Enhanced efficacy of CTLA-4 fusion anti-caries DNA vaccines in gnotobiotic hamsters1
Introduction
Dental caries remains one of the most common infectious diseases affecting humans in the world, and it is often at epidemic proportions, especially among the poor in developing countries[1]. For example, three-quarters of the 5-year-old children studied had evidence of significant dental decay, which was revealed by an oral health survey in China[2]. Caries immunization may be beneficial to those people in the future.
Streptococcus mutans (S. mutans) has been strongly implicated as a causative organism of dental caries[3,4]. Colonization of these microorganisms in tooth surfaces initiates the procedure of tooth decay. Two mechanisms, sucrose-independent and sucrose-dependent, are considered to mediate the colonization of S. mutans to tooth surfaces. A cell surface protein (PAc) is involved in the former mechanism, which mediates the initial adherence of S. mutans to the acquired pellicles on tooth surfaces[5,6]. The latter mechanism is due to the synthesis of water insoluble glucan from sucrose catalyzed by glucosyltrans-ferases (GTFs)[7,8]. Due to the importance of PAc and GTFs for cariogenicity of S. mutans, these proteins are rational candidate antigens in developing anti-caries vaccines.
Antibody responses are desirable to prevent dental caries. Antibodies against the recombinant protein containing the alanine-rich (A) region of PAc and the glucan binding (GLU) domain of GTF-I inhibit glucan synthesis, as well as the in vitro sucrose-independent and the sucrose-dependent adhesion of S. mutans to saliva-coated hydroxyapatite[9]. Passive immunization with milk containing antibodies against the A region of PAc and the GLU domain of GTF-I led to significantly less caries than the controls in rats[10]. We previously used porline-rich (P) region of PAc as a candidate for a potential DNA vaccine pGLUA–P, which encodes the GLU region of GTF-I and the A–P region of PAc. Rats immunized with pGLUA–P following subcutaneous injection near the submandibular gland developed significantly fewer caries lesions compared with the controls[11].
DNA vaccine is a promising new vaccine with many advantages over traditional vaccines, including its ease in preparation and administration, the ability to induce effective immune responses, as well as being a great potential for modification and improvement. The immune responses induced by DNA vaccines are initiated with the activation of antigen-presenting cells (APCs). Dendritic cells play a critical role in inducing immune responses of DNA vaccines[12,13]. Following genetic immunization, DNA vaccines can directly transfect local somatic cells in vivo. APCs can capture the antigens expressed by transfected cells, process, and then present them as major histocompatible complexes–peptide complexes to T cells in regional lymphoid organs where antigen-specific T cells are activated[14]. Cytotoxic T-lymphocyte-associated antigen 4 (CTLA-4) is a glycoprotein mainly expressed on activated T cells, and its extracellular V-domain has a strong binding affinity to B7 molecules (B7-1 and B7-2) primarily expressed on APCs[15–17]. A strategy of directing antigens directly to APCs by CTLA-4 was applied to enhance the efficacy of DNA vaccine. Vaccination with DNA encoding a model antigen (human Ig) and CTLA-4 have been shown to dramatically increase antibody responses compared with the control plasmid expressing the antigen alone[18].
To enhance the efficacy of anti-caries DNA vaccines, first we constructed CTLA-4 fusion DNA vaccine pGJA–P by cloning the signal peptide and extracellular regions of the human CTLA-4 gene and the hinge and Fc regions of the human Igγ1 gene into pGLUA–P[19]. Considering the possible application to clinical trials, the skeleton of pGJA–P was changed into the vector of pVAX1, which is especially designed for DNA vaccines, and then pGJA–P/VAX1 was constructed[20].
In this study, we cultured human dendritic cells (DCs), and investigated whether the CTLA-4–Ig–GLU–A–P fusion protein encoded by pGJA–P/VAX1 could specifically bind to human DCs. In addition, the immunogenicity and protective efficacy of pGJA–P/VAX1, pGJA–P, and pGLUA–P were compared via intramuscular (im) injection or intranasal (in) administration. Caries protection and anti-PAc-specific antibody responses were investigated in hamster experiments.
