11 beta-hydroxysteroid dehydrogenase type 1 promotes differentiation of 3T3-L1 preadipocyte1
Introduction
Obesity is reaching epidemic proportions and has significant health implications. Although many studies have focused on the role of 11 beta-hydroxysteroid dehydrogenase type 1 (11beta-HSD1) in the expansion of adipose tissue, the modulation of 11beta-HSD1 activity affects multiple target tissues, and may promote insulin resistance independent of obesity[1]. Examining the potential role of 11beta-HSD1 as a therapeutic target is an attractive research area for several reasons. 11beta-HSD1 knockout mice have low intracellular glucocorticoid levels, and are protected from obesity, diabetes, and dyslipidemia[2,3]. Conversely, the overexpression of 11beta-HSD1 in white adipose tissue results in elevated intracellular glucocorticoid levels, central obesity, insulin resistance, hypertension, hyperglycemia, and dyslipidemia in mice[4–6]. In vivo, 11beta-HSD1 catalyzes the conversion of glucocorticoids from the inactive to the active form. Glucocorticoids play an important role in normal physiology by modulating metabolic and immune responses[7]. Although it is now clear that glucocorticoids regulate the transcription of a diverse array of target genes, the precise mechanisms by which glucocorticoid receptor (GR)-mediated changes in cell proliferation and differentiation occur are still far from clear[8].
In this study, we explored the relationship between 11beta-HSD1 and preadipocyte differentiation in an in vitro 3T3-L1 preadipocyte model[9]. We constructed an 11beta-HSD1 siRNA vector and stably transfected 3T3-L1 cells to examine the effect of 11beta-HSD1 on adipocyte differentia-tion.
Materials and methods
3T3-L1 cell culture and induction of differentiation 3T3-L1 preadipocytes were cultured and induced to differentiate as previously described[10]. In short, the cells were cultured in Dulbecco’s modified Eagle’s medium (DMEM) (Gibco, Invitrogen Corporation, Carlsbad, CA, USA) containing 10% fetal bovine serum (FBS) in 5% CO2 at 37 °C for 2 d for confluence (d 0). Differentiation was induced by changing the medium to DMEM containing 10% FBS, 0.5 mmol/L 3-isobutyl-1-methyxanthine (Sigma, St Louis, MO, USA), 1 μg/mL insulin (Sigma, St Louis, MO, USA), and 1 mmol/L dexamethasone (Sigma, St Louis, MO, USA). After 48 h incubation (d 2), the medium was replaced with DMEM containing 10% FBS and 1 μg/mL insulin. On d 4, the medium was replaced with DMEM containing only 10% FBS, and then changed back to the same medium every 2 d for 4 d. The 3T3-L1 cells transfected with 11beta-HSD1 siRNA were cultured as above, with 200 μg/mL G418 (geneticin) (Sigma, St Louis, MO, USA).
Oil Red O staining and quantitative determination of triglyceride accumulation in cells The cells were stained with Oil Red O. Briefly, the cells were fixed with 10% formalin (pH 7.4), then stained with Oil Red O (stock solution: 3 mg/mL in isopropanol; working solution: 60% Oil Red O stock solution and 40% distilled water) for 5 min. The absorbance of the cell monolayers was spectrophotometrically determined at 510 nm after Oil Red O staining.
RNA preparation and real-time PCR Total RNA was isolated from cells using TRIzol (Invitrogen Corporation, Carlsbad, CA, USA). 1 mg total RNA was reverse transcribed using 200 U Moloney murine leukemia virus reverse transcriptase (Promega, Madison, WI, USA) in the presence of 0.5 mmol/L dNTP, 25 U RNase inhibitor, and 0.5 μg N6 random primers in a volume of 25 µL reaction buffer (Promega, Shanghai, China). Samples without reverse transcriptase treatment were also examined as controls for genomic DNA contamination. PCR primers (Table 1) were designed by Primer Design software Premier 5.0 (PREMIER Biosoft International, Palo Alto, CA,USA) using GenBank sequences and were synthesized by Invitrogen (Shanghai, China). Each real-time PCR reaction was carried out in triplicate in a total volume of 20 μL with Quanti Tect SYBR Green PCR Master Mix (MJ Research, Waltham, MA, USA) according to the following conditions: 5 min at 94 ºC, 44 cycles at 94 ºC for 30 s, 58 ºC for 30 s, and 72 ºC for 40 s using the ABI Prism 7700 sequence detection system (ABI, Oyster Bay, NY, USA). The Ct (cycle threshold) values (2Ct) for each gene were normalized to the expression levels of S18 (Ribosomal protein)(Table 1).
