Mechanisms of antiprostate cancer by gum mastic: NF-κB signal as target1
Introduction
Gum mastic is a resinous exudation obtained from the stem and leaves of Pistacia lentiscus trees. It has been extensively used for centuries in Mediterranean and Middle Eastern countries, both as a dietary supplement and herbal remedy. Medical trials show that gum mastic may have cytoprotective or anti-acid effects on the gastrointestinal system, such as relief of ulcers and reducing the intensity of gastric mucosal damage caused by anti-ulcer drugs and aspirin with little or no side effects[1–4]. Recent studies seem to suggest that gum mastic may exhibit antibacterial properties[5,6]. In our previous studies, gum mastic has been shown to inhibit the proliferation of androgen-dependent prostate cancer LNCaP cells by inhibiting the androgen receptor (AR) function[7]. Here it is found that gum mastic also inhibits the growth of androgen-independent prostate cancer PC-3 cells. Moreover, gum mastic blocks PC-3 cell cycle progression. AR expression is absent in PC-3 cells, however, what factor promotes its growth and what mechanisms are involved in the inhibition of gum mastic on PC-3 cells growth? We will try to answer these questions in this study.
The nuclear factor κB (NF-κB) proteins are a family of transcription factors that regulate expression of genes involved in immune and inflammatory responses, cell growth, differentiation, and apoptosis[8]. NF-κB activity was shown to be constitutively activated in a series of androgen-independent prostate cancer cell lines and prostate carcinoma xenografts[9,10]. There was an inverse correlation between AR status and constitutive activation of NF-κB. The DNA binding activity of NF-κB in CL2 cells, androgen-independent cells derived from androgen-sensitive LNCaP cells, was found to be higher than in parental LNCaP cells[11]. These data suggest that constitutive activation of NF-κB may correlate with AR loss and may contribute to compensatory cellular changes allowing cell survival and growth in the absence of AR activation. To verify the crucial role of NF-κB in the androgen-independent prostate cancer PC-3 cells, we blocked NF-κB activity by specific inhibitor, BAY11-7082. The results showed that PC-3 cell growth was dramatically inhibited. It is speculated that NF-κB is a main factor in promoting PC-3 cell proliferation.
The importance of NF-κB in the development and progression of prostate cancer has recently become widely recognized. Numerous studies have shown that the suppression of constitutive NF-κB activation by certain herbal medicines or by genetic manipulation can inhibit growth, induce apoptosis, and enhance chemosensitization[12–14]. Agents capable of suppressing NF-κB activation are therefore anticipated to be potentially useful in the prevention or treatment of prostate cancer. The present study was undertaken to ascertain the inhibition by gum mastic on the PC-3 cells and to define the mechanisms involved in this inhibition, taking NF-κB and the NF-κB signal as targets.
Materials and methods
Cell culture and treatments The human prostate cancer cell line, PC-3, was obtained from the American Type Culture Collection (Manassas, VA, USA). The cells were seeded in 35 mmol culture dishes in RPMI-1640 medium supplemented with 10% fetal bovine serum (FBS) and 5% CO2 at 37 °C until reaching approximately 70%–80% confluence. The cells were treated with gum mastic at the indicated concentrations in RPMI-1640 medium containing 5% FBS (GIBCO BRL Grand Island, NY, USA) medium. Gum mastic (Sigma, St Louis, MO, USA, N
Cell proliferation assay For the 3-(4,5-dimethylthiazol-2-yl)-2,5-diphenyltetrazolium bromide (MTT) assay, the cells were cultured in 96-well culture plates at a density of 1000 cells/well with 200 µL culture medium. After 24 h incubation, gum mastic was added for 24 or 48 h. At the time of evaluation of cell growth, 20 µL MTT (final concentration, 0.5 mg/mL) was added into each well. After another 4 h of incubation, formazan crystals produced by living cultured cells were dissolved with 100 µL DMSO to measure the absorbance at 570 nm.
