Decreased expression of human aquaporin-5 correlated with mucus overproduction in airways of chronic obstructive pulmonary disease1
Introduction
Water transport across epithelial and endothelial barriers in bronchopulmonary tissues occurs during airway hydration, alveolar fluid transport, and submucosal gland secretion. Many of the tissues involved in these processes are highly water permeable and express aquaporin (AQP) water channels[1]. AQP1 is expressed in microvascular endothelia throughout the lung and airways, AQP3 in basal epithelial cells in large airways and throughout the nasopharynx, AQP4 at the basolateral plasma membrane surface in epithelia throughout the trachea and the small and large airways, and AQP5 in type I alveolar epithelial cells and submucosal gland acinar cells[2]. In the lungs, AQP1 and AQP5 provide the principal route for osmotically-driven water transport[3]. AQP5 deletion in submucosal glands in the upper airways reduced fluid secretion and increased protein content[4].
Chronic obstructive pulmonary disease (COPD) is a major cause of chronic morbidity and mortality throughout the world, and is usually complicated by mucus hypersecretion in the airways leading to repeated infection[5] and irrever-sible airflow limitation[6]. Mucus consists mainly of water (95%) combined with salts, lipids, proteins, and glycoprotein. Abnormal mucus secretions have been proposed to promote bacterial adhesion and inhibit bacterial clearance by impeding cilia function[7], which may lead to exacerbations in COPD patients. So far, studies about mucus are focused on the regulation of mucin synthesis. However, the secretion of mucin is intimately related to the secretion of water and ions as well, for which little study has been done.
Thirteen mucin genes have been discovered[8]. Mucin 5AC (MUC5AC), a secreted mucin, colocalizes with glycoprotein-positive goblet cells and submucosal gland mucus cells. Thus, MUC5AC is the marker of mucus production in the epithelium airways[9]. In this study, we hypothesized that an abnormal gene expression of AQP may exist and become complicated by mucus hypersecretion in the airways of COPD patients. To this end, we examined the expression levels of AQP5, MUC5AC, and mucin in bronchial tissues of Chinese COPD patients to investigate the relationship of AQP5 and mucus overproduction.
Materials and methods
Bronchial tissues Bronchial tissues obtained from fiberoptic bronchoscopy and bronchial biopsy in West China Hospital (Sichuan, China) between April and July 2004 were prepared as described[10]. Twenty-five patients were diagnosed as COPD patients according to the Global Initiative for Chronic Obstructive Lung Disease (GOLD) 2004 (http://www.goldcopd.com), and another 20 were the control patients without COPD. Patients with asthma, cystic fibrosis, bronchiectasis, and diffuse panbronchiolitis were excluded. The COPD patients had history of productive coughing for 3 consecutive months each year for the past 2 years, with a forced expiratory volume in 1 s (FEV1) <80% of the predictive value, a FEV1/forced vital capacity (FVC) ratio <70%, and a reversibility in FEV1 <10% after inhalation of 400 µg salbutamol. According to FEV1% predicted value, there were 8 mild, 16 moderate, and 1 severe patient, respectively. The control patients had foreign body in the bronchus or benign bronchial stenosis and needed treatment with fiberoptic bronchoscopy. Eight healthy volunteers were also included in the control group (Table 1). All patients were ex-smokers or non-smokers, and the ex-smokers had given up smoking for more than 5 years. All patients had no respiratory tract infections within 2 weeks preceding the operation. The sites of biopsy were randomized to either the right or the left lower lobe, and 5 to 6 endobronchial biopsy specimens were taken from the tertiary carinae of the right or left lower lobes under direct visualization. The ethics committee of the West China Hospital, Sichuan University approved the study, and all patients gave written informed consent.
