CHRF-288 cells. The results in Figure 2 indicate that PNS can increase the expression level of the GR-NFT protein in K562, CHRF-288, and Meg-01cells, but not in HL-60 cell. Since Dex was used for the positive control only, and the effect of PNS in K562 and CHRF-288 cells was more obvious than those in HL-60 and Meg-01 cells, we selected 2 target cells to study the expression level of the GR-NTF protein induced by Dex. The results suggested that Dex also could increase the expression level of the GR-NFT protein in K562 and CHRF-288 cells.
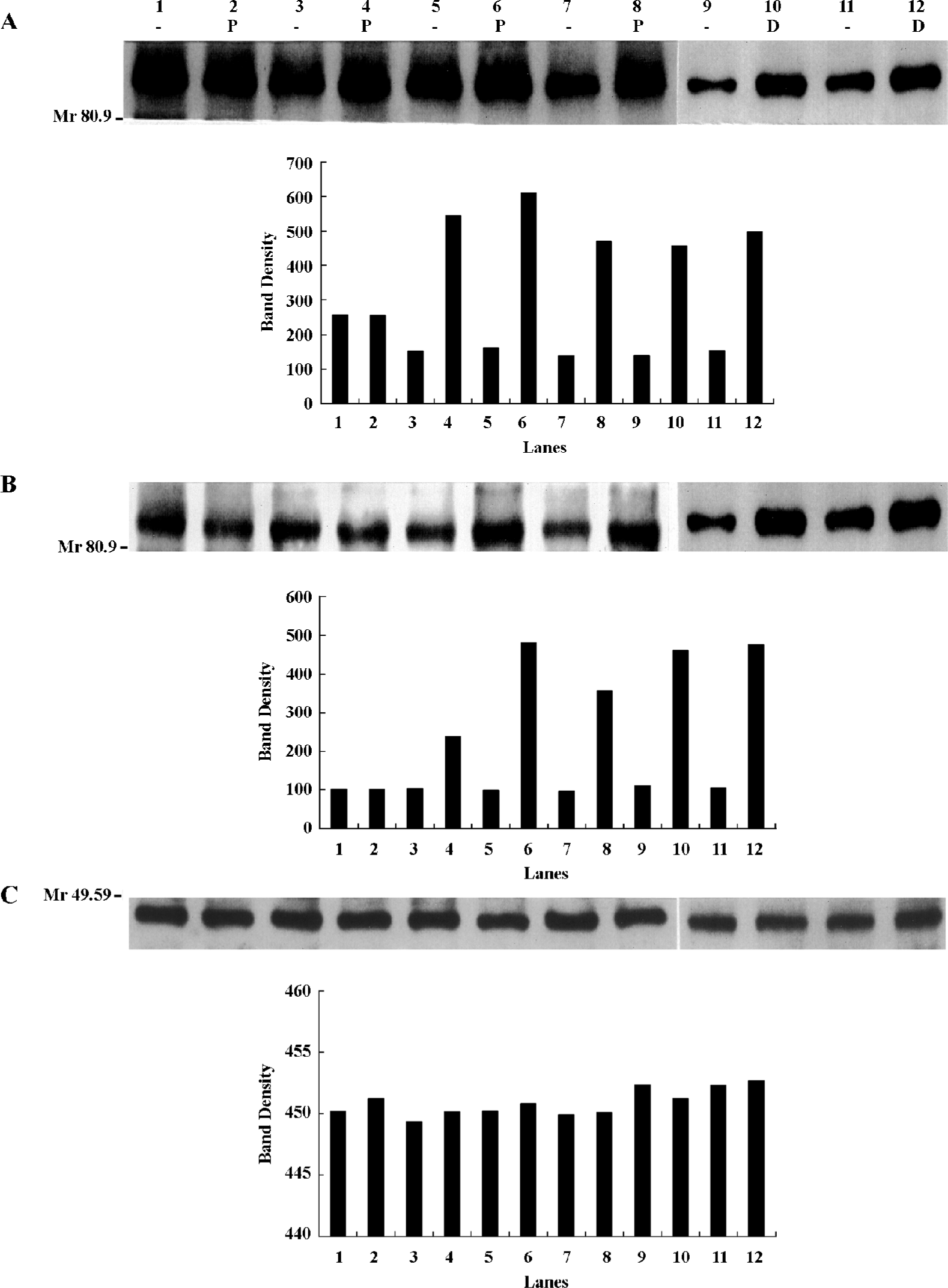
Upregulation of GR-NTF binding activity induced by PNS Evidence from the colony assay of CFU-GEMM, CFU-GM, CFU-E, CFU-MK, and GR-NTF protein expression by Western blotting supported the hypothesis that GR-NTF may be involved in the effects of PNS on the proliferation in hematopoietic cells. To confirm this possibility, EMSA was performed with a 32P-labelled GR-NTF consensus oligonucleotide(Figure 3). The binding complex of the GR-NTF protein with DNA revealed a single major band in the nuclei of cells. This complex band was competitively abolished by 500-fold excess of unradiolabeled GR-NTF consensus oligonucleotide (data not shown). The GR-NTF binding activity initiated by PNS was apparently elevated to form higher density bands (the complex of GR-NTF and DNA) in the nuclei of K562 and CHRF-288 cells (Figure 3, lanes 4 and 6). There was little binding activity of GR-NTF, which appeared as a shallow band in the nuclei of HL-60 cells treated with PNS, although there was no detectable band in the untreated cells. The binding activity of GR-NTF was no obvious difference between the PNS-treated and untreated Meg-01 cells (Figure 3). The results in Figure 3 indicate that PNS could induce the upregulation of GR-NFT binding activity in K562 and CHRF-288 cells, to a lesser extent in HL-60, but not in Meg-01 cells. Meanwhile, the positive control of Dex also could induce the upregulation of GR-NFT binding activity in K562 and CHRF-288 cells.
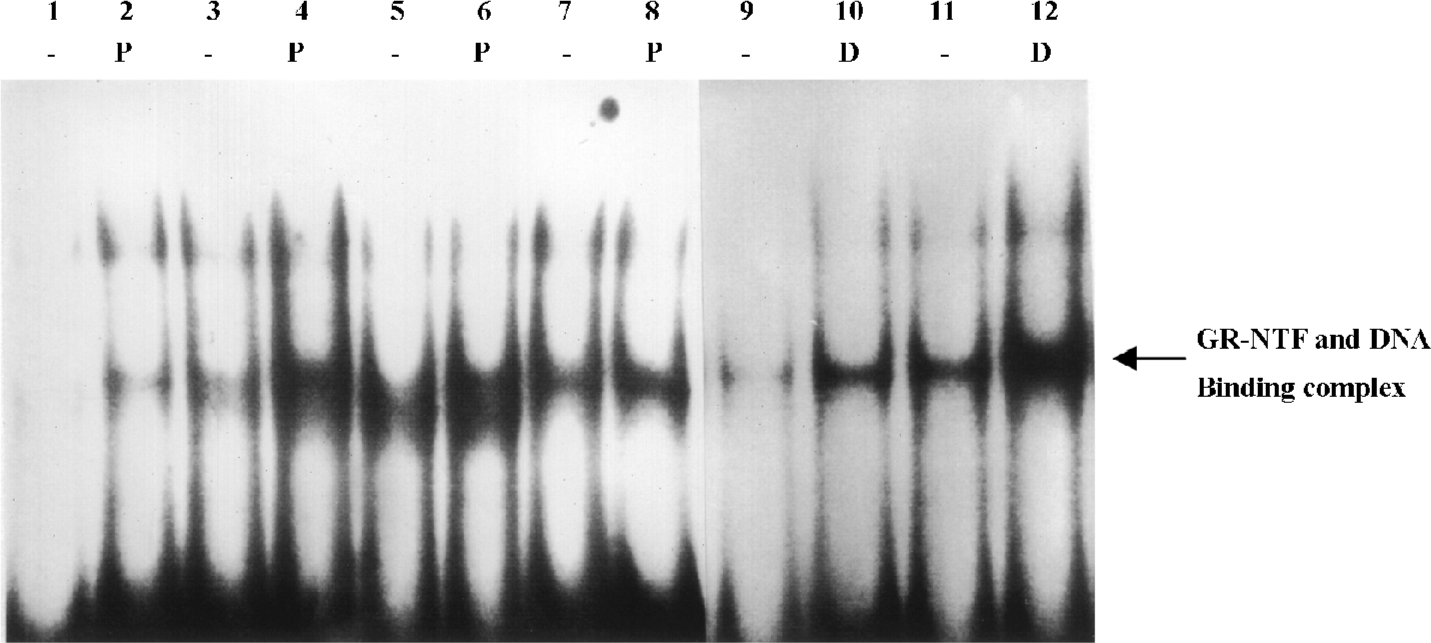
Discussion
