Propofol attenuation of renal ischemia/reperfusion injury involves heme oxygenase-1
Introduction
Renal ischemia/reperfusion (I/R)-induced tubular epithelial cell injury[1], called ischemic acute renal failure, is associated with high mortality in humans[2]. Protecting the kidney against I/R injury is very important during complicated renal operations and anesthesia.
Propofol, a highly lipid-soluble anesthetic, is widely used for the induction and maintenance of general anesthesia, as well as for intubated post-operation sedation for mechanically-ventilated adults in the intensive care unit. Propofol has been proven to ameliorate I/R injury in several organs, including the heart[3], lungs[4], brain[5], liver[6], and testicles[7]. Propofol can also limit oxidative injury in various tissues, including the kidneys[8]. However, the effects of propofol on renal I/R injury have been rarely reported.
Heme oxygenase (HO) catalyzes the conversion of heme to biliverdin, carbon monoxide, and free ferrous iron; the latter is rapidly converted to bilirubin by biliverdin reductase[9]. Among the 3 isoforms HO-1 is a ubiquitous and redox-sensitive inducible stress protein that is strongly induced by various stimuli, including heme, heavy metal, cytokines, hormones, endotoxins, heat shock, and I/R injury, while HO-2 is the constitutive form[10]. HO-3 has been identified, but its function is still unknown[11]. Furthermore, HO-1 has been shown to have protective effects against I/R injury. HO-1 induction in donor organs of rats has been shown to ease I/R injury, prolong graft survival, and improve the long-term function of the grafted kidney[12]. It has also been reported that the clipping of the renal artery in HO-1-deficient mice leads to the exacerbation of renal damage and death[13]. Recently, Acquaviva et al indicated that propofol attenuated peroxynitrite-mediated DNA damage and apoptosis by the upregulation of HO-1 expression in cultured astrocytes[14].
Based on these data, it is reasonable for us to hypothesize that propofol can inhibit renal I/R injury, and this effect is partly mediated by HO-1.
Materials and methods
Materials Propofol was obtained from Astrazeneca Pharmaceuticals (Milan, Italy). Polyclonal rabbit anti-rat HO-1 antibody and polyclonal rabbit anti-rat actin antibody were purchased from Santa Cruz Biotechnology (Santa Cruz, CA, USA). Secondary horseradish peroxidase-conjugated goat anti-rabbit IgG was purchased from Shanghai Sangon Biotechnology (Shanghai, China). Peroxidase-conjugated goat anti-rabbit secondary antibody was from Dako (Carpinteria, CA, USA). The One-step RNA PCR kit (Avian Myeloblastosis Virus, AMV) was obtained from TaKaRa Biotechnology (Otsu, Japan). Male Sprague–Dawley rats (200–250 g, Grade II) were from Zhejiang Academy of Medical Sciences (Hangzhou, China).
Experimental grouping This study was approved by our institutional animal research committee and conformed to the Guide for the Care and Use of Laboratory Animals published by the United States National Institutes of Health (NIH publication N
Induction of renal I/R and tissue preparation The rats were anesthetized with ketamine (50 mg/kg, ip). Body temperature was maintained at 37 °C by a heating lamp until the rats recovered from the anesthesia. The tail vein was cannulated for fluid or drug administration. Bilateral renal ischemia was induced by occlusion of both renal pedicles using non-traumatic microvascular clips. After 45 min ischemia, the clips were removed allowing the kidneys to reperfuse. Occlusion was verified visually by a change in the color of the kidneys to a paler shade, and reperfusion by a blush[17]. After 2, 6, and 24 h reperfusion, the rats of the I/R group and the propofol group were anesthetized with ketamine (50 mg/kg, ip). The chest and peritoneal cavities were opened carefully and blood was drawn from the heart for the measurement of blood urea nitrogen (BUN) and serum creatinine (SCr) levels. The kidneys were removed and cut into 3 parts; 1 part was fixed with 12% formaldehyde for histological and immunohistochemical analysis and the others were frozen at -80 °C for RNA and protein isolation. The samples of the sham-operated rats were collected only 2 h after the sham operation.