Materials and methods
Cells, animals, and plasmids COS-7 cells were generous gifts from Dr Hua TANG from the Institute of Immunology of the Secondary Military Medical University (Shanghai, China). The animals were purchased from Wuhan Institute of Biological Products (Wuhan, Hubei, China), and bred and maintained in the Hubei Medical Laboratory Animal Center (Wuhan, China). Five plasmids were used in the study: 2 CTLA-4 fusion anti-caries DNA vaccines (pGJA–P/VAX1 and pGJA–P), 1 non-fusion DNA construct (pGLUA–P), negative controls including pCI (Promega, Madison, WI, USA), and pVAX1 (Invitrogen, San Diego, CA, USA). As shown in Figure 1, pGLUA–P[11] encoded the GLU domain of the gtfB gene from S. mutans GS-5 and the A–P fragment of the Pac gene from S. mutans MT8148. pGJA–P[19] was constructed by inserting the signal peptide and extracellular regions of the human CTLA-4 gene and the hinge and Fc region of the human Igγ1 gene into pGLUA–P. The vector of pCI was the skeleton of pGLUA–P and pGJA–P. pGJA–P/VAX1[20] was obtained by cloning genes encoding the CTLA-4–Ig–GLU–A–P fusion protein from pGJA–P into the vector of pVAX1.
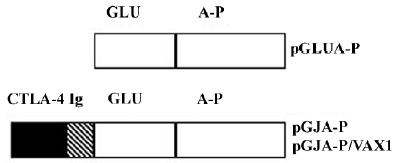
Plasmid preparation The plasmids were prepared with Endo-free plasmid mini kit II and maxi kit (Omega Biotek, Norcross, GA, USA). For immunization via in administration, DNA-bupivacaine complexes were prepared by adding bupivacaine hydrochloride (Sigma, St. Louis, MO, USA) to the aqueous DNA solution according to the fast mixing method[21]. The final complexes contained 0.25% bupivacaine.
Transfection and preparation of the fusion protein In the 6-well plates, the COS-7 cells were cultured at a concentration of 2×105 cells/well in RPMI-1640 (Gibco, Carlsbad, CA, USA) with 10% fetal calf serum (FCS, Gibco, USA). The cells were transfected with pGJA–P/VAX1 or pVAX1 using Sofast transfection reagent (Sunma Biotech, Xiamen, China) and cultured for 48 h before collecting the culture super-natant. The supernatant was concentrated by Amicon ultra-15 centrifuge filter devices (Millipore, Bedford, MA, USA), and stored at -70 oC.
The concentration of the fusion protein in the supernatant was determined by ELISA. Polyclonal goat anti-human IgG Ab (5 µg/mL, Southern Biotech, Birmingham, AL, USA) and peroxidase-conjugated goat anti-human IgG Ab (1:10,000, VectorLabs, Burlingame, CA, USA) were used to detect the fusion protein in the supernatant. Both were directed against the human Ig determinant in the fusion protein. Standard curves were generated by serially diluted human IgG (Sigma, USA) in the Ab-coated plates at a concentration range of 1.25–80 ng/mL.
Generation of human monocyte-derived DCs The monocytes were purified from human peripheral blood mononuclear cells by negative sorting using magnetic microbeads (Dynal, Oslo, Norway). The acquisition and treatment protocols of human blood were approved by Wuhan Blood Center. DCs were generated as described[22] by culturing the monocytes in complete medium supplemented with granulocyte–macrophage colony stimulating factor (GM–CSF, 50 ng/mL, R&D, Minneapolis, MN, USA) and interleukin (IL)-4 (50 ng/mL, R&D, USA). The complete medium included RPMI-1640, 1 mmol/L sodium pyruvate (Sigma, USA), 50 µmol/L 2-merca-ptothanol (Merck, West Point, PA, USA), 100 U/mL penicillin/ streptomycin, and 10% FCS. On d 5, the cells were stimulated by the addition of TNF-α (100 ng/mL, PeproTech, Rocky Hill, NJ, USA). The phenotype of the cells was assayed by flow cytometry. The Abs used for analysis were PE-conjugated anti-human CD14, anti-human CD80, anti-human CD86, and anti-human HLA-DR (eBioscience, San Diego, CA, USA). The isotype control used was PE-conjugated mouse IgG1 (eBioscience, USA).
Flow cytometric analysis of the fusion protein encoded by pGJA–P/VAX1 binding to human DCs Before the binding assay, the concentration of the fusion protein of the concentrated supernatant from pGJA–P/VAX1-transfected cells was determined by ELISA. It was equivalent to 0.22 µg/mL human IgG calibrated by the standard curve (R=0.998). There was no fusion protein that was detected in the supernatant from pVAX1-transfected cells.