GC have been shown to enhance the formation of mouse erythroid colonies in vitro[12]. In the presence of GC, lower concentrations of erythropoietin were required to induce maximal proliferation of erythroid progenitor cells in vivo, GC can restore normal erythropoiesis in pediatric aplastic anemia[13]. Lindern et al reported that the addition of the GR ligand Dex to EPO and SCF allowed the establishment of mass cultures of normal erythroid progenitors from mononuclear cells of human umbilical cord blood, bone marrow, and peripheral blood[14]. Erythroid progenitor cells could be induced to terminal erythroid differentiation upon the removal of SCF and Dex. Furthermore, GR-NTF is a key regulator of the decision between self-renewed and differentiation in erythroid progenitor cells[15].
The hematopoietic stem cells are characterized by the expression of the CD34 antigen, which distinguishes them from other immature cell types. In the early stage of hematopoietic cells, the expression of the CD34 antigen is at its highest level, and the CD34 antigen gradually decreases and finally disappears along with cell differentiation and maturation[16]. Therefore, CD34+ hematopoietic stem cells are the best target cells for the investigation of hematopoiesis regulation, and they are applied for stem cell transplantation and gene therapy. Traditionally, transplantation of hematopoietic stem cell is mainly used for the treatment of malignant blood disorders. Recent advances have made the transplantation of CD34+ hematopoietic stem cells a hot research field, which will provide new therapeutic approaches to the treatment of solid tumors, immunological disorders, and heredity diseases. In this study, we obtained CD34+ cells with high purity from human bone marrow by immunomagnetic beads. The amount of CD34+ cells was enough for the semisolid culture to observe the proliferation effects of PNS on human CD34+ cells.
Wang et al reported that PNS promoted the proliferation of granulocytic progenitors in both normal and aplastic anemia mice[17]. The supernatant prepared from the culture of mice spleen cells and fibroblastic cells after PNS treatment revealed stimulating activity on hematopoietic progenitor cells. It was suggested that PNS might induce fibroblastic cells and lymphocytes to secrete various kinds of cytokines for