Histology The kidneys were embedded in paraffin, serial sectioned (3–4 µm thick) and stained with HE[18]. The slides were reviewed blindly and scored with a semiquantitative scale evaluating morphological characteristics of the tubules by Paller’s standard. Specifically, for each kidney, 100 cortical tubules from at least 10 different areas were scored. Care was taken to avoid repeated scoring of different convolutions of the same tubule. Higher scores represented more severe damage (maximum score per tubule was 10), with points given for the presence and extent of tubular epithelial cell flattening (1 point), brush border loss (1 point), cell membrane bleb formation (1 or 2 points), interstitial edema (1 point), cytoplasmic vacuolization (1 point), cell necrosis (1 or 2 points), and tubular lumen obstruction (1 or 2 points)[19,20].
Immunohistochemistry The kidney tissue sections were hybridized with rabbit anti-rat HO-1 antibody (dilution 1:60) at 4 °C overnight. After washing, the sections were overlaid with peroxidase-conjugated goat anti-rabbit secondary antibody at 37 °C for 30 min. The negative control was prepared by PBS of the primary antibody. By counting the number of positive-stained kidney cells in 5 high-power (×400) fields per case, we determined the positive rate of HO-1. The standards for the HO-1 quantification was as follows: 0%, 0 point; <25%, 1 point; 25%–50%, 2 points; 50%–75%, 3 points; and >75%, 4 points.
RT-PCR The total RNA was extracted by the TRIzol reagent (Invitrogen, Carlsbad, CA, USA). Briefly, cDNA was prepared from 1 µg total RNA using the One-step RNA PCR kit (AMV). The primer sequences were as follows: HO-1 sense, 5'-ACT GCT GAC AGA GGA ACA CAA A-3'; HO-1 antisense, 5'-CAA CAG GAA ACT GAG TGT GAG G-3'; GAPDH sense, 5'-AAG GTC GGA GTC AAC GGA TTT-3'; and GAPDH antisense, 5'-AGA TGA TGA CCC TTT TGG CTC-3'.
GAPDH was used as a loading control. The amplification cycle was 95 °C for 1 min, 55 °C for 1 min, and 72 °C for 1 min, repeated for 30 cycles. RT-PCR products 8 μL were separated by electrophoresis on 1.8% agarose gel containing ethidium bromide 0.5 μg/mL (181 bp for HO-1 and 352 bp for GAPDH).
Western blot analysis The kidney tissues were homogenized in 10 mL homogenization buffer (20 mmol/L Tris, 5 mmol/L EDTA, 150 mmol/L NaCl, 1 mmol/L phenyl-methanesulfonyl fluoride) and centrifuged at 12 000×g at 4 °C for 10 min. The resulting supernatant was mixed with loading buffer and boiled for 3 min. The samples containing 50 µg protein were separated by 12% SDS-PAGE and transferred to nitrocellulose membranes. The membranes were blocked with 5% non-fat dry milk in TBST solution at room temperature for 2 h and incubated with the specific primary antibody against HO-1 at a dilution of 1: 200 at 4 °C overnight. After washing with TBST, the membranes were incubated with horseradish peroxidase-conjugated goat anti-rabbit IgG at a dilution of 1:2000 at room temperature for 1 h. The membranes were washed 3 times with TBST solution for 45 min each. Immunoreactive bands were visualized and quantified with the Quantity One Image software (Bio-Rad, CA, USA).
Statistical analysis All data were expressed as mean± SD. SPSS 10.0 software (Chicago, USA) was used for the data analysis. Difference was analyzed by one-way ANOVA and H-test. P< 0.05 was considered statistically significant.