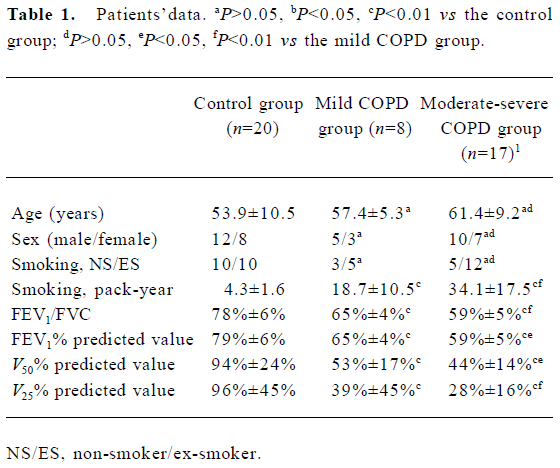
Full table
RT-PCR To examine the AQP5 mRNA levels in the human airways, semiquantitative RT-PCR was performed. Total RNA was isolated with Trizol reagent in accordance with the manufacturer’s protocol (Invitrogen, Carlsbad, CA, USA). The AQP5 primer sequences were as follows: upstream, 5'-AAG GCC GTG TTC GCA GAG TT-3' and downstream, 5'-TGG TCA GCT CCA TGG TCT TC-3'. The MUC5AC primer sequences were as follows[11]: upstream, 5'-TCC GGC CTC ATC TTC TCC-3' and downstream, 5'-ACT TGG GCA CTG GTG CTG-3'. The GADPH primer sequences were as follows: upstream, 5'-GAG CGA GAT CCC TCC AAA-3' and down-stream, 5'-ACT GTG GTC ATG AGT CCT TC-3'. The RT-PCR reagents were purchased from Promega Corporation (Madi-son, MI, USA). The reaction mixture contained 10 µL Avian Myeloblastosis Virus (AMV)/Thermus flacus (Tfl) 5×reaction buffer, 0.2 mmol/L deoxy-ribonucleoside triphosphate (dNTP) mixture, 1 µmol/L of each primer, 1 mmol/L MgSO4, 5U AMV reverse transcriptase, 5U Tfl DNA poly-merase, and 1 µg RNA sample in 50 µL volume. The first-strand cDNA was synthesized at 48 ℃ for 45 min, followed by denature of the template at 94 ℃ for 2 min. Amplication was achieved with 40 cycles of denaturing (94 ℃, 30 s), annealing (57 ℃, 1 min), and extension (68 ℃, 2 min). The final extension was performed at 68 ℃ for 7 min. The PCR products were analysed by 1.5% agarose gel electrophoresis, and the expressions were measured by densitometry using Molecular Analysis Software (BioRad, Hercules, CA, USA). Relative quantita-tions of gene expressions were normalized to GAPDH with each sample.
In situ hybridization The bronchial sections were fixed with 4% neutral buffered formaldehyde in 0.1% diethyl pyrocarbonate (DEPC). Six 4-µm sections were cut from each bronchial tissue block. A digoxigenin-labeled 24 bp RNA probe was designed by Shenergy Biocolor Company (Shanghai, China). The AQP5 primer sequence was 5'-AGA GCA GGT AGA AGT AAA GGA TGG CAG-3'. The probe was diluted in 50 pmol/µL. ISH reagents were purchased from Pan Path (Amsterdam, the Netherlands). Bound probes were detected with alkaline phosphatase-conjugated anti-digoxin (DIG) antibodies, and Nitroblue tetrazolium/5-bromo-4-chloro-3-indolyl phosphate was used as substrate. The sections were counterstained with methyl green. Hybridizations of several sections with AQP5 sense probes were used as negative controls.
The samples were dewaxed in xylene and dehydrated in a graded ethanol series prehybridization. Tissues were incubated for 15 min at 37 ℃ in 1 mg/mL proteinase K, and then blocked for 10 min in 0.1 mol/L triethanolamine and 0.25% acetic solution before equilibration in 2×standard saline citrate (SSC). The sections were prehybridized with 50% formamide in 2×SSC for 4 h at 50 ℃. Hybridization was carried out overnight at 42 ℃ and then the slides were washed for 15 min in 4×SSC at 37 ℃ and exposed for 30 min to 10 µg/mL RNase in 2×SSC at 37 ℃. After incubation for 30 min with normal goat blood serum, alkaline phosphatase-conjugated anti-DIG antibodies and Nitroblue tetrazolium/5-bromo-4-chloro-3-indolyl phosphate were added as substrates. The sections were counterstained with methyl green.
Histochemical analysis The bronchial sections were fixed with 4% neutral buffered formaldehyde. Six 4-µm sections were cut from each bronchial tissue block. Antihuman AQP5 polyclonal antibody and MUC5AC polyclonal antibody were purchased from Santa Cruz Biotechnology (Santa Cruz, CA, USA) and diluted at 1:100. The sections were stained with diaminobenzidine (DAB).