Cell cycle test PC-3 cells were plated in a 50 mL culture flask and treated with 30 µg/mL gum mastic or vehicle (0.1% DMSO) for 24 h. Then the cells were digested by trypsin and adjusted to the concentration of 1×106 cells/mL with PBS (137 mmol NaCl, 2.7 mmol KCl, 10 mmol Na2HPO4, and 2 mmol KH2PO4, pH 7.4). Based on the instructions of the CycleTEST PLUS DNA Reagent kit (Becton Dickinson, San Jose, CA, USA), 500 µL suspension cells were centrifuged at 800×g for 5 min at room temperature, then solution A (trypsin buffer), solution B (trypsin inhibitor and RNase buffer), solution C (propidium iodide stain solution) were added into the cells, respectively, for 10 min. Finally, the cells were analyzed using a FACScan flow cytometer (Becton Dickinson, Mountain View, CA, USA).
Nuclear extracts The cells were grown in the same conditions as described earlier and treated with gum mastic for 24 h. 2×109 cells were pelleted and resuspended in 1 mL cold hypotonic buffer (10 mmol/L HEPES, pH 7.9, 1.5 mmol/L MgCl2, 10 mmol/L KCl, 0.5 mmol/L DTT, and 0.5 mmol/L PMSF). Following 15 min incubation on ice, the cells were lysed by adding 50 µL of 10% NP-40 and centrifuged at 6000 ×g for 5 min at 4 °C. The pellets were resuspended in 70 µL cold hypertonic buffer (20 mmol/L HEPES, pH 7.9, 1.5 mmol/L MgCl2, 420 mmol/L NaCl, 0.2 mmol/L EDTA, 25% glycerol, 10 µg/mL aprotinin, and 0.5 mmol/L PMSF) and stirred gently at 4 °C for 30 min and centrifuged at 12 000×g for 5 min at 4 °C. The supernatant was dialyzed against the dialysis buffer (20 mmol/L HEPES, pH 7.9, 50 mmol/L KCl, 25% glycerol, 0.5 mmol/L DTT, and 0.5 mmol/L PMSF) for 2 h at 4 °C. The protein concentration of the dialyzed nuclear extracts was determined by the BCA method and stored at -80 °C in small aliquots.
Western blot analysis PC-3 cells, treated with gum mastic or 0.1% DMSO, were washed twice in cold PBS, lysed in whole cell lysis buffer (50 mmol Tris-Cl, pH 6.8, 10% sucrose, 2% SDS, and 5% β-mercaptoethanol) and harvested on ice for 30 min by cell scraping, and then centrifuged to get the whole extracts. For the Western blot analysis, 40 μg nuclear extracts or total extracts were separated on 10% SDS-PAGE and transferred to the nitrocellulose membrane. The nitrocellulose membrane was immediately blocked with 5% non-fat milk in PBS buffer for 1 h at room temperature. After blocking, the membrane was incubated with specific anti-NF-κB P65, anti-p-AKT, anti-cyclin D1, and anti-IκBα antibodies (Santa Cruz Biotechnology Inc, Santa Cruz, CA, USA) at 4 °C for 12 h, followed by the incubation with peroxidase-labeled second antibody for 1 h, and immunoreactive bands were visualized by enhanced chemiluminescence (ECL, Santa Cruz Biotechnology Inc, USA). β-actin (BD Biosciences, San Diego, CA, USA) was used to normalize the quantity of the protein on the blot.
RT-PCR analysis Total RNA was isolated from the treated cells by TRIzol reagent (Invitrogen, Carlsbad, CA, USA). Total RNA 2 μg were used to transcribe reversibly with the M-Mulv reverse transcriptase in the presence of random hexamer primer. The resulting cDNA preparation was subjected to PCR amplification using a PCR kit from TaKaRa Biotech (Dalian, China). The primers used for the cyclin D1 were as follows: sense 5'-GAAGTTGTTGGGGCTCCTCA-GGTT-3' and antisense 5'-CTGTCGCTGGAGCCCGTG-AAAAA-3', and for the housekeeping gene β-actin: sense 5'-GTGGGGCGCCCCAGGCACC-3' and antisense 5'-CTCCTT-AATGTCACGCACGATTT-3'. PCR conditions consisted of initial denaturation at 94 °C for 3 min and 28 cycles of amplification with denaturation at 94 °C for 40 s, primer-annealing at 60 °C for 40 s, and primer extension at 72 °C for 40 s. The final primer extension was performed at 72 °C for 10 min. The PCR products were analyzed by electrophoresis on a 1.5% agarose gel containing ethidium bromide and photographed under UV light.
