Antidiabetic effect of a novel non-thiazolidinedione PPAR γ/α agonist on ob/ob mice1
Introduction
Non-insulin-dependent diabetes mellitus (NIDDM, type 2 diabetes) is characterized by abnormal carbohydrate, fat and protein metabolisms, which are attributed to the diminished production of insulin or defective insulin action (insulin resistance)[1]. Insulin resistance is characterized by the impairment in insulin-regulated metabolic actions, including glucose transport, glycogen synthesis and gene expression characteristics[2]. Insulin resistance is a key factor in the onset and progress of type 2 diabetes. Ameliorating insulin resistance is an important strategy in the development of new pharmacological treatment for type 2 diabetes.
The peroxisome proliferator-activated receptors (PPAR) are ligand-activated nuclear receptors that regulate the expression of genes related to the carbohydrate, lipid and protein metabolisms[1,3]. Up to now, 3 PPAR subtypes have been discovered and characterized (PPAR-α, δ and γ)[4]. Different PPAR subtypes have been shown to play different but crucial roles in some important diseases[4]. PPARγ is expressed mainly in adipose tissues and plays a central role in adipogenesis and glucose homeostasis[3,5]. PPARα is highly expressed in the liver, heart and the skeletal muscle and contributes to lipid metabolism. The activation of PPARγ and PPARα modulates the expression of genes associated with carbohydrate, lipid and protein metabolisms, which in turn influence glucose uptake, insulin sensitivity and lipid metabolism[1,3]. Thus, because of the central role of PPAR isoforms in metabolisms, they have become attractive targets for drug discovery aimed at improving insulin sensitivity[6–9]. Thiazolidinediones (TZD) are a new class of oral antidiabetic agents that improve insulin sensitivity, lipid and glucose homeostasis in type 2 diabetes though the activation of PPARγ[10]. There are currently 2 available TZD for clinical treatment: rosiglitazone and pioglitazone, with a third earlier compound, troglitazone, withdrawn due to hepatoxicity. TZD improve glucose homeostasis by increasing insulin sensitivity in peripheral tissues[11–13]. The activation of PPARα lowers triglycerides and elevates plasma HDL cholesterol levels[14,15]. The lipid modulating activities of fibrates are presumably due to the activation of PPARα. Since type 2 diabetic patients often develop hyperglycemia, insulin resistance, dyslipidemia and other metabolic abnormalities, dual PPARγ/α agonist should be more beneficial in ameliorating major metabolic disorders than either PPARα or PPARγ selective agonists.
The benzopyran derivative T33, originally named T11, is a novel non-thiazolidinedione agent. Our previous study reported its structure and showed that T33 could stimulate the differentiation of 3T3-L1 adipocytes and increase the insulin-induced triglyceride accumulation[16]. Using a cell-based reporter gene assay, T33 was identified as a PPARγ/α dual agonist, which activated human PPARγ and PPARα with EC50 value of 19 and 148 nmol/L (unpublished data). Therefore, in the present study, the antidiabetic and insulin-sensitizing effects of T33 were evaluated in ob/ob mice, a type 2 diabetic animal model that were obese and insulin resistant.
Materials and methods
Compounds T33 and rosiglitazone were synthesized by Prof Yu-she YANG of the Shanghai Institute of Materia Medica. Both compounds were prepared as suspensions in 1% carboxymethylcellulose solution for in vivo studies.
Animals and treatment The ob/ob mice and their lean control (6–7 weeks old), obtained from Jackson Laboratories (Bar Harbor, Maine, USA), were maintained under a 12:12 light-dark cycle with free access to water and food. After 2 weeks of acclimation, the ob/ob mice were weighed and bled via the tail vein after 5 h fasting for the blood glucose values test. The ob/ob mice were assigned to 4 groups based on fasting blood glucose values (first criterion) and initial body weight (second criterion). The ob/ob mice were gavaged once daily with vehicle (1% CMC), T33 (4 mg/kg), T33 (8 mg/kg) or rosiglitazone (4 mg/kg), respectively for 20 d. At the same time, the lean mice were also treated with vehicle (1% CMC). Blood glucose levels were tested regularly for the mice that were fed (tested at 9:00 AM) and fasted (tested at 14:00 PM after 5 h fasting) using a One-Touch Basic Glucose Monitor (Lifescan, Milpitas, CA, USA). Body weight was also measured regularly. All animal experiments were approved by the Animal Care and Use Committee of the Shanghai Institute of Materia Medica, Chinese Academy of Sciences.