The samples were dewaxed in xylene and dehydrated in a graded ethanol series. Endogenous peroxidase activity was inhibited by incubating the slides with 3% H2O2 for 30 min, followed by washing in phosphate-buffered solution (PBS) and microwave heating (700 W) for 15 min in 10 mmol/L citric acid (pH 6.0). The sections were inactivated with normal goat serum for 20 min at 37 ℃. After repeated washing with PBS, the sections were incubated with the AQP5 and MUC5AC antibodies, placed in a humid chamber at 4 ℃ overnight, washed in PBS, and incubated at 37 ℃ for 30 min with biotinylated rabbit antigoat immunoglobulin, followed by repeated washing in PBS. The tissue was incubated with Streptavidin Peroxidase (SP) reagents for 30 min at 37 ℃ and then the sections were stained with freshly prepared DAB. After repeated washing with PBS and rinsing with tap water, the slides were counterstained with hematoxylin, and alcian blue-periodic acid-Schiff (AB-PAS) staining was performed as usual.
Image analysis All the images were generated by Spot Advanced Software (Diagnostic Instruments, MI, USA) and analyzed by IMAGE-Pro plus Version 4.5 (Media Cybernetics, HWY, USA). Two pathologists blinded to the patients’ clinical data reviewed all the images and made the analysis. High-power fields of bronchial tissues were randomly sampled and calculations were performed on 6 images per section examined at ×200 magnification. The mean of the 6 sections from each tissue was used for the statistical analysis. For the in situ hybridization and immunohistochemistry, the optical density (OD) of the positively-stained cells was normalized to the OD of the background in the same sections. For AB-PAS staining, the positively-stained areas in the submucosal glands were used to evaluate proliferations of the submucosal glands, normalized to areas of the bronchial tissues in the same fields of view.
Statistical analysis All data were expressed as the mean± SEM. Statistical analyses were performed using SPSS 12.0 software (SPSS Inc, IL, USA). Statistical significance was analyzed with one-way ANOVA, followed by Student-Newman-Keuls test to isolate significant difference. χ2-test and the Pearson correlation analysis were also used. A P-value less than 0.05 (2-tailed test) was considered statistically significant.
Results
AQP5 and MUC5AC mRNA expression in bronchial tissues To investigate the expression of AQP5 and MUC5AC mRNA, RT-PCR was performed. The signals of APQ5 and MUC5AC were detected in the bronchial tissues of all the patients. Bands of AQP5, MUC5AC, and GAPDH PCR products were located at 756, 682, and 298 bp, respectively (Figure 1). Compared with the control group, attenuated expressions of AQP5 were detected in the mild, moderate, and severe COPD groups (P<0.01, P<0.01). The mean OD of AQP5 were 0.68± 0.01 in the control group, 0.62±0.03 in the mild COPD group, and 0.56±0.04 in the moderate and severe COPD group (Figure 1A). The upregulated expressions of MUC5AC were also found in 2 COPD groups (P<0.01, P<0.01). The mean OD of MUC5AC were 0.43±0.03 in the control group, 0.48±0.05 in the mild COPD group, and 0.54±0.04 in the moderate and severe COPD group (Figure 1B). In the lung tissues, the differences in AQP5 in the groups were not noted and there was no MUC5AC expression in the lung tissues in all the groups (data not shown).
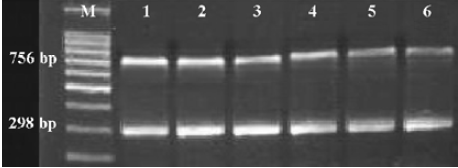
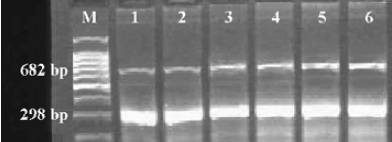
The influence of smoking on the expression of AQP5 and MUC5AC mRNA was also evaluated. There were 17 ex-smokers and 8 non-smokers in the COPD group, and 10 ex-smokers and 10 non-smokers in the control group. In the COPD group, the mean OD of AQP5 were 0.58±0.03 for the ex-smokers and 0.63±0.04 for the non-smokers, and the mean OD of MUC5AC were 0.53±0.04 for the ex-smokers and 0.49±0.03 for the non-smokers. Both differences were significant (P<0.05, P<0.05), but in the control group, the mean OD of AQP5 was 0.67±0.03 for the ex-smokers and 0.68±0.04 for the non-smokers, and the mean OD of MUC5AC was 0.45±0.04 for the ex-smokers and 0.43±0.03 for the non-smokers, without significant differences (P>0.05, P>0.05).