Transient transfection assay The luciferase reporter plasmids containing a 5×NF-κB consensus binding motifs were described previously[15]. Twenty-four hours before transfection, 1×105 cells were plated in a 24-well plate. Cells were transfected using Lipofectamine 2000 reagent (Invi-trogen, Carlsbad, CA, USA) according to the manufacturer’s instructions. A pRL-TK plasmid was also included in the transfections for the internal control. Following the transfections, the cells were incubated with designated concentrations of gum mastic for an additional 10 h in RPMI-1640 medium containing 5% FBS, then the cell extracts were prepared for luciferase assays using the dual Luciferase Assay System (Promega, Madison, WI, USA). All transfection experiments were performed in triplicate and repeated at least 3 times.
Results
Gum mastic inhibited the proliferation of androgen-independent prostate cancer PC-3 cells PC-3 cells, which were hormone-refractory prostate cancer cells constitutive for NF- κB activation, were selected for these studies. First, we detected the effect of gum mastic on the PC-3 cells proliferation by MTT assay. The results in Figure 1 show that gum mastic inhibited proliferation in a concentration-dependent and time-dependent manner. Gum mastic 40 µg/mL inhibited the proliferation about 50% when incubated for 48 h.
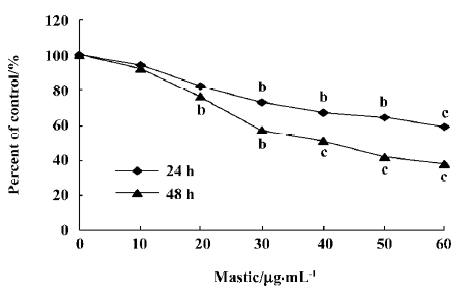
Gum mastic inhibited the cell cycle progression of PC-3 cells To further ascertain whether gum mastic affected the cell cycle of PC-3 cells, the cell cycle distributions of gum mastic-treated and vehicle-treated preparation were therefore analyzed by flow cytometry. As illustrated in Figure 2, the cells treated with gum mastic had larger populations of cells in the G1 phase and fewer populations of cells in the S phase, compared with the cells without gum mastic treatment.
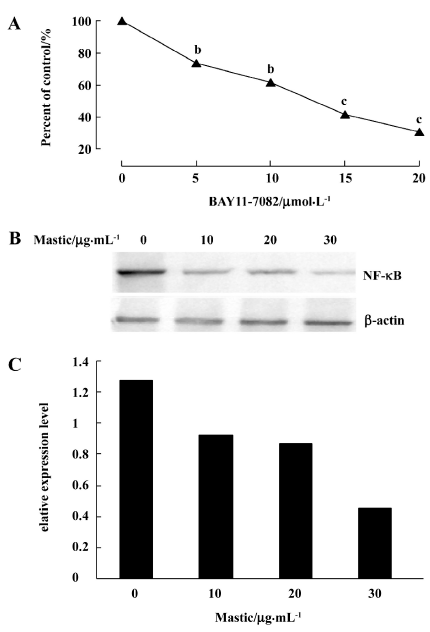
Gum mastic inhibited NF-κB expression NF-κB is known to be constitutively activated in PC-3 cells. When we blocked its expression by its specific inhibitor BAY11-7082, the PC-3 cells proliferation was inhibited dramatically by MTT assay (Figure 3A). It is speculated that NF-κB and the NF-κB signal are critical for PC-3 cell growth. To detect whether the inhibitory effect of gum mastic on PC-3 cells proliferation was due to the alteration of NF-κB expression, Western blotting was carried out to detect the change of the NF-κB protein level. Because NF-κB functions in the nucleus, nuclear extracts from treated and untreated PC-3 cells were used for the analysis. The results in Figure 3B show that gum mastic suppressed constitutive NF-κB expression in a dose-dependent manner.
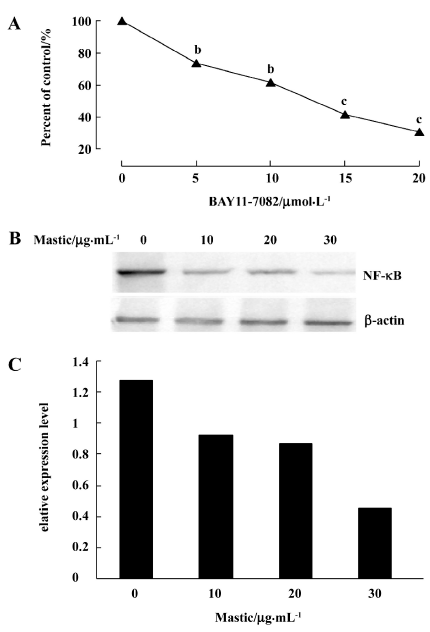
Gum mastic inhibited NF-κB binding to the κB consensus sequence NF-κB is a transcriptional factor and binds to the κB consensus sequence in the promoter of its target genes to regulate their expression. To study whether the NF-κB transcription function was affected by gum mastic, the reporter vector containing 5×NF-κB consensus binding motifs was used. Figure 4 shows that gum mastic inhibited the NF-κB binding to the κB consensus sequence.