Oral glucose tolerance test After 8 d treatment with the compounds, the ob/ob mice were subjected to an oral glucose tolerance test (OGTT). Briefly, the ob/ob mice were made to fast for 5 h and then orally administered with glucose (2.5 g/kg body weight). The blood glucose values were measured via blood drops obtained by clipping the tail of the mice at 0, 30, 60, 120 min after glucose administration. The results of the OGTT were also expressed as integrated areas under the curves (AUC) over 120 min.
Insulin tolerance test After 12 d treatment with the compounds, insulin sensitivity was determined by performing an insulin tolerance test (ITT). After 5 h fasting, the mice were injected with biosynthetic human insulin (Novolin R; Novo Nordisk AIS, Bagsvaerd, Denmark) at 0.4 U/kg body weight subcutaneously, and blood glucose values were measured via blood drops obtained by clipping the tail of the mice at 0, 15, 40, 90, 120 and 240 min after the insulin injection. The results of the ITT were also expressed as the percentage of the reduction of blood glucose value.
Blood sample analysis After 20 d treatment with the compounds, blood samples were collected via retro-orbital sinus in the fed and fasted mice, respectively. The samples were separated into serum, immediately frozen at -20 °C, and stored until measurement. Serum insulin, triglyceride and free fatty acid (FFA) values were measured using the Insulin RIA Kit (Shanghai Institute of Biological Products, Shanghai, China), Triglycerides Kit (Shanghai Institute of Biological Products, Shanghai, China) and NEFA Kit (Nanjing Jiancheng Bioengineering Institute, Nanjing, China) respectively.
Skeletal muscle and liver triglyceride measurement After 20 d treatment with the compounds, the mice that had fasted for 5 h were anesthetized with an intraperitoneal injection of sodium pentobarbital (40 mg/kg body weight). The gastrocnemius muscle and liver were removed and immediately frozen in liquid nitrogen, and then stored at -80 °C. The frozen skeletal muscle 15–20 mg or frozen liver 3–5 mg were used for triglyceride extraction. Each frozen tissue was added to 0.3 mL heptane-isopropanol-tween mixture (3:2:0.01 by volume) and homogenized. This homogenate was centrifuged at 1500×g at 4 °C for 15 min. Supernatants (upper phase contained extracted triglycerides) were collected and evaporated with vacuum centrifuge. The triglyceride content was determined using a triglyceride kit. All samples were measured in duplicates.
Statistics Results are expressed as mean±SD. Differences between groups were determined by one-way ANOVA. Fisher’s least significant differences post hoc analysis was used to identify significant differences (P<0.05). Differences between all time points with 0 min were analyzed by repeated ANOVA measurements in the OGTT and ITT experiments.
Results
Hypoglycemic effect of T33 on ob/ob mice The blood glucose level in the diabetic ob/ob mice was significantly higher than that of the lean mice. The ob/ob mice were treated with 4 mg/kg T33, 8 mg/kg T33, 4 mg/kg rosiglitazone or vehicle (1% CMC) for 20 d. After 4 d treatment, the significant decrease of the blood glucose level was observed in both the T33 and rosiglitazone-treated groups. T33 treatment led to dose-dependent reduction in blood glucose levels. The blood glucose levels of the mice that were fed and fasted decreased by 43.2% and 34.4%, respectively after 4 d treatment with 4 mg/kg T33, and the decrease rate of the 8 mg/kg T33-treated group was 57.6% and 39.7%, respectively (Tables 1, 2). During the 20 d treatment, the blood glucose levels of the 8 mg/kg T33-treated ob/ob mice that were fed and fasted were maintained at 4.5 to 7 mmol/L, which suggests that T33 could normalize the blood glucose of diabetic ob/ob mice. The hypoglycemic effect of T33 at a dose of 8 mg/kg was similar with that of rosiglitazone at a dose of 4 mg/kg on diabetic ob/ob mice (Tables 1, 2).