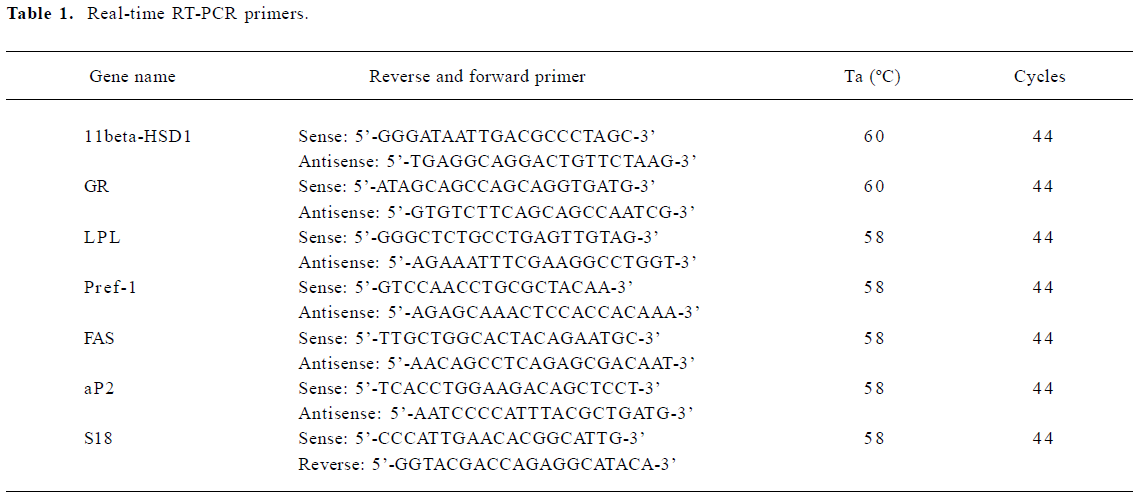
Full table
Construction of 11beta-HSD1 short interference siRNA expression plasmids BLAST 2 (Basic Local Alignment Search Tool) homology searching was used to identify 2 conserved cDNA fragments for RNA interference (RNAi) within the coding region of the mouse 11beta-HSD1 gene. The sequences were as follows:
sense: 5'-GAAGAGTCATGGAGGTCAA-3' and antisense:5'-AAAGCGAGGTGTACTATGA-3'.
From these sequences, we designed and synthesized 2 pairs of reverse complementary oligonucleotides, each containing a loop sequence (5'-TTCAAGAGA-3'), the RNA Pol III terminator (5'-TTTTTT-3') and 5' single-stranded overhangs for ligation into HindIII and BamHI-digested pGCsilencer H1/TetO1 RNAi vector (GeneChem, Shanghai, China). Oligonucleotides were annealed and inserted into the siRNA expression vectors to form pGCsilencer H1/TetO1–11beta-HSD1–1 and pGCsilencer H1/TetO1–11beta-HSD1–2, respectively. A control with limited homology to the human, mouse, and rat genome sequences was also created (GeneChem, Shanghai, China).
RNAi For stable expression, 2 mg each of the lipopolysaccharide-free vectors were transfected into 3T3-L1 cells(50% confluence) with Fugene 6 (Roche, Shanghai, China). The 3T3-L1 cells were seeded into 6-well culture plates with complete culture medium. The ratio of Fugene 6 to the vectors for transfection was 6:1. Two days after transfection, GFP (Green fluorescent protein) expression was observed. G418 was used for selection by adjusting the concentration of G418 from 400 to 800 µg/mL every 2 d, maintaining 800 µg/mL for 1 week. Non-transfected 3T3-L1 cells were used as a control for G418 sensitivity. G418-resistant cells were maintained in culture medium supplemented with 200 μg/mL G418 for further analysis.