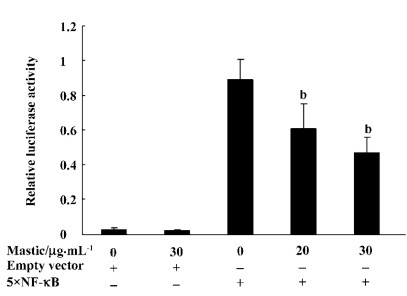
Gum mastic inhibited cyclin D1 expression Cyclin D1 is a typical NF-κB inducible gene whose promoter contains positive κB sequence for NF-κB binding[16]. RT-PCR was performed to determine whether the steady mRNA levels of cyclin D1 could be affected by gum mastic. It was found that gum mastic inhibited the cyclin D1 mRNA levels (Figure 5A). 30 µg/mL gum mastic decreased cyclin D1 mRNA levels about 50%. To further demonstrate the inhibitory effect of gum mastic on cyclin D1 protein levels, Western blotting was used. The results in Figure 5C show that the cyclin D1 protein levels were significantly decreased by gum mastic, which was consistent with the effect of gum mastic on cyclin D1 mRNA levels.
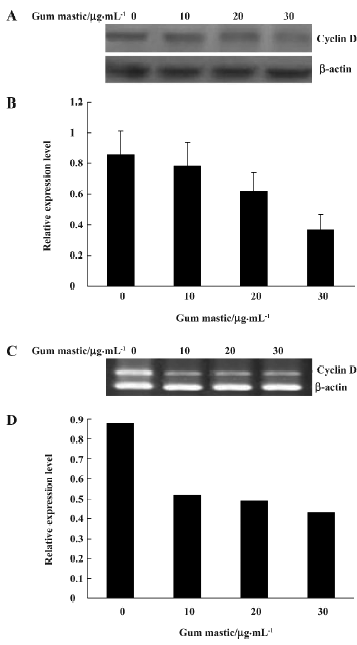
Gum mastic increased IκBα expression Translocation of NF-κB to the nucleus is normally regulated by IκBα degradation. We examined whether the inhibition of NF-κB activation by gum mastic was due to decreased degradation of IκBα. Western blotting for IκBα was performed as an index of total inhibitor protein levels. When the cells were treated with different concentrations of gum mastic for 24 h, significant dose-dependent increases in total IκBα protein levels were observed (Figure 6).
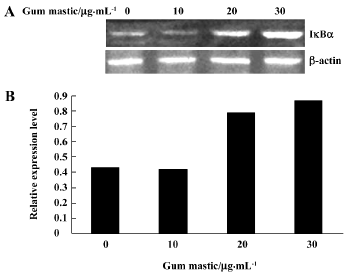
Gum mastic inhibited p-AKT expression To extend studies to the mechanisms involved in the gum mastic inhibition of NF-κB activity, the NF-κB upstream signal factor AKT protein levels were assayed by Western blotting. In prostate cancer cells, when AKT has been phosphorylated, it can activate I κ kinases (IKK) and finally activate NF-κB. So we detected the p-AKT protein levels with or without gum mastic treatment. It was found that gum mastic inhibited the p-AKT protein levels in PC-3 cells (Figure 7).
