Angiopoietin-1 protects mesenchymal stem cells against serum deprivation and hypoxia-induced apoptosis through the PI3K/Akt pathway1
Introduction
Mesenchymal stem cells (MSC) possess multilineage transdifferentiation potential[1] as well as continual self-renewal ability. MSC have become highlight in cell therapy and tissue engineering. After transplantation into the myocardial infarction model in vivo, MSC are able to differentiate into endothelial cells, vascular smooth muscle cells, and cardiac-like myocytes[2]. Both animal and clinical studies have demonstrated that MSC are capable of ameliorating cardiac function after myocardial infarction[3-7]. However, MSC have failed to produce satisfactory therapeutic effects in some investigations[8,9], possibly due to poor cell viability after transplantation in vivo[10,11]. Therefore, a new method that can improve the survival of MSC needs to be developed.
Angiopoietin-1 (Ang1) was isolated as a ligand of Tie-2 receptor[12], which was first identified in endothelial cells[13,14]. A variety of studies have demonstrated that the Ang1/Tie-2 signaling system plays an important role in vessel growth, remodeling, maturation, and normal lymphangiogenesis [15]. Furthermore, it is a strong anti-apoptotic factor in endothelial cells[16,17], neurons[18], synovial cells[19], and cardiomyocytes [20]. It is not known whether Ang1 also has a similar anti-apoptotic effect on MSC to improve cell survival after transplantation. In this study, experiments were designed to determine the effect of Ang1 on MSC viability under serum deprivation (SD) and hypoxic conditions in vitro, and the possible mechanisms behind it.
Materials and methods
Recombinant human Ang1, Tie-2 receptor–Fc (Tie-2/Fc) fusion protein, monoclonal Tie-2 antibody, and the antiphospho-Tie-2 (Y992) antibody were obtained from R&D Systems (Minneapolis, MN, USA). Akt, phospho-Akt, Bcl-2, Bax, and cleaved caspase-9 and -3 antibodies were purchased from Cell Signaling Technology (Danvers, MA, USA). The In situ cell death detection kit, POD (Roche, Mannheim, Germany), wortmannin (Alexis Biochemicals, Lausen, Switzerland), Hoechst 33342, and 3-(4,5-dimethylthiazol-2-yl)-2,5-diphenyltetrazolium bromide (MTT; Sigma, St Louis, MO, USA) were also used in the study.
MSC isolation and expansion The MSC were isolated and harvested as previously described[21]. In brief, Sprague–Dawley rats were sacrificed in accordance with the methods approved by the Animal Care and Utilization Committee of Zhejiang University (Hangzhou, China), and bone marrow samples were collected from the femoral and tibial cavities with basal Dulbecco’s modified Eagle’s medium (DMEM; Gibco, Carlsbad, CA, USA). Subsequently, nucleated cells were separated with 1.073 g/mL Percoll solution (GE Healthcare Bio-Sciences, Piscataway, NJ, USA) and seeded into 50-cm2 flasks with DMEM supplemented with 10% fetal bovine serum (FBS) and antibiotics. The cultures were maintained at 37 °C in a 5% CO2 incubator. After 24 h, non-adherent cells were removed, and adherent cells were washed 3 times with phosphate-buffered saline (PBS). The culture medium was changed every 3–4 d, and the cells were subcultured when confluency approached approximately 80%. The spindle-shaped, adherent MSC were expanded and cultured no more than 5 passages before use.
The cells were determined by fluorescence-activating cell sorting (Beckman Coulter, Fullerton, CA, USA) analysis before the experiments, using directly conjugated antibodies against CD44 [fluorescein–isothiocyanate conjugated (FITC); Caltag, Carlsbad, CA, USA], CD45 (FITC, Caltag, USA), and CD90 (phycoerythrin-conjugated; Caltag, USA)[21].
SD/hypoxia and Ang1 treatment protocol A well-established MSC apoptotic model induced by SD and hypoxia in vitro was followed in this study[22]. Briefly, the MSC culture medium was removed and the cells were washed twice with PBS before being plated in serum-free DMEM containing the required treatment. Flasks were kept in a modular incubator chamber (Billups–Rothenberg, Del Mar, CA, USA) infused with mixed gas (95% N2 and 5% CO2) until the concentration of oxygen was lower than 0.5% at 37 °C.