Results
Effect of propofol on serum BUN and SCr levels The level of BUN in the I/R group (38.12±3.57 mg/dL, 43.68±9.99 mg/dL, and 99.84±8.63 mg/dL at 2, 6, and 24 h after reperfusion, respectively) was significantly increased compared with the sham-operated group (17.46±3.8 mg/dL, P<0.05 at 6 h and P<0.01 at 2 and 24 h). The level of SCr in the I/R group (1.51±0.22 and 2.1±0.27 mg/dL at 6 and 24 h after reperfusion) was higher than that in the sham-operated group (0.94±0.05 mg/dL, P<0.05 at 6 h and P<0.01 at 24 h). Treatment with propofol significantly improved the renal injury induced by I/R at 24 h (BUN, 67.48±8.28 vs 99.84±8.63 mg/dL, P<0.01; SCr, 1.39±0.15 vs 2.1±0.27 mg/dL, P<0.01).
Histopathological analysis In the I/R group, the histopathological sections showed an obvious loss of brush border, bleb formation, cytoplasmic vacuolization, cell necrosis, and dilation of the renal tubules with proteinaceous casts. The pathological changes were markedly improved with the treatment of propofol (Figure 1). The mean histological score by Paller’s standard was obviously increased in the I/R group (94.0±14.7, 153.0±29.7, and 251.4±33.8 at 2, 6, and 24 h after reperfusion, respectively) compared with the sham-operated group (20.4±4.6, n=5, P<0.01). When propofol was added, the increase of the mean histological score caused by I/R was significantly suppressed at 6 (100.6±15.8 vs 153.0±29.7, n=5, P<0.01) and 24 h after reper-fusion (173.0±21.8 vs 251.4±33.8, P<0.01) (Figure 2).
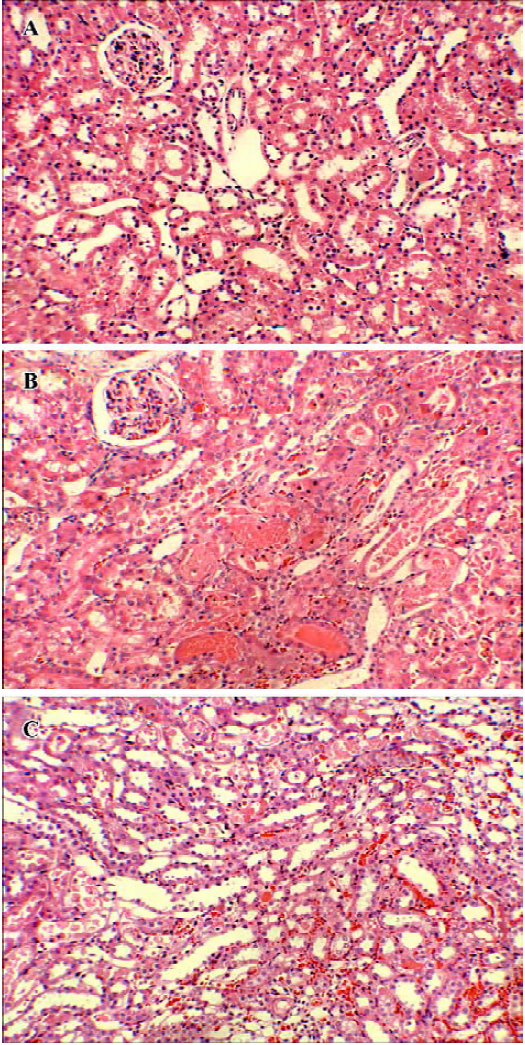
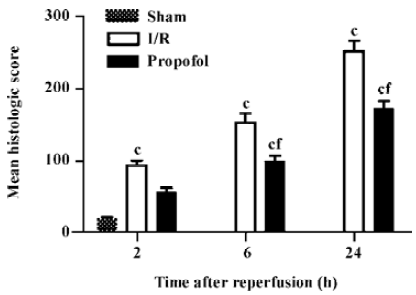
Immunohistochemical expression of HO-1 in the kidney tissues In the propofol-treated rat kidney tissues, a pronounced increase in intensity of HO-1 immunostaining was observed in the proximal and distal tubuli in the cortex and prominently in the outer strip region of the outer medulla when compared with the I/R group (0.36±0.17 vs 0.04±0.09, 1.48±0.23 vs 0.76±0.26, and 1.36±0.26 vs 0.64±0.17, n=5, P<0.05 at 2, 6, and 24 h after reperfusion, respectively), while it was not detected in the tissues from the sham-operated group (Figures 3, 4).