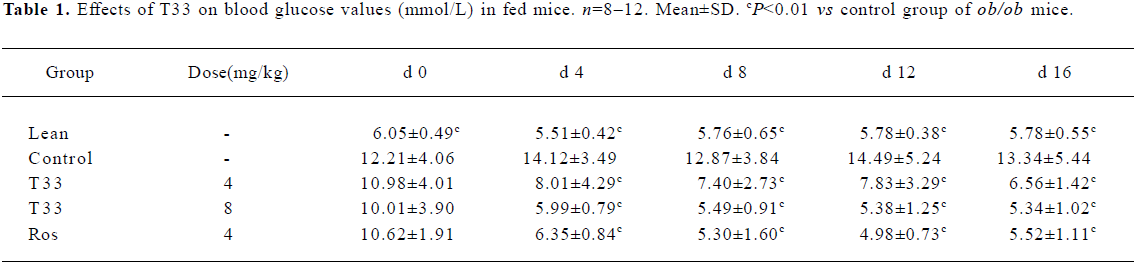
Full table
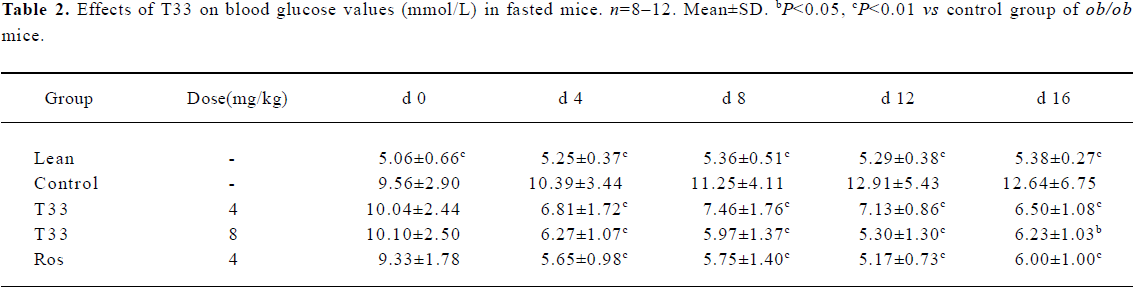
Full table
Insulin-sensitizing effects of T33 on ob/ob mice In the present study, the whole body insulin sensitivity was determined by performing OGTT and ITT. After 8 d treatment with the compounds, OGTT was performed. The ob/ob mice of the control group displayed a significantly stronger hyperglycemic response to an oral glucose administration, whereas the blood glucose level at each time point in both the 4 mg/kg and 8 mg/kg T33-treated ob/ob mice was lower than that of vehicle control (Figure 1A). The AUC in the T33-treated group also significantly decreased (Figure 1B). Moreover, the improvement of T33 on the impaired oral glucose tolerance on ob/ob mice was dose-dependent. Rosiglita-zone also improved the impaired oral glucose tolerance of ob/ob mice.
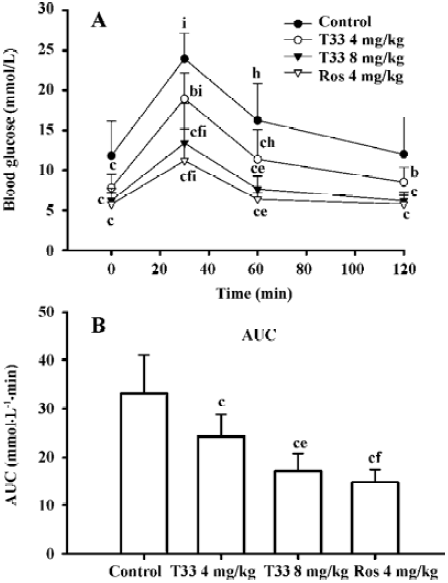
After 12 d treatment with T33 or rosiglitazone, ITT was performed in the ob/ob mice. No significant reduction of blood glucose level could be observed 15 min after the administration of insulin in the ob/ob mice of the vehicle control group, and only a 18.3% reduction could be observed at 40 min (Table 3), which suggests that ob/ob mice are insulin resistant. T33 at a dose of 4 mg/kg did not show any improvement of insulin tolerance, whereas the higher dose of T33 obviously improved insulin tolerance in the ob/ob mice. In the 8 mg/kg T33-treated group, the blood glucose value reduced by 28.9% and 44.5%, respectively at 15 and 40 min after the insulin injection, which suggests that T33 has a significant insulin sensitizing effect on ob/ob mice. Rosiglita-zone 4 mg/kg also showed potential effects in the ITT, which is similar with that of T33 at a dose of 8 mg/kg.