On d 7, the DCs were harvested. The supernatant from pVAX1-transfected cells was used as the negative control to determine whether the fusion protein could bind to DCs. One group of DCs were blocked with B7-1 and B7-2 monoclonal antibodies (SBA, Birmingham, AL USA) to determine whether the binding activity was due to the interaction of CTLA-4 and B7 molecules on DCs. Three groups were divided as follows: group A (DCs vs pVAX1), group B (DCs vs pGJA–P/VAX1), and group C (B7-blocked DCs vs pGJA–P/VAX1). Briefly, the three groups of DCs were mixed with an equal volume (300 µL) of the concentrated supernatant, respectively, which were from either pGJA–P/VAX1-transfected cells or pVAX1-transfected cells. After being incubated for 1 h at 4 oC, the DCs were washed twice with binding buffer [phosphate buffered saline (PBS) plus 2% FCS]. Then FITC-conjugated goat anti-human IgG (Sigma, USA) was added (1:100 dilution in binding buffer) and incubated for 45 min at 4 oC. The DCs were washed and resuspended in 200 µL of 1% formaldehyde in PBS. The mean fluorescence intensities (MFIs) of the cells in the assay were analyzed under the same setting in a FACScan flow cytometer (Becton, Dickinson and Company, San Jose, CA, USA,) which had been calibrated with standard beads (Becton, Dickinson and Company, USA).
Animal immunizations The hamsters were divided into 12 groups, with 8 hamsters per group. Experimental hamster caries models were created as described[11]. Briefly, newborn, female golden hamsters were weaned on d 23 and raised on a cariogenic diet (Keyes 2000)[23]. Antibiotics (ampicillin, chloramphenicol, and carbenicillin, 1.0 g/kg diet) were added to the diet on d 24–26, and the hamsters were then orally challenged with 2×109 colony forming units (CFU) of S. mutans Ingbritt on d 28–30. Bacterial samples from the occlusal surfaces of each hamster were examined to make sure that all were infected with S. mutans.
After 3 d of S. mutans infection, the 12 groups of hamsters were immunized by quadriceps muscle injection or in administration. The immunization protocols were reviewed and approved by the review board of Hubei Medical Laboratory Animal Center. Six groups were immunized by im injection. Each hamster was injected into the quadriceps muscle of 1 leg with 100 µg plasmid in 100 µL saline solution under ether vapor anesthesia. The sham group was only injected with 100 µL/hamster saline solution. Six groups were immunized by in administration. Plasmid 100 µg in the solution of 50 µL DNA–bupivacaine complexes was deposited into both nostrils with the aid of a micropipette (25 µL/per nostril). The sham group was immunized with 50 µL/hamster saline solution containing 0.25% bupivacaine. The same procedure of immunization employing the same dose of immunogen was used for boost immunizations 2 weeks later. On d 70, serum and saliva samples were collected as described[24], and then hamsters were killed. The mandibles were removed, cleaned, and stained with murexide. The teeth were sectioned and caries levels were scored by the Keyes method[25].
Antibody analysis An ELISA was established to determine anti-PAc IgG in the serum and anti-PAc IgA in saliva samples. Each well of a polystyrene microtiter plate was coated with 100 µL rPAc (10 μg/mL, provided by Prof Takahiko OHO) in carbonate buffer (pH 9.6) and incubated overnight at 4 oC. Non-specific binding sites were blocked with 3% bovine serum albumin in phosphate-buffered saline containing 0.05% Tween 20 (PBST) for 2 h at room tempera-ture. Diluted sera or saliva (1:100 or 1:10 dilution, respectively, in blocking buffer) were added in triplicate to individual wells and incubated for 2 h at 37 oC. After washing with PBST, the amount of bound Abs was detected with peroxidase-conjugated goat anti-mouse IgG (1:2500, VectorLabs, USA) or peroxidase-conjugated goat anti-mouse IgA (1:1000, Sigma, USA) diluted in blocking buffer, followed by the addition of O-phenylenediamine substrate (40 mg/mL) in a solution containing 0.1 mol/L citric acid, 0.2 mol/L Na2HPO4, and 3% H2O2 to the plates. The reactions were quenched in 50 µL 2 mol/L sulfuric acid, and absorbance was measured at 490 nm. The background values were subtracted from the values of the experimental samples. The data were simplified by calculating the mean±SD of the absorbance of each sample, determined in triplicate.