Western blot analysis Non-transfected and transfected 3T3-L1 cells were collected, and the total proteins were isolated using the TRIzol method. The protein concentration was determined by BCA assay (Pierce, Rockford, IL, USA). The total proteins (10 μg per lane) were subjected to 12% SDS-PAGE and transferred onto PROTRAN nitrocellulose membranes (Schleicher and Schuell, Dassel, Germany), then immunoprobed with rabbit anti-human primary antibody against 11beta-HSD1 (1:200; Santa Cruz Biotechnology, Santa Cruz, CA, USA) and horseradish peroxidase-conjugated goat anti-rabbit IgG as the secondary antibody (1:800; Santa Cruz Biotechnology, Santa Cruz, USA). Immune bands were visualized by applying an ECL Western blot analysis system according to the manufac-turer’s instructions (Amersham, Amersham Pharmacia, Piscataway, NJ, USA). The antibody against beta-actin, which detects the expression of beta-actin, was used as an internal control (Santa Cruz, USA). Exposed film was then analyzed using a densitometer (Furi, Shanghai, China) to determine the optical densities of each band, and the density ratio of 11beta-HSD1 to beta-actin bands was calculated.
Statistical analysis All results are expressed as mean±SD. Data were analyzed using one-way ANOVA with a correction for multiple comparisons as appropriate.
Results
Characterization of 11beta-HSD1 stably transfected cells To further investigate the role of 11beta-HSD1 on adipocyte differentiation, transfection of the pGCsilencer H1/TetO1–11beta-HSD1–2 vector remarkably downregulated the transcription and expression level of mouse 11beta-HSD1, while no significant differences were detected in the cells transfected with pGCsilencer H1/TetO1–11beta-HSD1–1 (Figure 1).
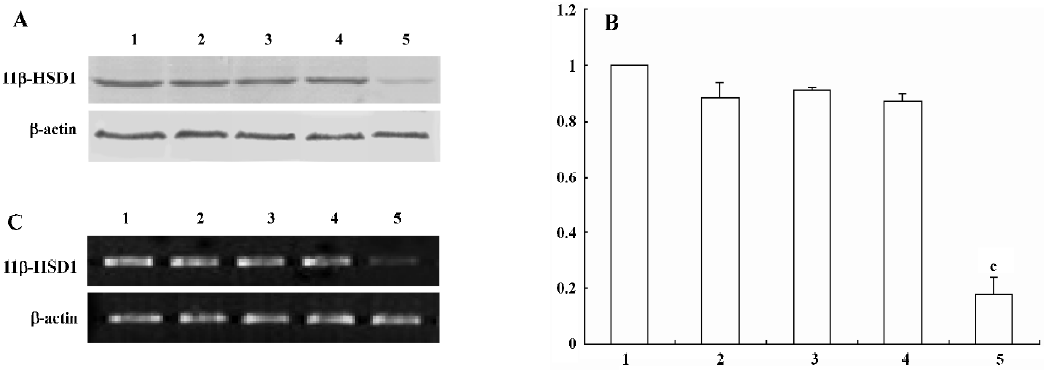
3T3-L1 preadipocyte as an in vitro differentiated model 3T3-L1 preadipocyte cells can be stimulated to differentiate into adipocytes. Differentiation was induced to the normal and transfected 3T3-L1 cells to examine the role of 11beta-HSD1 in adipocyte differentiation. On d 0, 2, 4, 6, and 8 of 3T3-L1 cell differentiation, Oil Red O staining was used to detect lipid droplets, which are indicative of adipocyte differentiation. Lipid droplets accumulated after stimulation (d 0) and the degree of lipid droplet accumulation increased over time. However, lipid accumulation was significantly inhibited in cells transfected with mouse 11beta-HSD1 siRNA compared with non-transfected 3T3-L1 cells and cells transfected with pGCsilencer H1/TetO1 (Figure 2A). The triglyceride content in the cells was measured by the absorbance of the cell monolayers, which was spectrophotometrically determined at 510 nm after Oil Red O staining. The triglyceride decreased in the cells transfected with 11beta-HSD1 siRNA (Figure 2B). These results suggest that 11beta-HSD1 is normally required for 3T3-L1 preadipocyte differentiation.