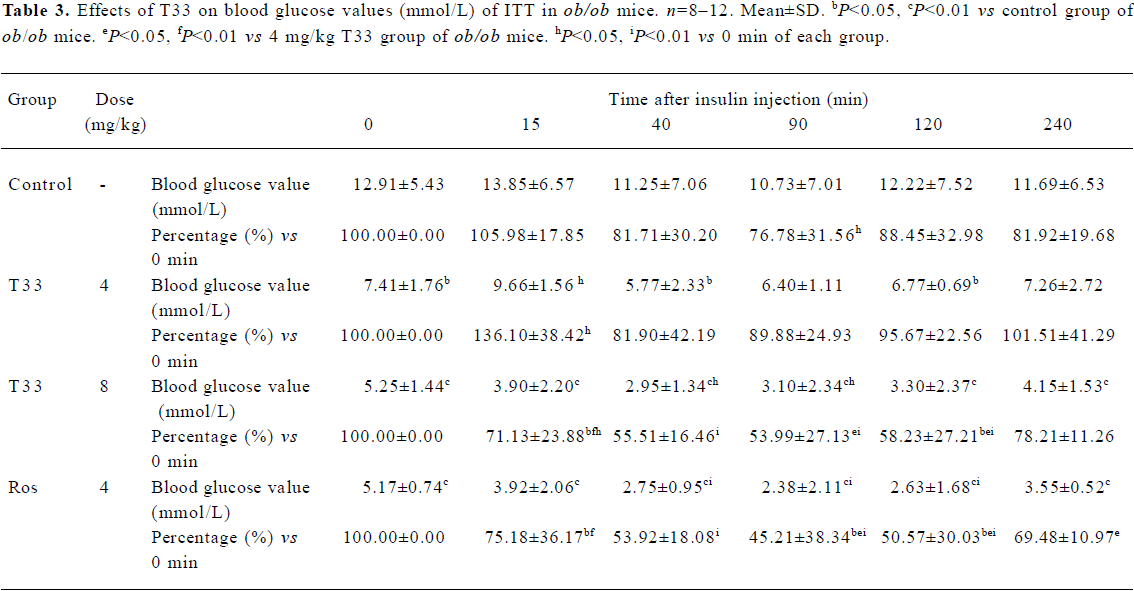
Full table
Effects of T33 on serum insulin, triglyceride, FFA and body weight of ob/ob mice Ob/ob mice are characterized by advanced hyperinsulinemia. Our data shows that the serum insulin concentration of the mice that were both fed and fasted was significantly higher than that of the lean mice (Figure 2A). Treatment with T33 at a dose of 8 mg/kg for 20 d decreased serum insulin levels significantly in the fasted mice (P<0.01), whereas no obvious reduction could be observed in the ob/ob mice treated by T33 at a dose of 4 mg/kg. Rosiglitazone treatment also led to a significant (P<0.05) reduction in serum insulin concentration in the fasted mice, whereas the reduction in the fed mice did not reach statistical difference.
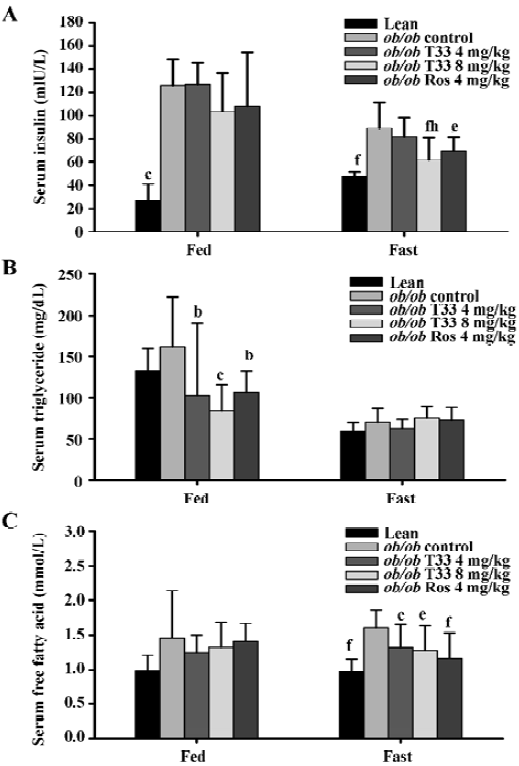
T33 treatment at a dose of 4 mg/kg and 8 mg/kg resulted in a 37% and 48% reduction in serum triglyceride concentration in the fed mice (P<0.05 and P<0.01 vs vehicle control mice respectively), whereas no significant reduction could be observed in the fasted mice (Figure 2B). Rosiglitazone treatment also reduced serum triglyceride level in the fed mice, but not in the fasted mice.