To identify the cell-specific expression of AQP5, in situ hybridization was performed. AQP5 expression was detected mainly in the submucosal gland cells, type I alveolar cells, and secondarily in the airway epithelial cells from all groups of patients. The positive signals (in purple) were expressed mainly in the nucleus and secondarily on the cell membrane. The COPD groups showed attenuated expressions and less positively-stained cells of submucosal glands and epithelial airway cells than the control group. The mean OD was 1.37±0.05 in the control group, 1.35±0.02 in the mild COPD patients, and 1.31±0.02 in the moderate and severe COPD patients; the differences were significant (P<0.01, P<0.01; (Figure 2A–2C). The differences of AQP5 expression in type I alveolar cells of all groups were not significant (P>0.05, P>0.05; data not shown). Hybridization with the sense probe revealed no staining, confirming the specificity of the assay (Figure 2D).
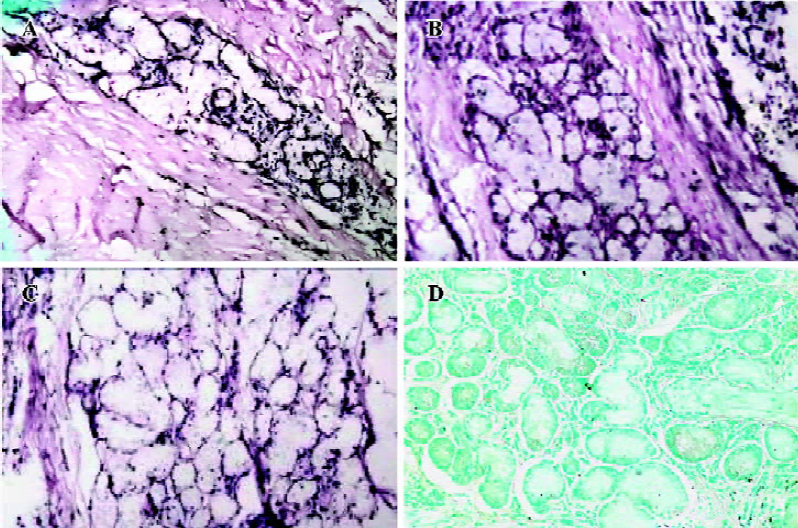
AQP5 and MUC5AC protein expression in bronchial tissues To examine the correlation between AQP5 expression and MUC5AC synthesis, immunohistochemical staining for AQP5 and MUC5AC were performed. AQP5 was expressed mainly in submucosal glands, type I alveolar cells, and secondarily at the apical membranes of cellula columno-epithelialis from patients in all the groups. The positive signals (in brown) were expressed mainly in the cytoplasm and secondarily on the cell membrane. The COPD groups showed attenuated expressions of AQP5 and less positively-stained cells of submucosal glands and epithelial airway cells than the control group. The mean OD was 1.36±0.02 in the control group, 1.34±0.01 in the mild COPD patients, and 1.30±0.02 in the moderate and severe COPD patients; the differences were significant (P<0.01, P<0.01; Figure 3A–3C). The differences of AQP5 expression in type I alveolar cells of all groups were not significant (P>0.05, P>0.05; data not shown).