Ang1 was reconstituted according to the manufacturer’s protocol. Concentrations of 12.5, 25, 50, 100, and 200 μg/L were selected for this study. The concentration of 50 μg/L was used to detect the protein expression after confirmation of its maximum effect on MSC apoptosis. The MSC were exposed to Ang1 for 24 h before the assessment of apoptosis and protein expression. To detect Akt phosphorylation, MSC were incubated in SD and a hypoxic environment for 24 h before being exposed to 50 μg/L Ang1 for 25 min.
Measurement of apoptosis
Morphological changes Hoechst33342 was used for the evaluation of chromosomal condensation and nuclear fragmentation. The cells were carefully washed with PBS and fixed in freshly prepared 4% paraformaldehyde for 30 min. The samples were exposed to 5 μg/mL Hoechst 33342 for 10 min at room temperature under dark conditions. Subsequently, all of the samples were observed under a phase contrast microscope and a fluorescence microscope. Apoptotic cells were characterized by morphological changes, such as cell shrinkage, nuclear condensation, and fragmentation.
Terminal deoxynucleotidyl transferase-mediated digoxigenin-dUTP nick-end labeling MSC apoptosis was also determined by terminal deoxynucleotidyl transferase-mediated-dUTP nick-end labeling (TUNEL) assays. TUNEL staining was performed mainly according to the manufacturer’s protocol with some modifications. Briefly, the MSC were carefully washed twice with PBS and fixed with freshly prepared 4% paraformaldehyde for 1 h at room temperature, followed by incubation with a blocking solution (3% H2O2 in methanol) for 30 min. The MSC were permeabilized with a freshly prepared solution containing 0.5% Triton X-100 in 0.1% sodium citrate for 1 h at 37 °C. The TUNEL reaction mixture (50 μL) was then added to the samples and incubation continued for another 1 h at 37 °C in a humidified atmosphere under dark conditions. Later, 50 μL of converter POD was added to the samples, followed by 30 min incubation at 37 °C. The chromogenic reaction was carried out with 3,3’-diaminobenzidine and terminated by the addition of tap water. Finally, the MSC were counterstained with hematoxylin and analyzed by a light microscope.
Cell survival assessment The MTT assay was used to evaluate cell viability. The MSC were cultured in 96-well tissue culture plates, and 1×104 cells were equally seeded into each well. MTT was added at a final concentration of 0.5 mg/mL after different treatments. Four hours later, the medium was removed and DMSO was applied to solubilize the purple formazan. The results were read with an ELISA reader at 570 nm.
Western blot analysis After the MSC were treated in different conditions for the required times, the cells were scraped from the flasks and harvested in 1.5 mL Eppendorf tubes. RIPA cell lysis buffer containing 50 mmol/L Tris (pH 7.4), 150 mmol/L NaCl, 1 mmol/L EDTA, 0.1% SDS, and 1% NP-40 (1 mmol/L phenylmethylsulfonyl fluoride and 10 mmol/L NaF were added before use) was applied to lyse the MSC. The tubes were vortexed until the cells were lysed completely, followed by centrifugation at 12 000×g for 30 min. The protein concentrations of the supernatant were determined by the Bio-Rad DC protein assay method (Bio-Rad, Hercules, CA, USA). All procedures were performed at 4 °C.
The proteins (30-100 μg) were then loaded onto SDS–polyacrylamide gels, separated according to the molecular weight, and subsequently, electrophoretically transferred onto polyvinylidene difluoride membranes using a Bio-Rad wet transfer system. After blockage with 0.1% Tween in Tris-buffered saline containing 5% milk at room temperature for 1 h, the membranes were incubated with specific primary antibodies by gentle agitation overnight at 4 °C. The conjugation of the primary antibodies with horseradish peroxidase-marked secondary antibodies was kept at room temperature for 1 h. Finally, bands were obtained by using an enhanced chemiluminescence detection system (Biological Industries, Beit Haemek, Israel) and were analyzed by Image-pro plus version 5.02 (Media Cybernetics, Bethesda, MD, USA).
Statistical analysis Data were expressed as mean±standard deviation and were analyzed with the commercially available statistical software package, SPSS 13.0 (SPSS, Chicago, IL, USA). One-way ANOVA test was performed, and post-hoc multiple comparisons were done with S-N-K. Statistical significance was defined as P<0.05.