Statistical analysis The statistical analysis of the ELISA antibody data and caries scores was performed with SPSS 10.0 software (SPSS Inc, Chincago, IL, USA) . Differences among the test and control groups were determined by ANOVA, followed by a multiple-mean comparison using Student–Newman–Keuls test. The value of P<0.05 was considered significant.
Results
Generation of human monocyte-derived DCs After 7 d culture with GM–CSF and IL-4, 70%–80% of the cells appeared as isolated or clustered floating cells with the typical dendritic morphology (Figure 2). An analysis of surface markers showed that the cells were homogeneous and expressed high levels of HLA-DR, CD80, and CD86, and had low level expressions of CD14 (Figure 3). The molecular profile of the cells is consistent with the results of Pickl et al[26] and Meierhoff et al[27].
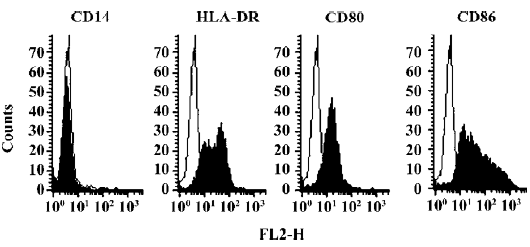
Binding of CTLA-4 fusion protein encoded by pGJA–P/VAX1 to human DCs In the assay, group A (DCs vs pVAX1) served as the negative control. The MFI of group A was 8.87 and 6.11% of the cells showed fluorescence. The MFI of group B (DCs vs pGJA–P/VAX1) was 12.09 and 12.69% of the cells showed fluorescence. The MFI of group C (blocked DCs vs pGJA–P/VAX1) was 7.68 and 2.29% of the cells showed fluorescence. As evidenced by an increased shift in fluorescence compared with negative control, the CTLA-4–Ig–GLU–A–P fusion protein encoded by pGJA–P/VAX1 was able to bind to human DCs (Figure 4). The specificity was confirmed by blockade of B7 molecules expressed on human DCs (Figure 4). The experiment was repeated 3 times.
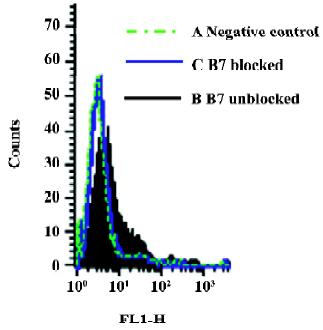
Specific anti-PAc antibody responses in hamsters The hamsters immunized with CTLA-4 fusion anti-caries DNA vaccines pGJA–P/VAX1, pGJA–P, and non-fusion DNA construct pGLUA–P showed significantly higher serum and salivary specific anti-PAc antibody responses than the negative controls and sham group (Figure 5). Furthermore, the hamsters immunized with pGJA–P/VAX1 and pGJA–P via the in route showed significantly higher serum and salivary anti-PAc-specific antibody responses than that of pGLUA–P (Figure 5). For immunization via the im route, serum IgG antibody responses induced by CTLA-4 fusion anti-caries DNA vaccines were significantly higher than that of non-fusion DNA construct pGLUA–P. There was no significant difference in anti-PAc antibody responses between the 2 CTLA-4 fusion anti-caries DNA vaccines.
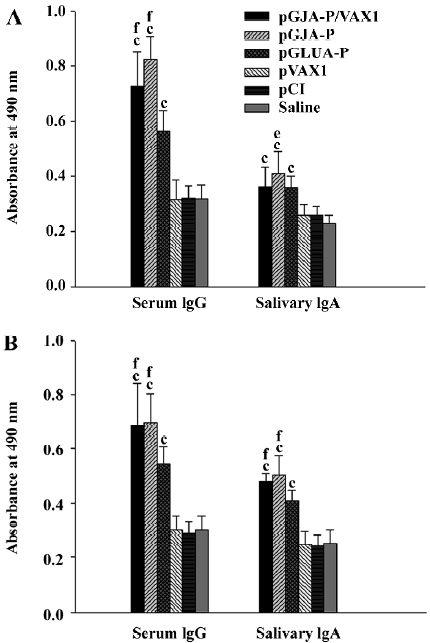
Caries protection pGJA–P/VAX1-, pGJA–P-, and pGLUA–P-immunized hamsters displayed significantly fewer enamel (E), dentinal slight (Ds), and dentinal moderate (Dm) lesions than that of the negative controls and sham group (Figure 6). pGJA–P/VAX1 and pGJA–P-immunized hamsters displayed significantly fewer E and Ds lesions than pGLUA–P–immunized hamsters (Figure 6). No significant difference in the caries score level was found between pGJA–P/VAX1 and pGJA–P-immunized hamsters.