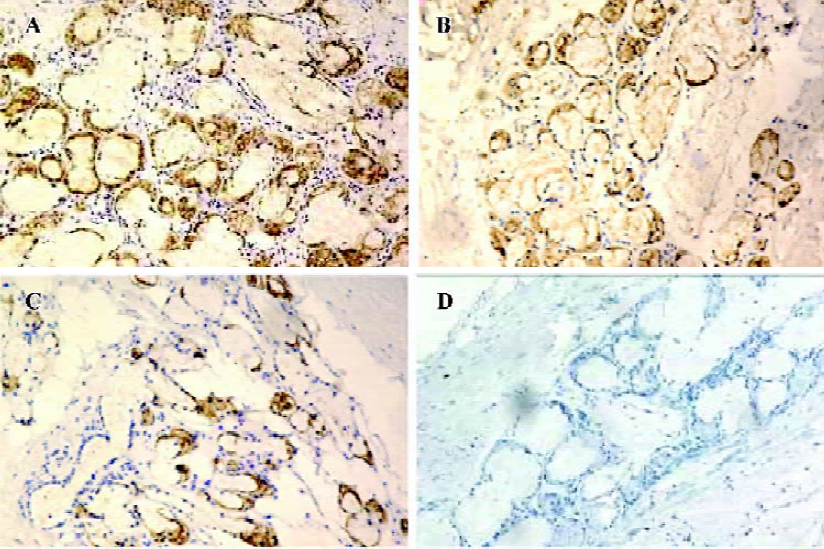
MUC5AC was expressed throughout the bronchial tissues from patients in all groups, but no positive signals were found in the lung tissue of any group. The positive signals (in brown) were expressed mainly in the cytoplasm and secondarily on the cell membrane. The COPD groups showed stronger expression and more positively-stained cells than the control group. The mean OD was 1.33±0.01 in the control group, 1.37±0.01 in the mild COPD patients, and 1.41± 0.03 in moderate and severe COPD patients; the differences were significant (P<0.01, P<0.01; Figure 4A–4C). When PBS was used instead of the AQP5 and MUC5AC antibodies, no staining resulted, confirming the specificity of the assay (Figures 3D,4D).
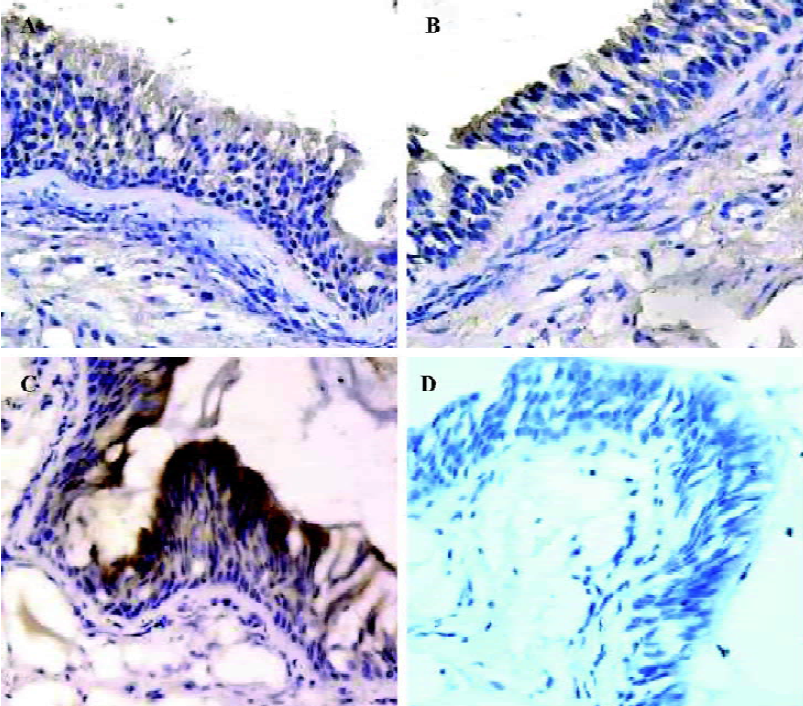
The results of the immunohistochemical staining for AQP5 and MUC5AC were used to evaluate the influence of smoking. In the COPD group, the mean OD of AQP5 was 1.31±0.02 for the ex-smokers and 1.34±0.02 for the non-smokers, and the mean OD of MUC5AC was 1.42±0.02 for the ex-smokers and 1.37±0.03 for the non-smokers. Both differences were significant (P<0.05, P<0.01), but in the control group, the mean OD of AQP5 was 1.36±0.03 for the ex-smokers and 1.35±0.02 for the non-smokers. The mean OD of MUC5AC was 1.31±0.02 for the ex-smokers and 1.34±0.02 for the non-smokers, without significant differences (P>0.05, P>0.05).
Mucin production in bronchial tissues To examine whether the decreased expression of AQP5 was associated with mucus hypersecretion, AB-PAS staining were performed. The sialic acid in the submucosal gland acinar cells was stained blue, while polysaccharide and neutral mucin were stained purple. Submucosal glands in bronchial tissues from all groups produce mucin. The ratios of the positively-stained areas in the submucosal glands to the areas of bronchial tissues in the same fields of view were 0.26±0.01 in the control group, 0.31±0.02 in the mild COPD patients, and 0.38±0.04 in the moderate and severe COPD patients; significant differences were noted (P<0.01, P<0.01; Figure 5A–5C).