Results
Ang1 protects MSC against apoptosis and cell death induced by SD and hypoxia Hoechst and TUNEL staining were used to evaluate the protective effect of Ang1 on MSC against SD and hypoxia-induced apoptosis. After 24 h of treatment, cell shrinkage and nuclear condensation were remarkably increased in the SD/hypoxia group. Surprisingly, Ang1 clearly exerted a protective effect on MSC, as demonstrated by morphological and nuclear changes (Figure 1A,1B). Ang1 reduced the apoptotic rate in a dose-dependent manner and peaked at the concentration of 50 μg/L (Figure 1C,2A,2B). However, the soluble Tie-2/Fc fusion protein, which acts as an inhibitor by sequestering Ang1 to block its function, abrogated its protective effect (Figure 1D,2C). Similarly, Ang1 increased MSC viability of during SD and hypoxia while Tie-2/Fc inhibited a positive effect (Figure 3).
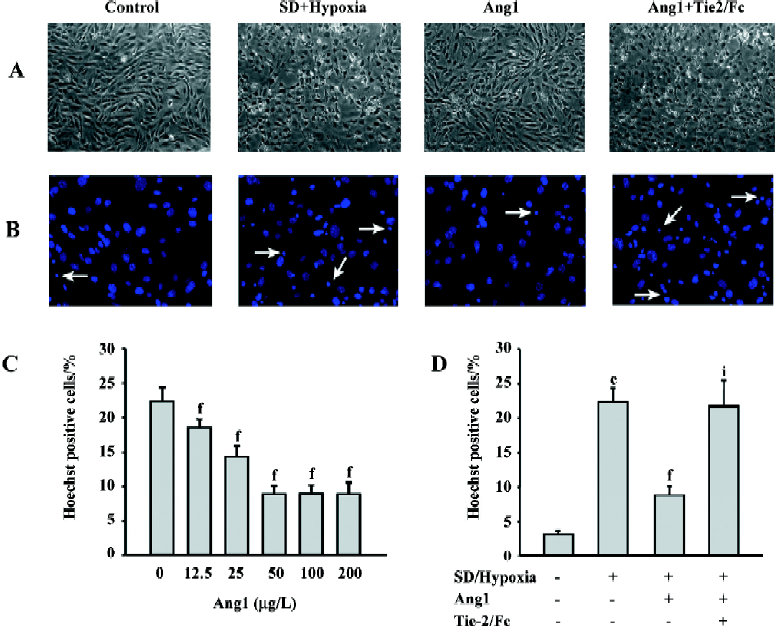
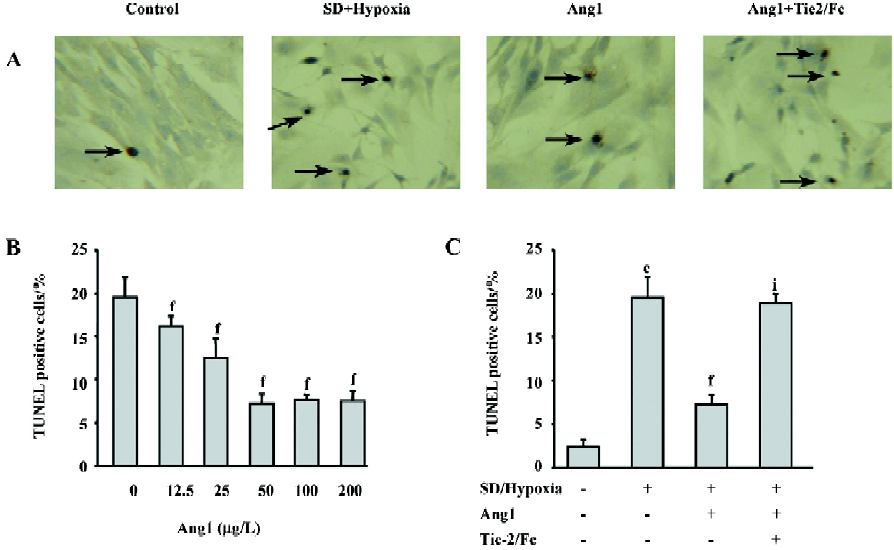
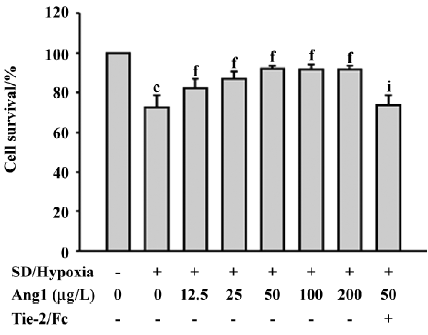
Ang1 induces Tie-2 receptor phosphorylation Western analysis demonstrated that the Tie-2 protein expression in MSC was slightly upregulated after SD and hypoxia insults, while the Ang1 application did not affect its level (Figure 4A). However, in the presence of Ang1, the phospho-Tie-2 expression of MSC was apparently increased, and the ratio of p-Tie-2/Tie-2 was critically increased in the Ang1 group as compared with the SD and hypoxia group (P<0.001). The ratio was strikingly decreased in the Ang1+Tie-2/Fc group (Figure 4B).