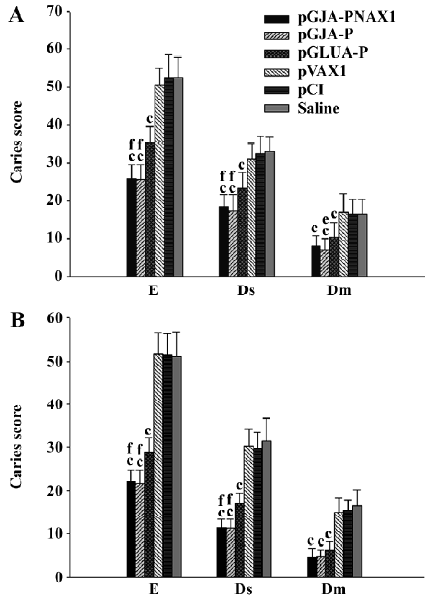
Discussion
Many studies have demonstrated that targeting an antigen as a CTLA-4 fusion protein to APCs can significantly enhance the magnitude of antibody responses[17,18,28]. We first employed the strategy to enhance the efficacy of anti-caries vaccines. It has been shown that the strategy is applicable to increase the magnitude of antibody responses against dental caries and significantly reduce the caries lesions. The biological activity of the CTLA-4 fusion protein is directly related with the efficacy of DNA vaccines or recombinant protein vaccines. Huang et al[17] have shown that mice immunized twice with Id–CTLA-4Y104A, a fusion protein with mutant CTLA-4 at residue 104 (Tyr→Ala) which loses binding activity to B7 molecules, induced only low titers of anti-Id Ab similar to the titers achieved by Id immuni-zation, whereas a single immunization with Id–CTLA-4 was able to produce significantly high titers of the anti-Id antibody. Deliyannis et al[28] demonstrated that the fusion protein of CTLA-4–hIg-hemagglutinin (HA) encoded by a HA-based influenza DNA vaccine was capable of binding to B7-NIT cells expressing membrane-bound B7-1 molecules. Mice receiving the targeted vaccine developed accelerated and increased antibody responses as compared with those receiving the nontargeted control. In this study, we chose DCs as the target cells and investigated the biological activity of the antigen encoded by CTLA-4 fusion DNA vaccines. The enhancement of immune response may be due to the targeting property of the CTLA-4 fusion protein. It has been hypothesized that directly targeting antigens to APCs through the interaction of CTLA-4 and B7 causes the antigen to be processed and presented to T cells with much higher efficiency, therefore leading to a stronger immune response[17]. T-independent B cells may also contribute to the enhanced immune responses. However, the mechanisms still deserve further investigation.
In the present study, we also provide evidence that pGJA–P/VAX1 was able to induce high levels of specific anti-PAc antibody responses comparable with the levels induced by DNA vaccine pGJA–P. Furthermore, significant inhibition of dental caries was also found in hamsters immunized with pGJA–P/VAX1. Investigators have doubted the effectiveness of pVAX1 as a DNA vaccine delivery vector for it failed to express substantial levels of protein compared with other mammalian expression vectors in vitro[29]. However, our study showed that the skeleton of the pVAX1 vector did not affect the potency of DNA vaccines in vivo. This will be helpful for the application of CTLA-4 fusion anti-caries DNA vaccines to be used by mankind in the future.
Salivary IgA is thought to be a key inhibitor of S. mutans infection[30]. Zhang et al[31] have reported that a chimeric protein SBR–GLU induced significant salivary anti-SBR and anti-GLU IgA responses after in immunization in mice, and that the inhibition of S. mutans colonization was in agreement with the salivary antibody responses. In that study, CTLA-4 fusion DNA vaccines pGJA–P/VAX1 or pGJA–P-immunized hamsters via the in route showed significantly higher levels of salivary anti-PAc IgA responses associated with fewer E, Ds, and Dm lesions than pGLUA–P-immunized groups. These results indicated that the degree of inhibition of dental caries may be intimately related to the levels of secretory IgA (S-IgA) responses. In the present study, the highest titer of S-IgA and the lowest caries scores were observed on hamsters immunized with CTLA-4 fusion anti-caries DNA vaccines via the in route. As a non-invasive delivery method, in delivery will be desirable for the application of anti-caries DNA vaccines.