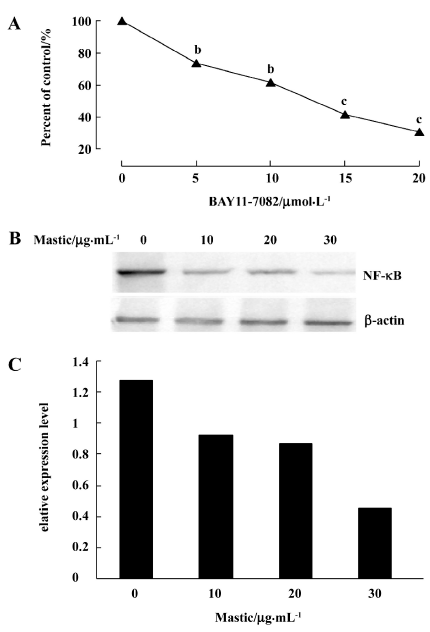
Discussion
Gum mastic was studied for the first time in our laboratory and found to possess antiprostate cancer properties. In this study, we first ascertained that gum mastic inhibited PC-3 cell growth and promoted PC-3 cell cycle arrest. Then we investigated the mechanisms involved in this regulatory system. As described in the Results section, gum mastic inhibited the NF-κB protein levels and the NF-κB signal pathway.
NF-κB expressed ubiquitously in various cell types and functioned as an important transcription factor governing many aspects of cellular and organismal physiology[8]. Mature NF-κB P65:P50 dimers are trapped in the cytoplasm of unstimulated cells by interaction with the inhibitory proteins termed IκBα. IKK phosphorylates IκBα proteins, thereby targeting them to rapid ubiquitin-dependent proteolysis that initiates the NF-κB activation. The activation of NF-κB leads to the expression of a large number of target genes. One of its target genes is cyclin D1, a cell cycle regulator, whose expression contributes to cellular proliferation[16]. One candidate kinase that may be involved in NF-κB activation in prostate cancer cells is PKB/AKT[17]. AKT can activate the NF-κB pathway via phosphorylation and activation of IKK in the NF-κB pathway[18,19]. Here gum mastic inhibited AKT expression and enhanced IκBα expression; this may resulted in its inhibition on NF-κB activity and NF-κB binding to κB sequence and cyclin D1 expression. The inhibitory effects of gum mastic on NF-κB and cyclin D1 finally lead to its induction of G1/S arrest and suppression of proliferation in PC-3 cells.
PC-3 cell growth is androgen-independent. Androgen and the androgen receptor play a critical role in the development of normal prostate and prostate cancer. Endocrine therapy for prostate cancer is aimed at reducing the level of circulating androgens or blocking the AR function. However, endocrine therapy is only palliative. Prostate cancer relapses generally occur within 1–2 years and become hormone refractory with a potentially fatal outcome[20]. How does this happen and how do prostate cells survive after androgen-ablation therapy?
AR can bind both to agonists, such as dihydrotesto-sterone and to selective androgen receptor modulators which act as antagonists or as weak agonists in a context-dependent fashion. Resistance to anti-androgen treatment has been postulated to reflect diverse mechanisms such as changes of AR functions, alterations of nuclear receptor cofactors, activation of growth factor or kinase pathways, and decreased expression of tumor suppressors or increased expression of anti-apoptotic genes[21–23]. Recently Zhu et al reported that the fundamental reason for androgen resistance is an increased monocyte/macrophage permeated in the prostate cancer cells and that these macrophages are a major localized source of the inflammatory cytokines that are linked to androgen resistance. Inflammatory cytokines triggered the signal pathway, which repressed the genes regulated by AR by the dismissal of the co-repressor complex[24].
On the other hand, inflammatory cytokines can stimulate NF-κB expression and function[25,26]. In fact, NF-κB over-expression was detected in androgen-independent prostate cancer cells in vitro and in vivo[9,10]. Accumulating evidence indicated that NF-κB is closely related to prostate cancer progression and chemotherapy resistance[27]. In contrast to androgen-dependent LNCaP, the androgen-independent prostate cancer cell lines, PC-3 and DU145, exhibit a high constitutive activation of IKK and subsequent NF-κB signaling[28]. Specific suppression of IKK expression dramatically inhibited the proliferation of PC-3 cells[29]. Taken together, it is speculated that NF-κB is the most important hallmark for the change from androgen-dependent to androgen-independent, and that NF-κB is necessary for the proliferation of androgen-independent prostate cancer cells. Therefore, NF-κB can be taken as a potential target for androgen-independent prostate cancer therapy.