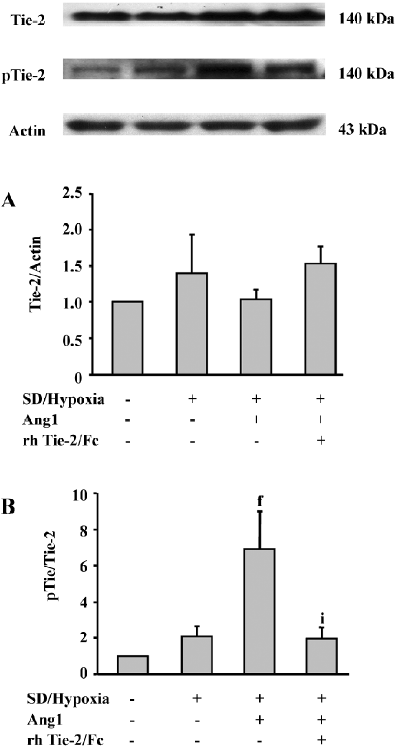
Ang1 activates Akt phosphorylation To evaluate downstream Ang1/Tie-2 signaling, the protein expressions in the phosphatidylinositol 3 kinase (PI3K)/Akt messenger pathway were examined by Western blot analysis. The data suggested that SD and hypoxia critically inhibited the phosphorylation of Akt (Ser473) in comparison with the control group, while exposure to Ang1 (50 μg/L) resulted in an increase in Akt phosphorylation. The ratio of p-Akt/Akt was obviously increased in the Ang1 group compared with the SD and hypoxia group. The presence of wortmannin (a selective inhibitor of PI3K) attenuated Akt phosphorylation induced by Ang1 (Figure 5).
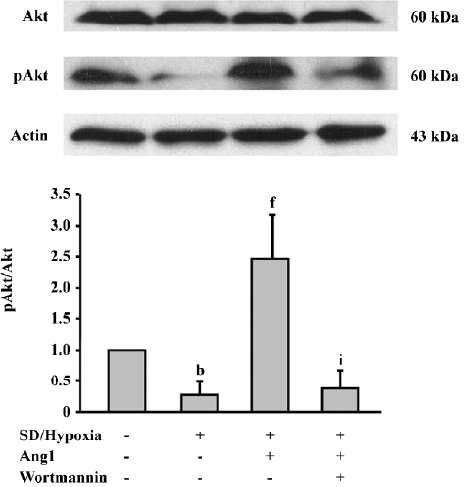
Ang1 increases the Bcl-2/Bax ratio Furthermore, we investigated the Bcl-2 family proteins to confirm the effect of SD and hypoxia and Ang1 on apoptosis-related proteins. Western blot analysis was performed and showed that SD and hypoxia decreased the ratio of Bcl-2/Bax, an important factor determining the susceptibility of apoptosis. The Bcl-2/Bax ratio increased by approximately 4-fold in response to Ang1 administration, whereas this effect was blocked by the inhibition of Ang1 and the PI3K/Akt pathway (Figure 6).
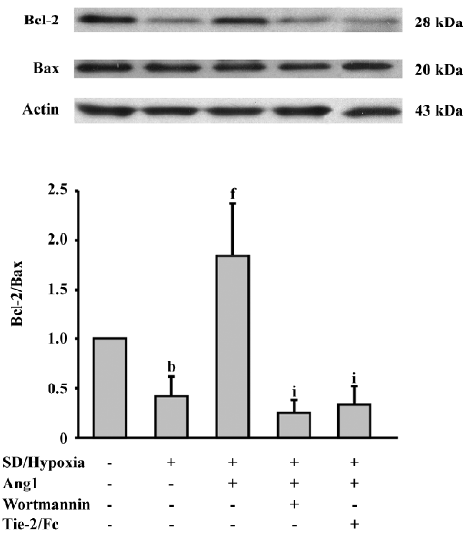
Ang1 decreases caspase-9 and -3 activation The results also illustrated the influence of Ang1 on SD and hypoxia-induced caspase activation. Almost no cleaved caspase-9 and -3 expressions were detected in the control group, while after SD and hypoxia treatment, both cleaved caspases were remarkably elicited. The intensities of these bands were attenuated when Ang1 was present in the culture medium. However, wortmannin, a specific PI3K inhibitor, and Tie-2/Fc reversed the inhibitory effect of Ang1 (Figure 7).