The results from the hamster experiments may help us to learn more about the immune responses induced by DNA vaccine. The systemic immune and mucosal immune systems are 2 distinct compartments of the immune system. Antibodies associated with the systemic compartment are mainly of the IgG isotype. In contrast, antibodies in the mucosa are primarily S-IgA[32]. It has been generally regarded that im injection cannot induce effective mucosal immune responses. However, in the study, anti-caries DNA vaccines delivered via the im route induced significantly higher salivary specific anti-PAc IgA responses than the negative controls. We think this can be explained as follows: first, the secreted fusion antigen from transfected cells in local tissues was transferred by the circulating system to the mucosal immune system and captured by DCs to initiate the immune responses; and second, the immune responses may be initiated by antigen-loaded DCs migrated from the muscular tissue to the mucosal immune system. Enioutina et al[33] found that antigen-pulsed DCs injected into UVB-exposed peripheral skin sites were able to migrate to the Peyer’s patches and stimulate both mucosal and systemic immune responses. However, it needs further study to explore the precise mechanism.
In conclusion, we demonstrated that CTLA-4 fusion anti-caries DNA vaccines had good immunogenicity and potent capacities against caries in gnotobiotic hamsters. The efficacy of anti-caries DNA vaccine has been markedly improved by fusing antigen to CTLA-4, which directs the antigen to APCs.
References
- Smith DJ. Dental caries vaccines: prospect and content. Crit Rev Oral Biol Med 2002;13:335-49.
- Wong MC, Lo EC, Schwarz E, Zhang HE. Oral health status and oral health behaviors in Chinese children. J Dent Res 2001;80:1459-65.
- Hamada S, Slade HD. Biology, immunology, and cariogenicity of Streptococcus mutans. Microbiol Rev 1980;44:331-84.
- Loesche WJ. Role of Streptococcus mutans in human dental decay. Microbiol Rev 1986;50:353-80.
- Koga T, Okahashi N, Takahashi I, Kanamoto T, Asakawa H, Iwaki M. Surface hydrophobicity, adherence, and aggregation of cell surface protein antigen mutants of Streptococcus mutans serotype c. Infect Immun 1990;58:289-96.
- Bowen WH, Schilling K, Giertsen E, Pearson S, Lee SF, Bleiweis A, et al. Role of a cell surface–associated protein adherence and dental caries. Infec Immun 1991;59:4606-9.
- Kuramitsu HK, Smorawinska M, Nakano YJ, Shimamura A, Lis M. Analysis of glucan synthesis by Streptococcus mutans. Dev Biol Stand 1995;85:303-7.
- Monchois V, Willemot RM, Monsan P. Glucansucrases: mechanism of action and structure-function relationships. FEMS Microbiol Rev 1999;23:131-51.
- Yu H, Nakano Y, Yamashita Y, Oho T, Koga T. Effects of antibodies against cell surface protein antigen PAc-glucosyltransferase fusion proteins on glucan synthesis and cell adhesion of Streptococcus mutans. Infect Immun 1997;65:2292-8.
- Mitoma M, Oho T, Michibata N, Okano K, Nakano Y, Fukuyama M, et al. Passive immunization with bovine milk containing antibodies to a cell surface protein antigen-glucosyltransferase fusion protein protects rats against dental caries. Infect Immun 2002;70:2721-4.
- Guo JH, Jia R, Fan MW, Bian Z, Chen Z, Peng B. Construction and immunogenic characterization of a fusion anti-caries DNA vaccine against PAc and glucosyltransferase I of Streptococcus mutans. J Dent Res 2004;83:266-70.
- Fu TM, Ulmer JB, Caulfield MJ, Deck RR, Friedman A, Wang S, et al. Priming of cytotoxic T lymphocytes by DNA vaccines: requirement for professional antigen presenting cells and evidence for antigen transfer from myocytes. Mol Med 1997;3:362-71.