Gum mastic inhibited NF-κB activity and the NF-κB signal pathway and has potential properties for treating prostate cancer. The main ingredients of gum mastic are mono-terpenes, including α-pinene (40%) and β-myrcene (9%), by gas chromatography mass spectrometry analysis[30]. Many monoterpenes, including α-pinene, may exhibit anticancer activities. We speculated that α-pinene is a main active ingredient in gum mastic, and further study will extract the active component from gum mastic and investigate its value to treat prostate cancer in vitro and in vivo. It was observed that boswellic acids extracted from other gum resins inhibited proliferation and induced apoptosis in PC-3 cells[29]. A hexane extract from gum mastic induced apoptosis in human colon cancer HCT116 cells[31]. Many agents inhibiting NF-κB activity also induced apoptosis[32,33]; however, we did not find the apoptosis in PC-3 cells treated with gum mastic by the flow cytometer assay. Gum mastic has little effect on the mRNA levels of bcl-xl, another NF-κB target gene which inhibits apoptosis (data not shown). In addition to the NF-κB pathway, further study will be required to investigate the cytotoxic mechanisms of gum mastic against prostate cancer.
References
- Al-Habbal MJ, Al-Habbal Z, Huwez FU. A double-blind controlled clinical trial of mastic and placebo in the treatment of duodenal ulcer. Clin Exp Pharmacol Physiol 1984;5:541-4.
- Huwez FU, Al-Habbal MJ. Mastic in treatment of benign gastric ulcers. Gastroenterol Jpn 1986;3:273-4.
- Marone P, Bono L, Leone E, Bona S, Carretto E, Perversi L. Bactericidal activity of Pistacia lentiscus mastic gum against Helicobacter pylori. J Chemother 2001;13:611-4.
- Al-Said MS, Ageel AM, Parmar NS, Tariq M. Evaluation of mastic, a crude drug obtained from Pistacia lentiscus for gastric and duodenal anti-ulcer activity. J Ethnopharmacol 1986;15:271-8.
- Andrikopoulos NK, Kaliora AC, Assimopoulou AN, Papapeorgiou VP. Biological activity of some naturally occurring resins, gums and pigments against in vitro LDL oxidation. Phytother Res 2003;5:501-7.
- Huwez FU, Thirlwell D, Cockayne A. Ala’Aldeen DA. Mastic gum kills Helicobacter pylori. N Engl J Med 1998;339:1946.
- He ML, Yuan HQ, Jiang AL, Gong AY, Chen WW, Zhang PJ, et al. Gum mastic inhibits the expression and function of the androgen receptor in prostate cancer cells. Cancer 2006;12:2547-55.
- Junghan S, Arnold BR. NF-κB activation in human prostate cancer: important mediator or epiphenomenon? J Cell Biochem 2004;91:100-17.
- Christopher S, Lang L, Rajasubramaniam S, Poornima BN, Vetrichelvan J, Lee AB, et al. Nuclear factor-κB is constitutively activated in prostate cancer in vitro and is overexpressed in prostatic intraepithelial neoplasia and adenocarcinoma of the prostate. Clin Cancer Res 2004;10:5501-7.
- Suh J, Payvandi F, Edelstein LC, Amenta PS, Zong WX, Celine G, et al. Mechanisms of constitutive NF-κB activation in human prostate cancer cells. Prostate 2002;52:183-200.
- Gasparian AV, Yao YJ, Kowalczyk D, Lyakh LA, Karseladze A, Slaga TJ, et al. The role of IKK in constitutive activation of NF-kappaB transcription factor in prostate carcinoma cells. J Cell Sci 2002;115:141-51.
- Raffoul JJ, Wang Y, Kucuk O, Forman JD, Sarkar FH, Hillman GG. Genistein inhibits radiation-induced activation of NF-kappaB in prostate cancer cells promoting apoptosis and G2/M cell cycle arrest. BMC Cancer 2006;6:107-15.
- Kwon O, Kim KA, Kim SO, Ha R, Oh WK, Kim MS, et al. NF-{kappa}B inhibition increases chemosensitivity to trichostatin A-induced cell death of Ki-Ras-transformed human prostate epithelial cells. Carcinogenesis 2006;27:2258-68.
- Greten FR, Eckmann L, Greten TF, Park JM, Li ZW, Egan LJ, et al. IKKb links inflammation and tumorigenesis in a mouse model of colitis-associated cancer. Cell 2004;118:285-96.
- Wang D, You Y, Case SM, McAllister-Lucas LM, Wang L, DiStefano PS, et al. A requirement for CARMA1in TCR-induced NF-kappa B activation. Nat Immunol 2002;3:830-5.