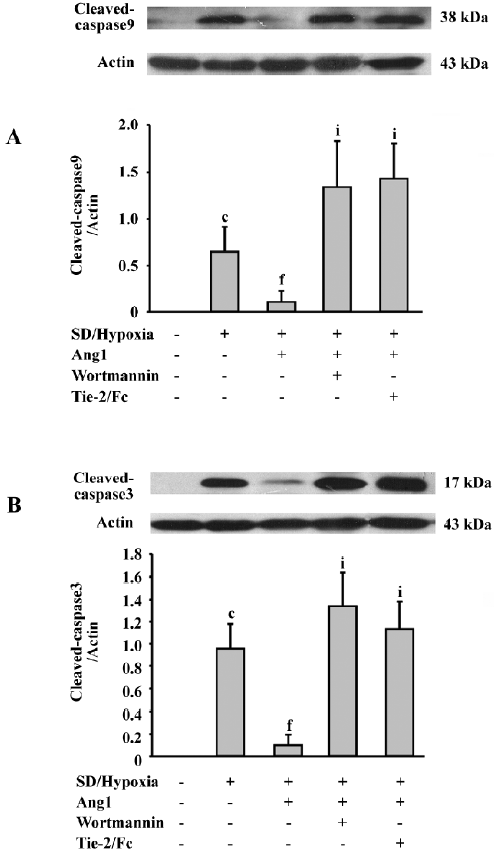
Discussion
In this study, we explored a new strategy to improve the survival of MSC in vitro and showed favorable results for the first time that Ang1 could improve MSC viability after SD and hypoxia-induced apoptosis. After exposure to Ang1, the Tie-2 protein became remarkably phosphorylated in MSC. Ang1 also induced the phosphorylation of Akt, increased the Bcl-2/Bax ratio, and decreased cleaved caspase-9 and -3 activation. Interestingly, all these effects were abrogated by Tie-2/Fc and wortmannin. Therefore, Ang1/Tie-2 and its downstream PI3K/Akt signal messenger pathway were involved in the mechanism of the Ang1 protection of MSC from SD and hypoxia-induced apoptosis.
Recent stem cell research in cardiology suggested that MSC are promising in regenerating the infracted myocardium[23]. However, most transplanted cells die within 4 d after transplantation to the ischemic heart [24]. Donor cell survival after transplantation remains among the major limitations that greatly influence the outcome of heart cell therapy. With emerging interest in gene modification, Ang1, Akt, and the HO-1 gene have been used to modify MSC. The engraftment of such modified MSC further improved cell survival and cardiac functions[24-26] . However, the safety of gene therapy has become an increasing public concern, and a variety of problems still remain[27,28]. In the present study, a much safer approach in the protein level and with potential clinical value was investigated.
The data used in the present study demonstrated the protective effect of Ang1 on MSC and showed the role of PI3K/Akt in Ang1/Tie-2 signaling, which was consistent with the observations on endothelial cells and neurons[18,29]. Akt has been shown to become phosphorylated to regulate the function of many cellular proteins involved in metabolism, survival/apoptosis, differentiation, and proliferation[30]. Increased Akt activity in genetically-modified MSC has been shown to protect cells against SD and hypoxia-induced apoptosis[26]. Consistent with previous reports, Ang1 promoted Akt phosphorylation and reduced MSC apoptosis in our study.