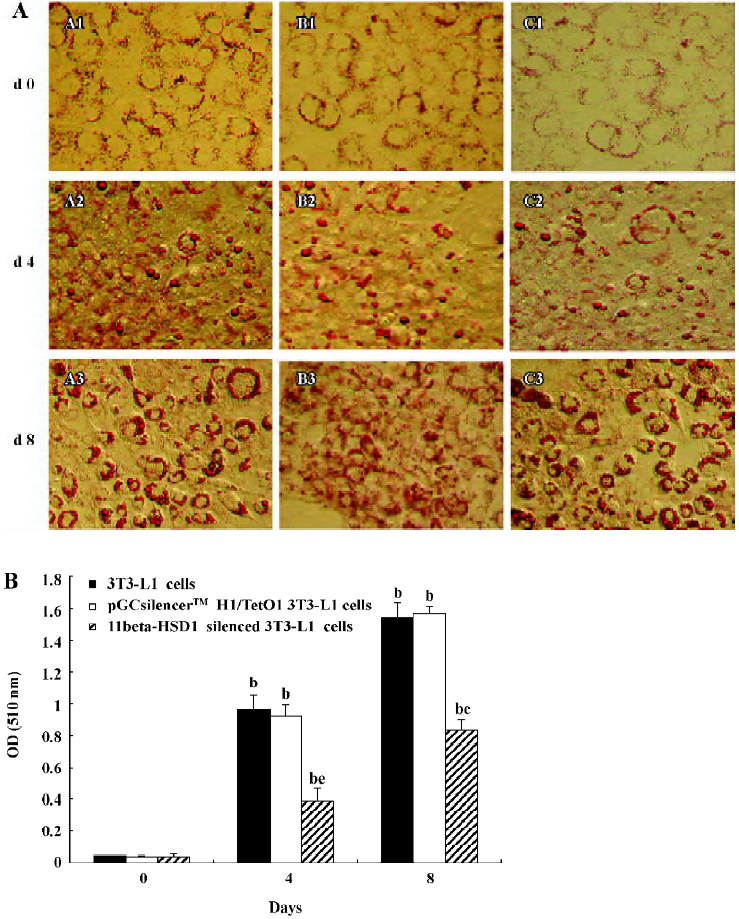
Expression of 11beta-HSD1 protein During adipocyte differentiation (d 0, 2, 4, 6, and 8), the expression of the 11beta-HSD1 protein was assessed by Western blot analysis. A major band migrating at 34 kDa, corresponding to the 11beta-HSD1 protein, was confirmed to be present in the 3T3-L1 cells. 11beta-HSD1 protein expression was upregulated during the course of adipocyte differentiation (d 8 vs d 0, P<0.01; Figure 3).
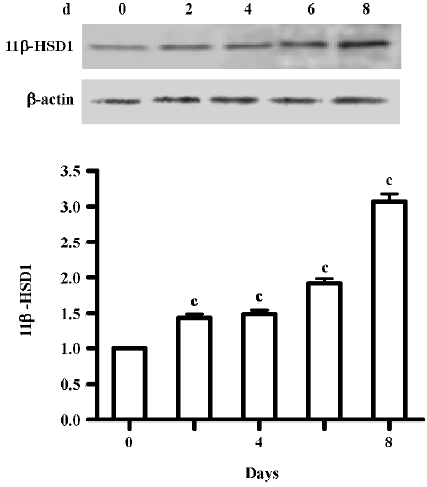
mRNA expression of GR GR mRNA was detected by real-time PCR from both non-transfected 3T3-L1 cells and 11beta-HSD1 siRNA-transfected 3T3-L1 cells during the course of adipocyte differentiation (d 0, 2, 4, 6, and 8). GR expression was upregulated on d 4 and 6, but was rapidly downregulated on d 8 in the control and siRNA-transfected 3T3-L1 cells (Figure 4).
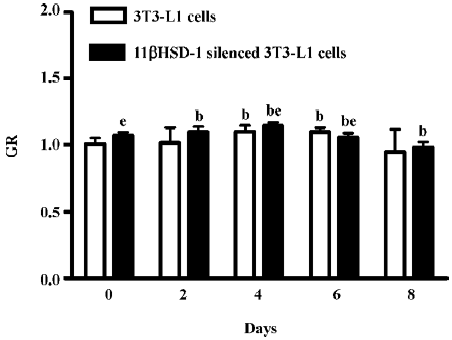
Expression of markers of 3T3-L1 differentiation The expression of several adipocyte differentiation markers, such as fatty acid synthetase and lipoprotein lipase, was also upregulated upon stimulation of differentiation in the non-transfected 3T3-L1 cells and the cells transfected with pGCsilencer H1/TetO1, but were downregulated in the cells transfected with 11beta-HSD1-siRNA (Figure 5). Similarly, the expression of preadipocyte factor-1, an inhibitor of adipocyte differentiation, was downregulated upon stimulation of differentiation and had no changes in the cells transfected with 11beta-HSD1 siRNA (Figure 5).