- Denis CG, Chris A, Julie YR, Richard GP, Albert SB. NF-κB controls cell growth and differentiation through transcriptional regulation of cyclin D1. Mol Cell Biol 1999;19:5785-99.
- Le Page C, Koumakpayi IH, Lessard L, Saad F, Mes-Masson AM. Independent role of phosphoinositol-3-kinase (PI3K) and casein kinase II (CK-2) in EGFR and Her-2-mediated constitutive NF-kappaB activation in prostate cancer cells. Prostate 2005;65:306-15.
- Ozes ON, Mayo LD, Gustin JA, Pfeffer SR, Pfeffer LM, Donner DB. NF-kappaB activation by tumour necrosis factor requires the Akt serine-threonine kinase. Nature 1999;401:82-5.
- Romashkova JA, Makarov SS. NF-kappaB is a target of AKT in anti-apoptotic PDGF signalling. Nature 1999;401:86-90.
- Denis LG, Griffiths K. Endocrine treatment in prostate cancer. Semin Surg Oncol 2000;18:52-74.
- Koivisto P, Kononen J, Palmberg C. Androgen receptor gene amplification: a possible molecular mechanim for androgen deprivation therapy failure in prostate cancer. Cancer Res 1997;57:314-9.
- Debes JD, Tindall DJ. Mechanisms of androgen-refractory prostate cancer. N Engl J Med 2004;351:1488-90.
- Chen CD, Welsbie DS, Tran C, Baek SH, Chen R, Vessella R, et al. Molecular determinants of resistance to antiandrogen therapy. Nat Med 2004;10:33-9.
- Zhu P, Baek SH, Bourk EM, Ohgi KA, Garcia-Bassets I, Sanjo H, et al. Macrophage/cancer cell interactions mediate hormone resistance by a nuclear receptor derepression pathway. Cell 2006;124:615-29.
- Buss H, Dorrie A, Schmitz ML, Hoffmann E, Resch K, Kracht M. Constitutive and IL-1-inducible phosphorylation of p65 NF-κB at serine 536 is mediated by multiple protein kinases including IκB kinase IKKα, IKKβ, IKKε, TBK1 and an unknown kinase and couples p65 to TAFII31-mediated IL-8 transcription. J Biol Chem 2004;279:55633-43.
- Kruppa G, Thoma B, Machleidt T, Wiegmann K, Kronke M. Inhibition of tumor necrosis factor (TNF)-mediated NF-kappa B activation by selective blockade of the human 55-kDa TNF receptor. J Immunol 1992;148:3152-7.
- Shukla S, Maclennan GT, Marengo SR, Resnick MI, Gupta S. Constitutive activation of PI3K-Akt and NF-kappaB during prostate cancer progression in autochthonous transgenic mouse model. Prostate 2005;64:224-39.
- Fradet V, Lessard L, Begin LR, Karakiewicz P, Masson AM, Saad F. Nuclear factor-kappaB nuclear localization is predictive of biochemical recurrence in patients with positive margin prostate cancer. Clin Cancer Res 2004;10:8460-4.
- Tatiana S, Jurgen EG, Berthold B, Yves L, Waltraud Z, Felicitas G, et al. Inhibition of IκB kinase activity by acetyl-boswellic acids promotes apoptosis in androgen-independent PC-3 prostate cancer cells in vitro and in vivo. J Biol Chem 2005;280:6170-80.
- Koutsoudaki C, Krsek M, Rodger A. Chemical composition and antibacterial activity of the essential oil and the gum of Pistacia lentiscus Var. chia. J Agric Food Chem 2005;53:7681-5.
- Balan KV, Demetzos C, Prince J, Dimas K, Cladaras M, Han Z, et al. Induction of apoptosis in human colon cancer HCT116 cells treated with an extract of the plant product, Chios mastic gum. In Vivo 2005;19:93-102.
- Huang YT, Pan SL, Guh JH, Chang YL, Lee FY, Kuo SC, et al. YC-1 suppresses constitutive nuclear factor-kappaB activation and induces apoptosis in human prostate cancer cells. Mol Cancer Ther 2005;4:1628-35.
- Shishir S, Hesham MA, Raymond L, Bharat BA. Curcumin (diferuloylmethane) inhibits constitutive NF-κB activation, induces G1/S arrest, suppresses proliferation, and induces apoptosis in mantle cell lymphoma. Biochem Pharmacol 2005;70:700-13.