There are two major signaling pathways of apoptosis: the death receptor pathway and the mitochondrial pathway[31,32]. A recent study showed that SD and the hypoxia-induced apoptosis of MSC may not be affected by the death receptor pathway[22]. Therefore, Bcl-2, Bax, and caspase-9 and -3 levels were examined in this study to confirm the mitochondrial pathway, which may be involved in the regulation of apoptosis. The presence of an anti-apoptotic molecule, such as Bcl-2, can inhibit the activation of Bax following a death signal[33]. The ratio of Bcl-2/Bax is an important indicator of cell susceptibility to apoptosis[34,35]. Caspase-9 is activated by apoptosome, which is composed of cytochrome c, Apaf-1, and procaspase-9. It plays a critical role in the mitochondrial apoptosis pathway[32]. Caspase-3 has been demonstrated as a key mediator of mitochondrial events of apoptosis[36] and can be activated by SD and hypoxia in MSC[22]. In accordance with previous studies, the reduction of MSC apoptosis of by Ang1 administration correlated with an increase of the Bcl-2/Bax ratio and a decrease of caspase-9 and -3 activation in this study.
In conclusion, data in this study demonstrated that Ang1 protects MSC against SD and hypoxia-induced apoptosis. The results indicate that Ang1/Tie-2 signaling and its downstream PI3K/Akt messenger pathway are crucial for MSC survival, providing a promising approach for improving MSC viability.
References
- Pittenger MF, Mackay AM, Beck SC, Jaiswal RK, Douglas R, Mosca JD, et al. Multilineage potential of adult human mesenchymal stem cells. Science 1999;284:143-7.
- Orlic D, Kajstura J, Chimenti S, Jakoniuk I, Anderson SM, Li B, et al. Bone marrow cells regenerate infarcted myocardium. Nature 2001;410:701-5.
- Wollert KC, Meyer GP, Lotz J, Ringes-Lichtenberg S, Lippolt P, Breidenbach C, et al. Intracoronary autologous bone-marrow cell transfer after myocardial infarction: the BOOST randomised controlled clinical trial. Lancet 2004;364:141-8.
- Tomita S, Li RK, Weisel RD, Mickle DA, Kim EJ, Sakai T, et al. Autologous transplantation of bone marrow cells improves damaged heart function. Circulation 1999;100:II247-56.
- Hu X, Wang J, Chen J, Luo R, He A, Xie X, et al. Optimal temporal delivery of bone marrow mesenchymal stem cells in rats with myocardial infarction. Eur J Cardiothorac Surg 2007;31:438-43.
- Wang JA, Fan YQ, Li CL, He H, Sun Y, Lv BJ. Human bone marrow-derived mesenchymal stem cells transplanted into damaged rabbit heart to improve heart function. J Zhejiang Univ Sci B 2005;6:242-8.
- Wang JA, Li CL, Fan YQ, He H, Sun Y. Allograftic bone marrow-derived mesenchymal stem cells transplanted into heart infarcted model of rabbit to renovate infarcted heart. J Zhejiang Univ Sci 2004;5:1279-85.
- Kolossov E, Bostani T, Roell W, Breitbach M, Pillekamp F, Nygren JM, et al. Engraftment of engineered ES cell-derived cardiomyocytes but not BM cells restores contractile function to the infarcted myocardium. J Exp Med 2006;203:2315-27.
- Lunde K, Solheim S, Aakhus S, Arnesen H, Abdelnoor M, Egeland T, et al. Intracoronary injection of mononuclear bone marrow cells in acute myocardial infarction. N Engl J Med 2006;355:1199-209.
- Toma C, Pittenger MF, Cahill KS, Byrne BJ, Kessler PD. Human mesenchymal stem cells differentiate to a cardiomyocyte phenotype in the adult murine heart. Circulation 2002;105:93-8.
- Zhang M, Methot D, Poppa V, Fujio Y, Walsh K, Murry CE. Cardiomyocyte grafting for cardiac repair: graft cell death and anti-death strategies. J Mol Cell Cardiol 2001;33:907-21.
- Davis S, Aldrich TH, Jones PF, Acheson A, Compton DL, Jain V, et al. Isolation of angiopoietin-1, a ligand for the TIE2 receptor, by secretion-trap expression cloning. Cell 1996;87:1161-9.
- Dumont DJ, Yamaguchi TP, Conlon RA, Rossant J, Breitman ML. Tek, a novel tyrosine kinase gene located on mouse chromosome 4, is expressed in endothelial cells and their presumptive precursors. Oncogene 1992;7:1471-80.
- Maisonpierre PC, Goldfarb M, Yancopoulos GD, Gao G. Distinct rat genes with related profiles of expression define a TIE receptor tyrosine kinase family. Oncogene 1993;8:1631-7.