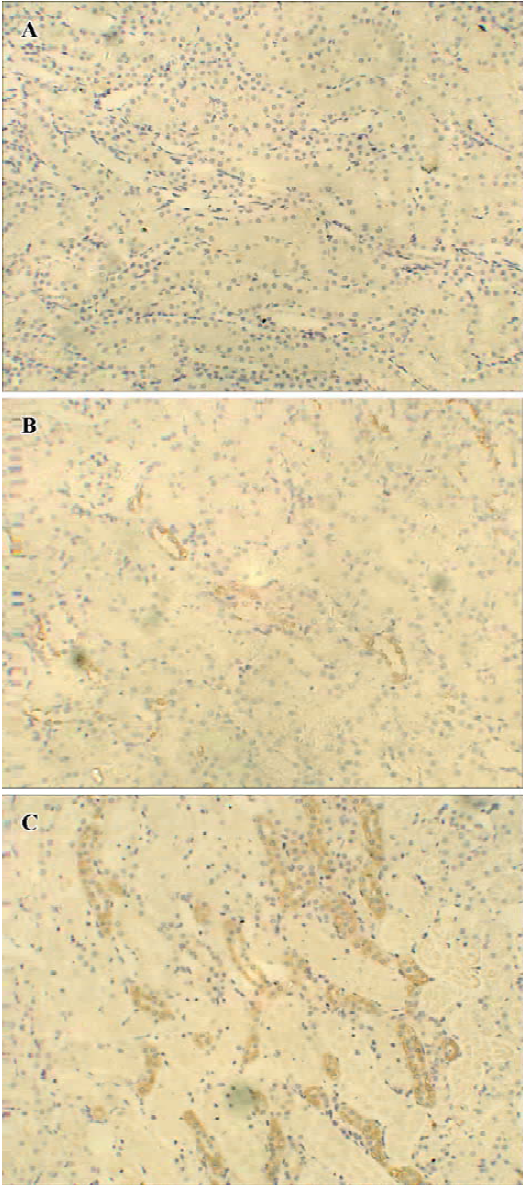
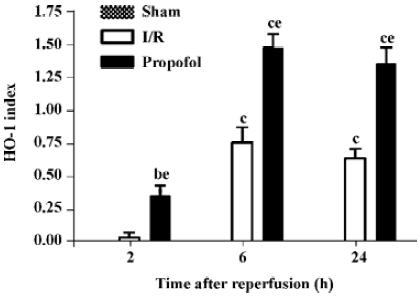
mRNA expression of HO-1 in the kidney tissues At 6 and 24 h after reperfusion, the transcription level of HO-1 increased in the I/R group compared with the sham-operated group (P<0.01, n=4). However, the transcription level of HO-1 was comparable with or without I/R treatment at 2 h. Interestingly, propofol dramatically elevated the HO-1 transcription level 2 h after reperfusion (P<0.01, n=4), and also increased the HO-1 transcription level 6 and 24 h after reperfusion (P<0.05, n=4) (Figure 5).