hematopoiesis[18]. The report also indicated that PNS induced differentiation of the leukemia cell line K562 into granulocytic lineage cells. Other investigators found that PNS was effective in preventing 60Co-irradiated mice from hematopoiesis suppression through an increasing quantity of hemoglobin and number of granulocytes and enhancing the proliferation of progenitors in radiation mice[19]. We have reported the effects of PNS on hematopoiesis through a series of studies, including the mouse model with aplastic anemia, the proliferation test of hematopoietic cells, and intracellular transcription regulation. It was indicated that PNS could increase the number of peripheral white blood cells, improve hematopoiesis function of bone marrow, and promote the proliferation of hematopoietic progenitors of CFU-GM and CFU-E in mice with immune-mediated aplastic anemia when bone marrow was suppressed[20]. Also, PNS could induce CD34+ hematopoietic stem cell differentiation committed towards granulocytes by exposure to PNS at optimal concentrations in the suspension culture. The percentage of the granulocyte-specific marker CD33+ and CD15+ cells were much higher than those of the no-PNS control by analysis of flow cytometry[21]. Also, we observed the differentiation effects of PNS on the blastic cell lines above with flow cytometry analysis; the results showed no obvious differentiation phenomena when treated with PNS, which may be explained by their abnormal heterogeneity, incapable of differentiation, which is different from normal hematopoietic progenitor cells. In thisstudy, we observed that PNS could not only promote the proliferation of CD34+ cells and significantly raise the colony numbers of CFU-GEMM in vitro, but also enhance the proliferation of granulocytic, erythrocytic, and megakaryocytic progenitors of bone marrow to increase CFU-GM, CFU-E, and CFU-MK colony formation in vitro. Our results suggest that PNS might act as a growth factor or synergistic efficacy with growth factors, such as SCF, EPO, GM-CSF, and IL-3 in the proliferation of hematopoietic stem/progenitor cells. It may provide useful evidence for the possible application of PNS in treating blood diseases in the future.