The ob/ob mice showed elevated FFA levels when compared with the lean mice (Figure 2C). After 20 d treatment with T33, the serum FFA levels in the ob/ob mice that were made to fast was significantly reduced as compared with that of the control mice(P<0.05). However, no significant decrease was observed in the fed mice (Figure 2C). Rosiglita-zone treatment led to a significant (P<0.05) reduction in serum FFA concentration in the fasted mice, but not in the fed mice.
During the whole treatment, an increase in body weight was observed in the ob/ob mice. No significant difference could be found between the control and T33 or rosiglitazone-treated groups, which suggests that there is no T33 treatment-related effect on the body weight of ob/ob mice (Table 4).
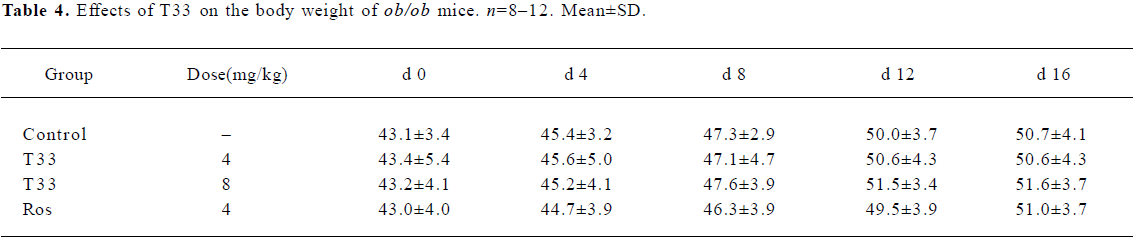
Full table
Effect of T33 on the triglyceride deposition in the skeletal muscle and liver To investigate the lipid deposition in the skeletal muscle and liver after T33 and rosiglitazone treatment in the ob/ob mice, the triglyceride content in the skeletal muscle and liver was evaluated. The triglyceride content in the skeletal muscle and liver of the ob/ob mice was much higher than that of the lean mice. After 20 d treatment with T33 at a dose of 8 mg/kg, the triglyceride content in the skeletal muscle of the ob/ob mice was reduced significantly (P<0.05; Figure 3A). In contrast with its effect on intramuscular lipid deposition, T33 did not have any effect on triglyceride content in the liver of the ob/ob mice. However, rosiglitazone at a dose of 4 mg/kg significantly increased the hepatocyte lipid deposition in the ob/ob mice after 20 d treatment (Figure 3B).