- You ZY, Huang X, Hester J, Toh HC, Chen SY. Targeting dendritic cells to enhance DNA vaccine potency. Cancer Res 2001;61:3704-11.
- Coombes BK, Mahony JB. Dendritic cell discoveries provide new insight into the cellular immunobiology of DNA vaccines. Immunol Lett 2001;78:103-11.
- Drew DR, Boyle JS, Lew AM, Lightowlers MW, Chaplin PJ, Strugnell RA. The comparative efficacy of CTLA-4 and L-selectin targeted DNA vaccines in mice and sheep. Vaccine 2001;19:4417-28.
- Linsley PS, Ledbetter JA. The role of the CD28 receptor during T cell responses to antigen. Annu Rev Immunol 1993;11:191-212.
- Huang TH, Wu PY, Lee CN, Huang HI, Hsieh SL, Kung J, et al. Enhanced antitumor immunity by fusion of CTLA-4 to a self tumor antigen. Blood 2000;96:3663-70.
- Boyle JS, Brady JL, Lew AM. Enhanced responses to a DNA vaccine encoding a fusion antigen that is directed to sites of immune induction. Nature 1998;392:408-11.
- Guo JH, Fan MW, Bian Z, Jia R, Chen Z, Peng B. Construction and expression in vitro of a targeted fusion anticaries DNA vaccine. Zhonghua Kouqiang Yixue Zazhi 2004;38:282-4. (in Chinese).
- Jia R, Guo JH, Fan MW, Bian Z, Chen Z, Fan B, et al. Immunogenicity of CTLA4 fusion anti-caries DNA vaccine in rabbits and monkeys. Vaccine 2006;24:5192-200.
- Pachuk CJ, Ciccarelli RB, Samuel M, Bayer ME, Troutman RD, Zurawski DV, et al. Characterization of a new class of DNA delivery complexes formed by the local anesthetic bupivacaine. Biochim Biophys Acta 2000;1468:20-30.
- Sallusto F, Lanzavecchia A. Efficient presentation of soluble antigen by cultured human dendritic cells is maintained by granulocyte/macrophage colony-stimulating factor plus interleukin 4 and downregulated by tumor necrosis factor α. J Exp Med 1994;179:1109-18.
- Navia JM. Animal models in dental research. Tuscaloosa, Alabama: University of Alabama Press; 1977.
- Fontana M, Dunipace AJ, Stookey GK, Gregory RL. Intranasal immunization against dental caries with a Streptococcus mutans-enriched fimbrial preparation. Clin Diagn Lab Immunol 1999;6:405-09.
- Keyes PH. Dental caries in the molar teeth of rats. A method for diagnosing and scoring several types of lesions simultaneously. J Dent Res 1958;37:1088-99.
- Pickl WF, Majdic O, Kohl P, Stockl J, Riedl E, Scheinecker C, et al. Molecular and functional characteristics of dendritic cells generated from highly purified CD14+ peripheral blood monocytes. J Immunol 1996;157:3850-9.
- Meierhoff G, Krause SW, Andreesen R. Comparative analysis of dendritic cells derived from blood monocytes or CD34+ hematopoietic progenitor cells. Immunobiology 1998;198:501-13.
- Deliyannis G, Boyle JS, Brady JL, Brown LE, Lew AM. A fusion DNA vaccine that targets antigen-presenting cells increases protection from viral challenge. Proc Natl Acad Sci USA 2000;97:6676-80.
- Locher CP, Witt SA, Ashlock BM, Levy JA. Enhancement of antibody responses to an HIV-2 DNA vaccine using an expression vector containing a constitutive transport element. DNA Cell Biol 2002;21:581-6.
- Russell MW, Hajishengallis G, Childers NK, Michalek SM. Secretory immunity in defense against cariogenic mutans streptococci. Caries Res 1999;33:4-15.
- Zhang P, Jespersgaard C, Lamberty-Mallory L, Katz J, Huang Y, Hajishengallis G, et al. Enhanced immunogenicity of a genetic chimeric protein consisting of two virulence antigens of Streptococcus mutans and protection against infection. Infect Immun 2002;70:6679-787.
- Lamm ME. Interaction of antigens and antibodies at mucosal surfaces. Annu Rev Microbiol 1997;51:311-40.
- Enioutina EY, Visic DM, Daynes RA. The induction of systemic and mucosal immunity to protein vaccines delivered through skin sites exposed to UVB. Vaccine 2002;20:2116-30.