In order to explore the intracellular signal pathway correlated with proliferation and differentiation induced by PNS in hematopoietic cells, we observed the regulation of GATA family transcription factors, GATA-1 and GATA-2, AP-1 family transcription factors NF-E2, c-Jun, c-Fos, and NF-κB family transcription factors, c-Rel and NF-κB proteins, by Western blotting. The majority of these transcription factors were upregulated at distinct degrees in response to PNS, although displaying different susceptibility to PNS among 4 cell lines of HL-60, K562, CHRF-288, and Meg-01 cells[22,23]. EMSA results indicated that the DNA binding activity of GATA and AP-1 initiated by PNS was apparently elevated. The immune precipitation showed that both GATA-1 and GATA-2 proteins were at a phosphorylated status[20,21]. It suggested that PNS might play a role in the upregulation of gene expression related to proliferation and differentiation in hematopoietic cells, through increasing synthesis, DNA binding activity of multiple transcription factors, and their phosphorylated status.
In this study, the nuclear transcription factor of GR was detected to elucidate whether PNS was involved in the signaling pathway similar to GC in relation to the proliferation of hematopoietic cells. Western blotting showed that GR-NTF protein levels of either the amino or carboxyl terminus in 3 cell lines of K562, CHRF-288, and Meg-01 treated with PNS increased by 2.4–2.8-fold and 1.3–3.9-fold over the untreated cells, respectively. Meanwhile, the positive control of Dex also elevated the expression of the GR-NTF protein. GR-NTF binding activity initiated by PNS or Dex apparently elevated to form higher density bands (the complex of GR-NTF and DNA) in K562 and CHRF-288 cells. The little binding activity of GR-NTF appeared as a shallow band in HL-60 cells treated with PNS, although there was no detectable band in the untreated cells. These data suggest that the intracellular signal pathway of PNS is involved in GR-NTF, which might play a role in the upregulation of gene expression, correlated with the proliferation in hematopoietic cells by increasing its synthesis and DNA binding activity.
References
- Jiang KY, Qian ZN. Effects of panax notoginseng saponins on post-hypoxic cell damage of neurons in vitro. Acta Pharmacol Sin 1995;16:399-402. Chinese..
- Wang YF, Yang FC, Zhan Y. The progress on the treatment of cardiocerebrovascular diseases by panax notoginseng saponins. J Yunnan Chin Med Scien & Tradition Chin Med 1997;18:36-8. Chinese..
- Beato M. Gene regulation by steroid hormones. Cell. 1989;56:335-44.
- Wright D, Almlof K, Mcewan T, Gustafsson J, Wright APH. Delieation of a small region within the major transactivation domain of the human glucocorticoid receptor that mediates transactivation of gene expression. Proc Natl Acad Sci USA 1994;91:1619-23.
- Lefstin JA, Thomas JR, Yamamoto KR. Influence of a steroid receptor DNA-binding domain on transcriptional regulatory functions. Genes Dev 1994;8:2842-56.