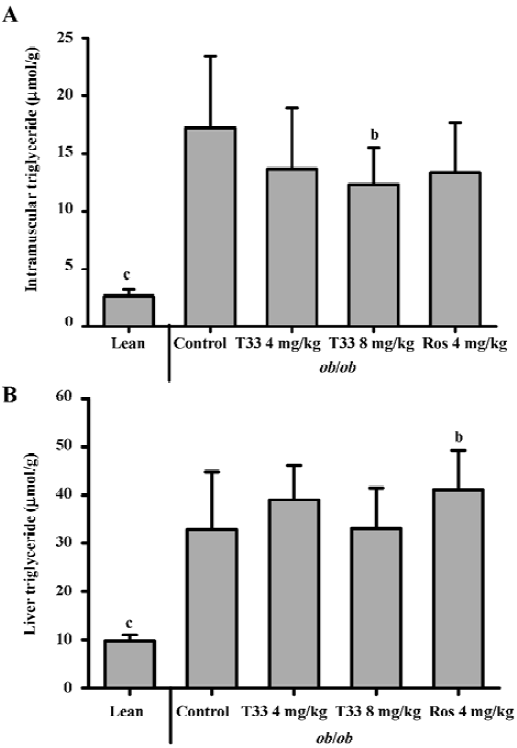
Discussion
Insulin resistance in type 2 diabetes is associated with both hyperglycemia and hyperlipidemia. PPAR are ligand-activated transcription factors, which offer a promising therapeutic approach for the treatment of different diseases. Fibrates and TZD used in the treatment of dyslipidemia and diabetes are ligands for PPARα and PPARγ, respectively. Therefore, a PPARγ/α dual agonist should facilitate better management of insulin resistance, hyperglycemia and hyperlipidemia of type 2 diabetes. T33, a benzopyran derivative with a different chemical structure to TZD, could increase the triglycerides accumulation in 3T3-L1 adipocytes and shows dual activation of γ and α isoforms of PPAR in a cell-based reporter gene assay[16]. In the present study, we evaluated the antidiabetic effects of T33 in ob/ob mice. As the most potent, efficacious, less toxic and marketed TZD derivative[10,17], rosiglitazone was chosen as the positive control in the present study. Since the ED50 value of the hypoglycemic effect of rosiglitzone in ob/ob mice had been reported to be 3.6 mg/kg[17], the dose of rosiglitazone was set at 4 mg/kg. The same, and a much higher dose of T33, were used to compare its effect with rosiglitazone.
Ob/ob mice, a model of severe obesity, insulin resistance, and diabetes caused by leptin deficiency[18], was the commonly used animal model for the evaluation of antidiabetic and insulin-sensitizing drugs. The ob/ob mice developed remarkable hyperinsulinemia and relatively mild hyperglycemia at the age of 6 weeks; the hyperglycemia could only be maintained until 14 weeks. In the present study, T33 showed dose-dependent reduction in blood glucose levels. T33 8 mg/kg reduced the blood glucose concentration to the normal level, which is similar to the hypoglycemic efficacy of rosiglitazone at a dose of 4 mg/kg. Among the PPARγ agonists in the market, rosiglitazone is claimed to be the most potent and efficacious. However, there were several PPARα/γ dual agonists which showed more potent hypoglycemic effects than rosiglitazone, although they were less potent in the activation of PPARγ[17,19]. Therefore, we speculate that the activation of PPARα of PPARα/γ dual agonists play an important role in their hypoglycemic effect.
The severely impaired glucose tolerance and insulin tolerance confirmed the insulin resistant state of the ob/ob mice. T33 treatment showed marked amelioration in oral glucose tolerance and insulin tolerance in the ob/ob mice, which suggests potent insulin-sensitizing properties of this compound. Compensatory hyperinsulinemia, another characteristic of type 2 diabetes, appears to contribute to the development of many other disorders such as dyslipidemia and hypertension. Ob/ob mice exhibit marked hyperinsulinemia, and the T33 treatment reduced the serum insulin concentration significantly in the fed mice and fasted mice, which also suggests the insulin-sensitizing effect of T33 on ob/ob mice. Therefore, all these results suggest that T33 could improve insulin resistance and increase the whole body insulin sensitivity.
The metabolic profile of type 2 diabetes included impaired glucose metabolism and insulin resistance, frequently combined with dyslipidemia[20]. In an insulin-resistant condition, lipolysis in the peripheral adipose tissue increased, enhancing FFA production and leading to a high plasma FFA level[2]. Many tissues, such as the liver and skeletal muscle, exposed on the high plasma FFA level, can cause and progress insulin resistance, since FFA can switch to TG accumulation in liver and skeletal muscles, which impacts insulin action[21]. In the present study, T33 exhibited potent lipid-lowering efficacy in the ob/ob mice. The lower FFA and TG levels in peripheral circulation may contribute to the insulin-sensitizing effect of T33.