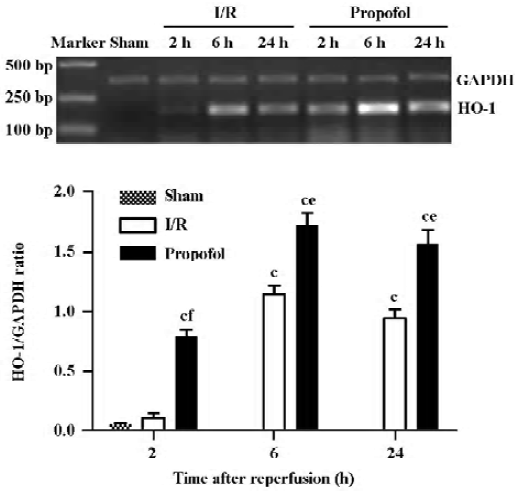
Quantitation of the HO-1 protein expression in the kidney tissues using Western blot analysis The induction of HO-1 was not only at the transcription level, but also occurred at the expression level. The expression of HO-1 markedly increased in the I/R-treated kidneys 6–24 h after reperfusion (P<0.01, n=4), whereas the HO-1 protein was expressed at an exceedingly low level in the I/R and sham-operated group 2 h after reperfusion. In contrast, the expression of the HO-1 protein was observed 2 h after reperfusion, and increased 6 and 24 h after reperfusion in the propofol-treated kidneys than that in I/R-treated kidneys (P<0.05, n=4) (Figure 6).
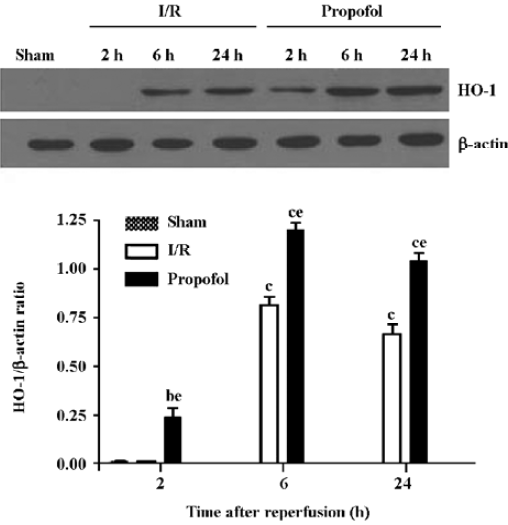
Discussion
In the current study, we demonstrated that propofol treatment significantly reduced renal dysfunction and injury in renal I/R rats. Furthermore, these protective effects are in part mediated by the induction of HO-1.
Propofol is known to exert protective effects against I/R injury on various organs[3–7,21]. Our data also demonstrated that propofol effectively reduced the increase of BUN and SCr levels, and the mean histological score induced by I/R. The morphological abnormality of kidney tissues was eased by treatment with propofol, which suggests that propofol has protective effects against renal I/R injury. The production of free radicals and subsequent lipid peroxidation plays a key role in I/R injury. Propofol has an antioxidant property, and the majority of studies attributed this capacity to the phenolic structure of propofol. Propofol appears to inhibit lipid peroxidation either by reacting with lipid peroxyl radicals to form the relatively stable propofol phenoxyl radical[22], or by scavenging peroxynitrite, which is an important molecule in the cellular toxicity of I/R[23], or both. The other important factors involved in the development of I/R injury are the activation of neutrophils overloaded with cellular calcium and the opening of the mitochondrial permeability transition pore (MPTP). Propofol can inhibit the activity of neutrophils[24] and calcium influx across plasma membranes[25]. It has also reported that the protective effect of propofol against I/R injury was accompanied by less MPTP opening in isolated hearts[26]. These mechanisms may partially explain our results. Moreover, we found that propofol ameliorated renal I/R injury accompanied with an up-regulation of HO-1 expres-sion.
Scapagnini et al suggested that the ultimate stimulation of the HO-1 pathway was likely to account for the antioxidant/anti-inflammatory properties of bioactive polyphenols[27]. The induction of HO-1 has been shown to play an impor-tant role in the adaptive protection of tissues against I/R injury[12,13,28]. The end products of heme degradation such as biliverdin, bilirubin, and CO provide important physiological roles. Both biliverdin and bilirubin could remove reactive oxygen species generated by I/R and are thereby regarded as potent endogenous antioxidants[29]. CO relaxes blood vessels[30] and inhibits the proliferation of vascular smooth muscle cells[31]. In addition, CO is capable of suppressing platelet aggregation[32]. These features suggest that HO-1 plays a critical role in microcirculation. Our data showed that when induced 6 h after reperfusion, propofol effectively potentiated the transcription and expression levels of HO-1. In the propofol-treated group, both the transcription and expression of HO-1 could be detected, even 2 h after reperfusion. Moreover, propofol treatment significantly reduced the renal dysfunction and histological damage caused by I/R. These data, together with previously published reports[14,27], suggest that the induction of HO-1 might be an important mechanism for the protective effects of propofol against renal I/R injury.