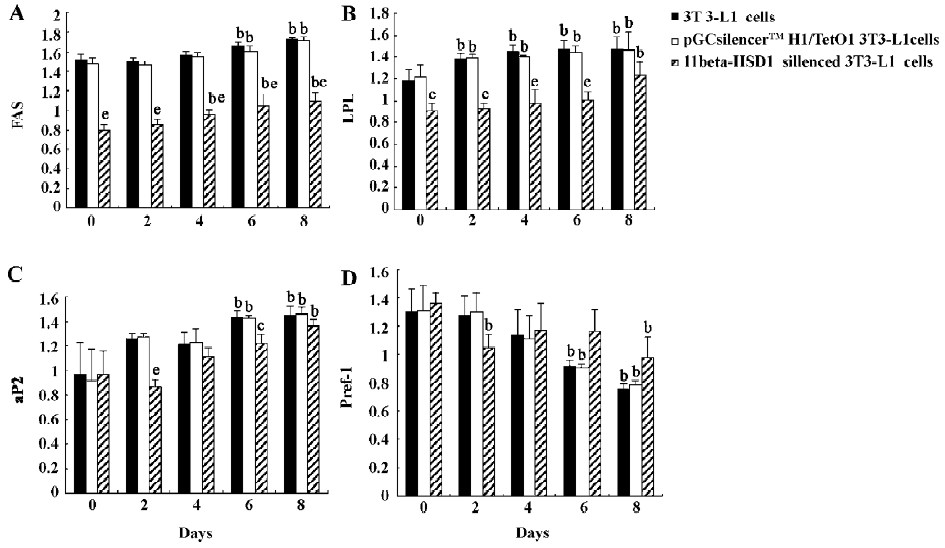
Discussion
Just as transgenic and knockout models allow researchers to study gene function in vivo, overexpression and knocking down with RNA interference are powerful technologies for exploring gene function in mammalian cultured cells.
11beta-HSD1 is a microsome enzyme located in various tissues. It interconverts inactive cortisone and active cortisol, regulating the level of cortisol. Glucocorticoid as a prereceptor regulator promotes adipocyte differentiation.
Recent studies in mouse models have suggested that 11beta-HSD1 may represent the long-sought link between obesity and insulin resistance[1,4,11]. 11beta-HSD1 catalyzes the conversion of glucocorticoid molecules from an inactive to an active form. There is accumulating evidence suggesting that glucocorticoids may play a role in adipocyte maturation, at least in part, via the inhibition of cell proliferation and the induction of differentiation[8]. To verify this hypothesis, we used in vitro RNAi experiments to explore the effect of 11beta-HSD1 on preadipocyte differentiation. We synthesized 2 pairs of mouse 11beta-HSD1 mRNA-specific interfere plasmids: pGCsilencer H1/TetO1–11beta-HSD1–1 and pGCsilencer H1/TetO1–11beta-HSD1–2. The pGCsilencer H1/TetO1–11beta-HSD1–2 showed significant silencing effects on the expression of 11beta-HSD1 at both RNA and protein levels (Figure 1). It is not clear why pGCsilencer H1/TetO1–11beta-HSD1–1 did not show significant silencing effects.
Compared with non-transfected 3T3-L1 cells, we found that pGCsilencer H1/TetO1–11beta-HSD1–2-transfected cells exhibited much less adipoconversion and triglyceride (Figure 2A,B). Small lipid droplets could be observed in transfected cells as late as d 8. This notable decrease in adipoconversion was consistent throughout the whole process of differentia-tion.