- Gao RL, Wu CQ, Chong BH. The intracellular signaling pathway initiated by ginsenosides in hematopoietic cells. Chin J Hematol 1999;20:292-5. Chinese..
- Gao RL, Jin JM, Chong BH. The effect of panax ginsenosides on generation of human blood cells. J Zhejiang College of Trad Chin Med 1998;22:37-8. Chinese..
- Abgrall JF, Berthou C, Sensebe L, Le Niger C, Escoffre M. Decreased in vitro megakaryocyte colony Formation in chronic idiopathic thrombocytopenic purpura. Br J Hematol 1993;85:803-12.
- Jin JM, Tao H, Gao RL, Chong BH. Proliferation and differentiation of human CD34+ hematopoietic stem/progenitor cells induced by panax ginsenosides. Chin J Integr Tradi West Med 2000;20:673-6. Chinese..
- Chen XH, Gao RL, Xu WH, Jin JM, Lin XJ. Effect of ginsenosides in inducing proliferation and transcription factor of erythrocytic, granulo-monocytic and megakarocytic cell line. Chin J Integr Tradi West Med 2001;21:40-2. Chinese..
- Gao RL, Wu CQ, Jin JM, BH Chong. Ginsenosides stimulate proliferation of human megakarocytic progenitors through by ginsenosides GATA-1 transcription factor. J Clinic hematol 1999; 12: 50–4. Chinese.
- Golde DW, Bersch N, Cline MJ. Potentiation of erythropoiesis in vitro by dexamethasome. J Clin Invest 1976;57:57-62.
- Zito GE, Lynch EC. Prednisone-responsive congenital erythroid hypoplasia. J Am Med Assoc. 1977;237:991-2.
- Lindern MV, Zauner W, Mellitzer G, Steinlein P, Fritsch G, Huber K, et al. The glucocorticoid receptor cooperates with the erythropoietin receptor and c-kit to enhance and sustain proliferation of erythroid progenitors in vitro. Blood 1999;94:550-9.
- Wessely O, Deiner EM, Beug H, von Lindern M. The glucocorticoid receptor is a key regulator of the decision between self-renewal and differentiation in erythroid progenitors. EMBO J 1997;16:267-80.
- Terstappen LW, Huang S, Safford M, Lansdorp PM, Loken MR. Sequential generation of hematopoietic colonies derived from single no lineage committed CD34+/CD38- progenitors. Blood 1991;77:1218-27.
- Wang YP, Niu H, Jiang R, Wang Y, Zhu BD. Effects of panax notoginseng saponins on proliferation, regulation of erythroid progenitors. J Anatomy 1996;19:138-42. Chinese..
- Jiang R, Wang YP, Wang SL. Effects of total saponins of panax notoginseng saponins on proliferation of CFU-GM and its mechanism. J Chongqin Med Univ 1999;24:14-7. Chinese..
- Chen MT, Zhu PD, Luo YP, Wang YP, Jiang R. Differentiation of K562 cell line induced by three different saponins. Leukemia 1999;81:28-9. Chinese..
- Gai Y, Gao RL, Niu YP, Jin JM, Si YQ. Effects of panax notoginosides on proliferation of hematopoietic progenitor cells in mice with immune-mediated aplastic anemia. Chin J Integr Tradi West Med 2003;23:680-3. Chinese..
- Gao RL, Qian XD, Ma K, Jin JM. Panax notoginosides promotes proliferation and differentiation of human CD34+ hematopoietic stem/progenitor cells. Stem Cell & Cell Therapy 2003;1:38-42. Chinese..
- Gao RL, Xu WH, Chen XH, Qian XD, Wu CQ. Upregulation of transcription factors NF-E2, c-Jun and c-Fos of AP-1 family induced by panax notoginosides in hematopoietic cells. J Exper Hematol 2004;12:16-9. Chinese..
- Gao RL, Xu WH, Lin XJ, Chen XH, Wu CQ. Upregulation of transcription factors GATA-1 and GATA-2 induced by panax notoginosides in hematopoietic cells. Chin J Hematol 2004;25:28-4. Chinese..