Decreased plasma FFA oxidation and increased FFA flux from peripheral tissues to the liver contribute to hepatic steatosis, which is an important factor associated with insulin resistance[22]. Rosiglitazone had been found to produce moderate to severe fatty liver in rodents[23]. In the present study, the TG content in the liver of the ob/ob mice was markedly increased when compared with the lean mice. Treatment with rosiglitazone at a dose of 4 mg/kg could further increase hepatocyte lipid deposition, whereas T33 at the same dose as rosiglitazone produced a lower degree of hepatocyte lipid accumulation. Moreover, T33 at a dose of 8 mg/kg did not increase TG content in the liver, which suggests that T33 had an improved side effect profile compared to rosiglitazone.
The skeletal muscle and adipose tissues are 2 major peripheral tissues that account for whole body glucose utilization. Under insulin-stimulated conditions, approximately 80% of glucose disposal occurs in the skeletal muscle, whereas adipose tissues account for a smaller fraction of whole body glucose uptake[24,25]. Increasing evidences show that the accumulation of TG in skeletal muscles may contribute to the impaired insulin regulated metabolic actions, including glucose transport, glycogen synthesis and gene expression[26]. In the present study, the TG content in the skeletal muscle was significantly higher than that of the lean mice, which is consistent with the impaired glucose uptake in the soleus muscle of those mice (unpublished data). T33 treatment at a dose of 4 and 8 mg/kg for 20 d could significantly decrease the intramuscular TG in ob/ob mice, with rosiglitazone exhibiting similar effects. Our unpublished data show that the treatment of ob/ob mice for 20 d with T33 at a dose of 8 mg/kg could increase both basal and insulin-stimulated glucose uptake in the soleus muscle and increased insulin signaling in the EDL muscle. Therefore, T33 could reduce lipid deposit in the skeletal muscle, which might contribute to the insulin-sensitizing effect of this compound on the skeletal muscle.
In summary, the PPARγ/α dual agonist T33 exerts potent and efficacious hypoglycemic, hypolipidemic and insulin-sensitizing effects in ob/ob mice. It not only lowered lipids in peripheral circulation, but also reduced triglyceride deposit in the skeletal muscle. We believe that T33 is a promising antidiabetic compound that will be helpful for the treatment of type 2 diabetes. Further studies should be carried out to develop T33 as a novel therapy for type 2 diabetes.
References
- DeFronzo RA, Bondonna RC, Ferranini E. Pathogenesis of NIDDM: A balanced overview. Diabetes Care 1992;15:318-67.
- DeFronzo RA, Ferrannnini E. Insulin resistance: a multifaceted syndrome responsible for NIDDM, obesity, hypertension, dyslipidemia, and atherosclerotic cardiovascular disease. Diabetes Care 1991;14:173-94.
- Schoonjans K, Staels B, Auwerx J. The peroxisome proliferator activated receptors (PPARs) and then effects on lipid metabolism and adipocyte differentiation. Biochim Biophys Acta 1996;1302:93-109.
- Kliewer SA, Xu HE, Lambert MH, Willson TM. Peroxisome proliferator-activated receptor: from genes to physiology. Recent Prog Horm Res 2001;56:239-65.
- Schoonjans K, Peinado-Onsurbe J, Lefebvre AM, Heyman RA, Briggs M, Deeb S, et al. PPARalpha and PPARgamma activators direct a distinct tissue-specific transcriptional response via a PPRE in the lipoprotein lipase gene. EMBO J 1996;15:5336-48.
- Loviscach M, Rehman N, Carter L, Mudaliar S, Mohadeen P, Ciaraldi TP, et al. Distribution of peroxisome proliferators-activated receptors (PPARs) in human skeletal muscle and adipose tissue: relation to insulin action. Diabetologia 2000;43:304-11.
- Berger J, Moller DE. The mechanisms of action of PPARs. Annu Rev Med 2002;53:409-35.
- Guerre-Millo M, Gervois P, Raspe E, Madsen L, Poulain P, Derudas B, et al. Peroxisome proliferators-activated receptor alpha activators improve insulin sensitivity and reduce adiposity. J Biol Chem 2000;275:16638-42.
- Shearer BG, Hoekstra WJ. Recent advances in peroxisome proliferator-activated receptor science. Curr Med Chem 2003;10:267-80.
- Young PW, Cawthorne MA, Coyle PJ, Holder JC, Holman GD, Kozka IJ, et al. Repeat treatment of obese mice with BRL 49653, a new and potent insulin sensitizer, enhances insulin action in white adipocyte. Diabetes 1995;44:1087-92.