In conclusion, propofol attenuates renal I/R injury in rats, which might provide an effective strategy for renal protection during anesthesia. Furthermore, HO-1 might be a new protective pathway of propofol against renal I/R injury.
References
- Bonventre JV. Mechanisms of ischemic acute renal failure. Kidney Int 1993;43:1160-78.
- Star RA. Treatment of acute renal failure. Kidney Int 1998;54:1817-31.
- Lim KH, Halestrap AP, Angelini GD, Suleiman MS. Propofol is cardioprotective in a clinically relevant model of normothermic blood cardioplegic arrest and cardiopulmonary bypass. Exp Biol Med 2005;230:413-20.
- Balyasnikova IV, Visintine DJ, Gunnerson HB, Paisansathan C, Baugluman VL, Minshall RD, et al. Propofol attenuates lung endothelial injury induced by ischemia-reperfusion and oxidative stress. Anesth Analg 2005;100:929-36.
- Ergun R, Akdemir G, Sen S, Tasci A, Ergungor F. Neuroprotective effects of propofol following global cerebral ischemia in rats. Neurosurg Rev 2002;25:95-8.
- Lin LN, Wang WT, Wu JZ, Hu ZY, Xie KJ. Protective effect of propofol on liver during ischemia-reperfusion injury in patients undergoing liver surgery. Zhongguo Wei Zhong Bing Ji Jiu Yi Xue 2004;16:42-4. Chinese..
- Unsal A, Devrim E, Guven C, Eroglu M, Durak I, Bozoklu A, et al. Propofol attenuates reperfusion injury after testicular torsion and detorsion. World J Urol 2004;22:461-5.
- Runzer TD, Ansely DM, Godin DV, Chambers GK. Tissue antioxidant capacity during anesthesia: propofol enhances in vivo red cell and tissue antioxidant capacity in a rat model. Anesth Analg 2002;94:89-93.
- Foresti R, Motterlini R. The heme oxygenase pathway and its interaction with nitric oxide in the control of cellular homeo-stasis. Free Radic Res 1999;31:459-75.
- Ohta K, Yachie A, Fujimoto K, Kaneda H, Wada T, Toma T, et al. Tubular injury as a cardinal pathologic feature in human heme oxygenase-1 deficiency. Am J Kidney Dis 2000;35:863-70.
- McCoubrey WK Jr, Huang TJ, Maines MD. Isolation and characterization of a cDNA from the rat brain that encodes hemoprotein heme oxygenase-3. Eur J Biochem 1997;247:725-32.
- Tullius SG, Nieminen-Kelha M, Buelow R, Reutzel-Selke A, Martins PN, Pratschke J, et al. Inhibition of ischemia/reperfusion injury and chronic graft deterioration by a single-donor treatment with cobalt-protoporphyrin for the induction of heme oxygenase-1. Transplantation 2002;74:591-8.
- Wiesel P, Patel AP, Carvajal IM, Wang ZY, Pellacani A, Maemura K, et al. Exacerbation of chronic renovascular hypertension and acute renal failure in heme oxygenase-1-deficient mice. Circ Res 2001;88:1088-94.
- Acquaviva R, Campisi A, Murabito P, Raciti G, Avola R, Mangia-meli S, et al. Propofol attenuates peroxynitrite-mediated DNA damage and apoptosis in cultured astrocytes. Anesthesiology 2004;101:1363-71.
- Yagmurder H, Akca G, Aksoy M, Baltaci B, Dikmen B. The effects of ketamine and propofol on bacterial translocation in rats after burn injury. Acta Anasethesiol Scand 2005;49:177-82.