- Thurston G. Role of angiopoietins and Tie receptor tyrosine kinases in angiogenesis and lymphangiogenesis. Cell Tissue Res 2003;314:61-8.
- Papapetropoulos A, Garcia-Cardena G, Dengler TJ, Maisonpierre PC, Yancopoulos GD, Sessa WC. Direct actions of angiopoietin-1 on human endothelium: evidence for network stabilization, cell survival, and interaction with other angiogenic growth factors. Lab Invest 1999;79:213-23.
- Kwak HJ, So JN, Lee SJ, Kim I, Koh GY. Angiopoietin-1 is an apoptosis survival factor for endothelial cells. FEBS Lett 1999;448:249-53.
- Valable S, Bellail A, Lesne S, Liot G, Mackenzie ET, Vivien D, et al. Angiopoietin-1-induced PI3-kinase activation prevents neuronal apoptosis. FASEB J 2003;17:443-5.
- Hashiramoto A, Sakai C, Yoshida K, Tsumiyama K, Miura Y, Shiozawa K, et al. Angiopoietin 1 directly induces destruction of the rheumatoid joint by cooperative, but independent, signaling via ERK/MAPK and phosphatidylinositol 3-kinase/Akt. Arthritis Rheum 2007;56:2170-9.
- Wang Z, Cui M, Sun L, Jia Z, Bai Y, Ma K, et al. Angiopoietin-1 protects H9c2 cells from H2O2-induced apoptosis through AKT signaling. Biochem Biophys Res Commun 2007;359:685-90.
- Xie XJ, Wang JA, Cao J, Zhang X. Differentiation of bone marrow mesenchymal stem cells induced by myocardial medium under hypoxic conditions. Acta Pharmacol Sin 2006;27:1153-8.
- Zhu W, Chen J, Cong X, Hu S, Chen X. Hypoxia and serum deprivation-induced apoptosis in mesenchymal stem cells. Stem Cells 2006;24:416-25.
- Perin EC, Geng YJ, Willerson JT. Adult stem cell therapy in perspective. Circulation 2003;107:935-8.
- Tang YL, Tang Y, Zhang YC, Qian K, Shen L, Phillips MI. Improved graft mesenchymal stem cell survival in ischemic heart with a hypoxia-regulated heme oxygenase-1 vector. J Am Coll Cardiol 2005;46:1339-50.
- Jiang S, Haider H, Idris NM, Salim A, Ashraf M. Supportive interaction between cell survival signaling and angiocompetent factors enhances donor cell survival and promotes angiomyogenesis for cardiac repair. Circ Res 2006;99:776-84.
- Mangi AA, Noiseux N, Kong D, He H, Rezvani M, Ingwall JS, et al. Mesenchymal stem cells modified with Akt prevent remodeling and restore performance of infarcted hearts. Nat Med 2003;9:1195-201.
- Kaiser J. Clinical research. Death prompts a review of gene therapy vector. Science 2007;317:580.
- Kaiser J. Gene therapy. Side effects sideline hemophilia trial. Science 2004;304:1423-5.
- Kim I, Kim HG, So JN, Kim JH, Kwak HJ, Koh GY. Angiopoietin-1 regulates endothelial cell survival through the phosphatidylinositol 3'-kinase/Akt signal transduction pathway. Circ Res 2000;86:24-9.
- Fayard E, Tintignac LA, Baudry A, Hemmings BA. Protein kinase B/Akt at a glance. J Cell Sci 2005;118:5675-8.
- Ashkenazi A, Dixit VM. Death receptors: signaling and modulation. Science 1998;281:1305-8.
- Green DR, Reed JC. Mitochondria and apoptosis. Science 1998;281:1309-12.
- Gross A, Jockel J, Wei MC, Korsmeyer SJ. Enforced dimerization of BAX results in its translocation, mitochondrial dysfunction and apoptosis. EMBO J 1998;17:3878-85.
- Oltvai ZN, Milliman CL, Korsmeyer SJ. Bcl-2 heterodimerizes in vivo with a conserved homolog, Bax, that accelerates programmed cell death. Cell 1993;74:609-19.
- Yang E, Korsmeyer SJ. Molecular thanatopsis: a discourse on the BCL2 family and cell death. Blood 1996;88:386-401.
- Lakhani SA, Masud A, Kuida K, Porter GA Jr, Booth CJ, Mehal WZ, et al. Caspases 3 and 7: key mediators of mitochondrial events of apoptosis. Science 2006;311:847-51.