The mechanisms by which 11beta-HSD1 regulates adipocyte differentiation are still unclear. Glucocorticoids inhibit cellular proliferation by inducing cell cycle arrest at the G1 phase; the prereceptor modulation of cortisol metabolism has a dramatic effect on the cell proliferation rate[7,8]. Numerous studies have focused on examining the effects of various steroid moieties on 11beta-HSD1 activity since factors that inhibit metabolism of the 11beta-hydroxyl group increase glucocorticoid potency. Glucocorticoids, the C/EBP-α (CAATT enhancer binding proteins alpha), PPAR-gamma (peroxisome proliferator activiated receptor) agonists, and some pro-inflammatory cytokines (TNF-alpha, interleukin 1-beta) can increase 11beta-HSD1 expression, while GH (growth hormone), acting via IGF-1 (insulin-like growth factor-1) and LXR (liver X receptor) agonists can inhibit its expression[12–14]. A key question remains concerning the extent to which the upregulation of adipose 11beta-HSD1 in obesity influences intra-adipose cortisol levels and the metabolic consequences of obesity.
In the present study, the level of 11beta-HSD1 increased (Figure 3) in the differentiation of 3T3-L1, but GR was increased shortly after induced differentiation in the 3T3-L1 cells, even following 11beta-HSD1 knock-down (Figure 4). In light of the known effect of glucocorticoids on adipose tissue function and distribution, it has been postulated that the enhanced conversion of E to F by 11beta-HSD1 within omental adipose tissue may play an important role in the pathogenesis of central obesity. Cortisol is essential for adipocyte differentiation[15], while 11beta-HSD1 promotes differentiation and may be related to the development of obesity and insulin-resistance.
Recently, it has been shown that the inhibition of GR using a 10-fold molar excess of the GR-antagonist RU38486 (the name of the drug), could block the cortisol-mediated upregulation of 11beta-HSD1 expression and 11-oxoreductase activity. These results suggest that this effect is mediated exclusively by GR. However, 11beta-HSD1 is thought to modulate glucocorticoid hormone action by regulating ligand supply to GR[16], and our results suggest that it may be an autoprotected mechanism for the effect of glucocorticoids.
This study provides a useful new model for determining the relative impact of the divergent GR-mediated pathways on cell growth. The data presented here emphasize the pivotal role of 11beta-HSD1 as a prereceptor determinant of GR-mediated signal transduction. It has recently been shown that 11beta-HSD1 activity regulates adipocyte differentiation in an autocrine manner[17] by supplying adequate amounts of cortisol to support cell differentiation and preventing excessive and detrimental amounts of cortisol in mature cells. This study demonstrates the importance of this autocrine 11beta-HSD1 action for proper cell differentiation and function. This may explain why the timing of 11beta-HSD1 expression is correlated with that of GR when stimulated by cortisol[18]. Cortisol induces adipogenesis and 11beta-HSD1 activity in preadipocytes. When adipocyte differentiation is initiated, 11beta-HSD1 dehydrogenase activity is switched to reductase activity which generates cortisol and thereby promotes adipocyte differentiation[7,19,20].
In summary, our data support the hypothesis that 11beta-HSD1 acts as a prereceptor regulator to stimulate preadi-pocyte differentiation in an autocrine manner. The level of triglyceride decreased and the transcription of some marker genes were downregulated in 11beta-HSD1 siRNA cells (Figures 2B, 5). Thus, this enzyme may serve as a critical link between obesity and the development of insulin resistance. Further studies will be needed to explore the regulation and function of 11beta-HSD1 at the transcription and expression levels. A better understanding of the biological pathways mediated by 11beta-HSD1 may lead to new therapies for obesity and diabetes mellitus.
References
- Wake DJ, Walker BR. 11 beta-Hydroxysteroid dehydrogenase type 1 in obesity and the metabolic syndrome. Mol Cell Endocrinol 2004;215:45-54.
- Kotelevtsev YV, Holmes MC, Burchell A. 11 beta-Hydroxysteroid dehydrogenase type 1 knockout mice show attenuated glucocorticoid inducible responses and resist hyperglycaemia on obesity or stress. Proc Natl Acad Sci USA 1997;94:14924-9.
- Morton NM, Holmes MC, Fievet C. Improved lipid, lipoprotein profile, hepatic insulin sensitivity, and glucose tolerance in 11 beta-hydroxysteroid dehydrogenase type 1 null mice. J Biol Chem 2001;276:41293-300.
- Walker BR. 11beta-Hydroxysteroid dehydrogenase type 1 in obesity. Obes Res 2004;12:1-3.