- Gelb AW, Bayona NA, Wilson JX, Cechetto DF. Propofol anesthesia compared to awake reduces infarct size in rats. Anesthesiology 2002;96:1183-90.
- Chen CC, Liu ZM, Wang HH, He W, Wang Y, Wu WD. Effects of ulinastatin on renal ischemia-reperfusion injury in rats. Acta Pharmacol Sin 2004;25:1334-40.
- Pedraza-Chaverri J, Maldonado PD, Medina-Campos ON, Olivares-Corichi IM, Granados-Silvestre MA, Hernandez-Pando R, et al. Garlic ameliorates gentamicin nephrotoxicity: relation to antioxidant enzymes. Free Radic Biol Med 2000;29:602-11.
- Paller MS, Hoidal JR, Ferris TF. Oxygen free radicals in ischemic acute renal failure in the rat. J Clin Invest 1984;74:1156-64.
- Wetzels JF, Yu L, Shanley PF, Burke TJ, Schrier RW. Infusion of glycine does not attenuate in vivo ischemia acute renal failure in the rat. J Lab Clin Med 1993;121:263-7.
- Cheng YJ, Wang YP, Chien CT, Chen CF. Small-dose propofol sedation attenuates the formation of reactive oxygen species in tourniquet-induced ischemia-reperfusion injury under spinal anesthesia. Anesth Analg 2002;94:1617-20.
- Murphy PG, Bennett JR, Myers DS, Davies MJ, Jones JG. The effect of propofol anaesthesia on free radical-induced lipid peroxidation in rat liver microsomes. Eur J Anaesth 1993;10:261-6.
- Mathy-Hartert M, Mouithys-Mickalad A, Kohnen S, Deby-Dupont G, Lamy M, Hans P. Effects of propofol on endothelial cells subjected to a peroxynitrite donor (SIN-1). Anaesthesia 2000;55:1066-71.
- Nagata T, Kansha M, Irita K, Takahashi S. Propofol inhibits FMLP-stimulated phosphorylation of p42 mitogen-activated protein kinase and chemotaxis in human neutrophils. Eur J Anaesth 2001;86:853-8.
- Puttick RM, Terrar DA. Differential effects of propofol and enflurane on contractions dependent on calcium derived from the sarcoplasmic reticulum of guinea pig isolated papillary muscles. Anesth Analg 1993;77:55-60.
- Javadov SA, Lim KHH, Kerr PM, Suleiman MS, Angelini GD, Halestrap AP. Protection of hearts from reperfusion injury by propofol is associated with inhibition of the mitochondrial permeability transition. Cardiovasc Res 2000;45:360-9.
- Scapagnini G, Foresti R, Calabrese V, Giuffrida Stella AM, Green CJ, et al. Caffeic acid phenethyl ester and curcumin: a novel class of heme oxygenase-1 inducers. Mol Pharmacol 2002;61:554-61.
- Maines MD, Raju VS, Panahian N. Spin trap (N-t-butyl-alpha-phenylnitrone)-mediated suprainduction of heme oxygenase-1 in kidney ischemia/reperfusion model: role of the oxygenase in protection against oxidative injury. J Pharmacol Exp Ther 1999;291:911-9.
- Stocker R, Yamamoto M, McDonagh AF, Glazer AN, Ames BN. Bilirubin is an antioxidant of possible physiological importance. Science 1987;235:1043-6.
- Ndisang JF, Tabien HE, Wang R. Carbon monoxide and hypertension. J Hypertens 2004;22:1057-74.
- Stanford SJ, Walters MJ, Hislop AA, Haworth SG, Evans TW, Mann BE, et al. Heme oxygenase is expressed in human pulmonary artery smooth muscle where carbon monoxide has an anti-proliferative role. Eur J Pharmacol 2003;473:135-41.
- Beltowski J, Jamroz A, Borkowska E. Heme oxygenase and carbon monoxide in the physiology and pathology of the cardiovascular system. Postepy Hig Med Dosw 2004;58:83-99. (online).