- Beeson M, Sajan MP, Dazon M, Grebenev D, Gomez-Daspet J, Miura A, et al. Activation of protein kinase C-zeta by insulin and phosphatidylinositol-3,4,5(PO4)3 is defective in muscle in type 2 diabetes and impaired glucose tolerance: Amelioration by rosiglitazone and exercise. Diabetes 2003;52:1926-34.
- Miyazaki Y, He H, Mandarino LJ, DeFronzo RA. Rosiglitazone improves downstream insulin receptor signaling in type 2 diabetic patients. Diabetes 2003;52:1943-50.
- Zierath JR, Ryder JW, Doebber T, Woods J, Wu M, Ventre J, et al. Role of skeletal muscle in thiazolidinedione insulin sensitizer (PPARgamma agonist) action. Endocrinology 1998;139:5034-41.
- Robins S, Collins D, Wittes J, Papademetriou V, Deedwania P, Schaefer E, et al. Relation of gemfibrozil treatment and lipid levels with major coronary events. VA-HIT: a randomized controlled trial. J Am Med Assoc 2001;285:1585-91.
- Diabetes Atherosclerosis Intervention Study Investigators. Effect of fenofibrate on progression of coronary artery disease in type 2 diabetes: The Diabetes Atherosclerosis Intervention Study, a randomised study. Lancet 2001;357:905-10.
- Tang L, Yu J, Leng Y, Feng Y, Yang Y, Ji R. Synthesis and insulin-sensitizing activity of a novel kind of benzopyran derivative. Bioorg Med Chem Lett 2003;13:3437-40.
- Chakrabarti R, Misra P, Vikramadithyan RK, Premkumar M, Hiriyan J, Datla SR, et al. Antidiabetic and hypolipidemic potential of DRF 2519-a dual activator of PPAR-alpha and PPAR-gamma. Eur J Pharmacol 2004;491:195-206.
- Haluzik M, Colombo C, Gavrilova O, Chua S, Wolf N, Chen M, et al. Genetic background (C57BL/6J versus FVB/N) strongly influences the severity of diabetes and insulin resistance in ob/ob mice. Endocrinology 2004;145:3258-64.
- Ljung B, Bamberg K, Dahllof B, Kjellstedt A, Oakes ND, Ostling J, et al. AZ242, a novel PPARα/γ agonist with beneficial effects on insulin resistance and carbohydrate and lipid metabolism in ob/ob mice and obese Zucker rats. J Lipid Res 2002;43:1855-63.
- Cha BS, Ciaraldi TP, Park KS, Carter L, Mudaliar SR, Henry RR. Impaired fatty acid metabolism in type 2 diabetic skeletal muscle cells is reversed by PPARgamma agonists. Am J Physiol Endocrinol Metab 2005;289:E151-9.
- Sinha S, Perdomo G, Brown NF, O’Doherty RM. Fatty acid-induced insulin resistance in l6 myotubes is prevented by inhibition of activation and nuclear localization of nuclear factor kappa B. J Biol Chem 2004;279:41294-301.
- Wiegman CH, Bandsma RH, Ouwens M, van der Sluijs FH, Havinga R, Boer T, et al. Hepatic VLDL production in ob/ob mice is not stimulated by massive de novo lipogenesis but is less sensitive to the suppressive effects of insulin. Diabetes 2003;52:1081-9.
- Chen X, Osborne MC, Rybczynski PJ, Zeck R, Yang M, Xu J, et al. Pharmacological profile of a novel, non-TZD PPARgamma agonist. Diabetes Obes Metab 2005;7:536-46.
- DeFronzo RA, Jacot E, Jequier E, Maeder E, Wahren J, Felber JP. The effect of insulin on the disposal of intravenvous glucose. Results from indirect calorimetry and hepatic and femoral venous catheterization. Diabetes 1981;30:1000-7.
- Marin P, Rebuffe-Scrive M, Smith U, Bjorntorp P. Glucose uptake in human adipose tissue. Metabolism 1987;36:1154-60.
- Kelley DE, Goodpaster BH, Storlein L. Muscle triglyceride and insulin resistance. Annu Rev Nutr 2002;22:325-46.