- Masuzaki H, Paterson J, Shinyama H, Morton NM, Mullins JJ, Seckl JR, et al. A transgenic model of visceral obesity and the metabolic syndrome. Science 2001;294:2166-70.
- Masuzaki H, Yamamoto H, Kenyon CJ, Elmquist JK, Morton NM, Paterson JM, et al. Transgenic amplification of glucocorticoid action in adipose tissue causes high blood pressure in mice. J Clin Invest 2003;112:83-90.
- Tomlinson JW, Walker EA, Bujalska IJ, Draper N, Lavery GG, Cooper MS, et al. 11β-hydroxysteroid dehydrogenase type 1: A tissue-specific regulator of glucocorticoid response. Endocr Rev 2004;25:831-66.
- Rabbitt EH, Lavery GG, Walker EA, Cooper MS, Stewart PM, Hewison M. Prereceptor regulation of glucocorticoid action by 11beta-hydroxysteroid dehydrogenase: A novel determinant of cell proliferation. FASEB J 2002;16:36-44.
- Felmer RN, Clark JA. The gene suicide system ntr/cb1954 causes ablation of differentiated 3t3l1 adipocytes by apoptosis. Biol Res 2004;37:449-60.
- Blagoev B, Kmousechmarova I, Nielsen MM, Fernandez MM, Voldby J, Andersen JS, et al. Inhibition of adipocyte differentiation by resistin -like molecule alpha. Biochemical characterization of its oligomeric nature. J Biol Chem 2002;277:42011-6.
- Nair S, Lee YH, Lindsay RS, Walker BR, Tataranni PA, Bogardus C, et al. 11 beta-Hydroxysteroid dehydrogenase type 1: genetic polymorphisms are associated with Type 2 diabetes in Pima Indians independently of obesity and expression in adipocyte and muscle. Diabetologia 2004;47:1088-95.
- Moisan MP, Edwards CR, Seckl JR. Differential promoter usage by the rat 11 beta-hydroxysteroid dehydrogenase gene. Mol Endocrinol 1992;6:1082-7.
- Williams LJ, Lyons V, MacLeod I, Rajan V, Darlington GJ, Poli V, et al. C/ebp regulates hepatic transcription of 11beta-hydroxysteroid dehydrogenase type 1. A novel mechanism for cross-talk between the c/ebp and glucocorticoid signaling pathways. J Biol Chem 2000;275:30232-9.
- Berger J, Tanen M, Elbrecht A, Hermanowski-Vosatka A, Moller DE, Wright SD, et al. Peroxisome proliferator-activated receptor-gamma ligands inhibit adipocyte 11beta-hydroxysteroid dehydrogenase type 1 expression and activity. J Biol Chem 2001;276:12629-35.
- Yang K, Khalil MW, Strutt BJ, Killinger DW. 11beta-Hydroxy-steroid dehydrogenase 1 activity and gene expression in human adipose stromal cells: effect on aromatase activity. J Steroid Biochem Mol Biol 1997;60:247-53.
- Lindsay R, Deborah J. Subcutaneous adipose 11beta-hydroxy-steroid dehydrogenase type 1 activity and messenger ribonucleic acid levels are associated with adiposity and insulinemia in Pima Indians and Caucasians. J Clin Endocrinol Metab 2003;88:2738-44.
- Eijken M, Hewison M, Cooper MS, de Jong FH, Chiba H, Stewart PM, et al. 11β-hydroxysteroid dehydrogenase expression and glucocorticoid synthesis are directed by a molecular switch during osteoblast differentiation. Mol Endocrinol 2005;19:621-31.
- Engeli S, Bohnke J, Feldpausch M, Gorzelniak K, Heintze U, Janke J. et al. Regulation of 11β-HSD genes in human adipose tissue: influence of central obesity and weight loss. Obes Res 2004;12:9-17.
- Bujalska IJ, Walker EA, Hewison M, Stewart PM. A switch in dehydrogenase to reductase activity of 11β-hydroxysteroid dehydrogenase type 1 upon differentiation of human omental adipose stromal cells. J. Clin Endocrinol Metab 2002;87:1205-10.
- Bujalska IJ, Kumar S, Hewison M, Stewart PM. Differentiation of adipose stromal cells: The roles of glucocorticoids and 11β-hydroxysteroid dehydrogenase. Endocrinology 1999;140:3188-96.