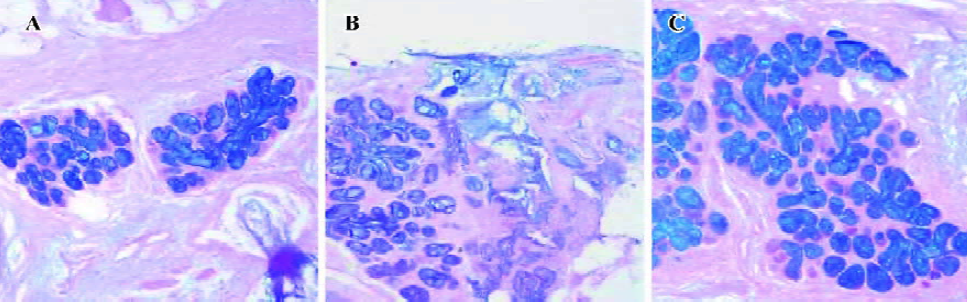
Smoking resulted in increased positively-stained areas in the submucosal glands. In the COPD group, they were 0.37±0.02 for the ex-smokers and 0.32±0.02 for the non-smokers, 0.25±0.01 for the ex-smokers and 0.26±0.01 for the non-smokers in the control group. The difference in the COPD group was significant (P<0.01), and that of the control group was to the contrary (P>0.05).
Correlation analysis The downregulation of AQP5 expression correlated significantly with mucus overproduc-tion, the data of FEV1/FVC and FEV1% predicted values, V50% predicted value, and V25% predicted value. The expression levels of MUC5AC mRNA negatively correlated with the data of FEV1/FVC and FEV1% predicted values, V50% predicted value, and V25% predicted value (Table 2).

Full table
Discussion
The discovery of the AQP family of water channel proteins has provided insight into molecular mechanisms of membrane water permeability. The specific expression pattern of AQP5 in the lungs suggests that it plays a role in the regulation of mucus secretion. In this study, we evaluated the expression of AQP5, MUC5AC, and mucin in the airways in bronchial tissues. Attenuated AQP5 mRNA expression was demonstrated in the airways of COPD patients. Con-comitantly, MUC5AC and mucus secretion increased greatly in the airways of COPD patients. Smoking attenuated the expressions of AQP5 and increased the staining of MUC5AC and mucin in submucosal glands in the COPD groups, while there were no significant differences in the control group. The downregulation of AQP5 expression correlated significantly with mucus overproduction, the data of FEV1/FVC and FEV1% predicted values, V50% predicted value, and V25% predicted value. The expression levels of MUC5AC mRNA negatively correlated with the data of FEV1/FVC and FEV1% predicted values, V50% predicted value, and V25% predicted value. These results suggest that the attenuated gene expression of AQP5 existed and was complicated by mucus hypersecretion in the airways of Chinese COPD patients. Smoking results in airway remodeling in patients with COPD.
Mucus protects the underlying airway epithelium from dehydration, pathogens, and chemical and particulate irritants, but mucus hypersecretion in the airways leads to the obstruction of airflow. Abnormal mucus secretions have been proposed to promote dysfunction of antibiosis enzymes and antibacterial peptides[7]. Submucosal glands are thought to play an important role in the regulation of mucus volume and composition, and are important in antimicrobial defense[12]. Immunocytochemistry of human and rat airways indicated the expression of AQP water channels in glandular epithelia[13,14]. In the current study, we found the expression of AQP5 at the apical membranes of cellular columnoepithelialis and submucosal glands, the sites of active mucus secretion. This confirmed the importance of AQP5 in the regulation of mucus secretion. AQP5 was also found expressed in the apical surface of type I pneumocytes[15], which involved fluid movement across epithelial and endothelial barriers occurring in interstitial and alveolar pulmonary edema. Song et al[16] showed that gland fluid from AQP5-null mice had a substantially higher protein concentration and mildly elevated chloride concentration compared with fluid from wild-type mice. Ma et al[17] found that AQP5 deletion resulted in impaired secretion of fluid from salivary glands. Furthermore, Krane et al[18] showed that the decreased rate of fluid secretion onto the airway surface in AQP5-null mice could increase airway reactivity in response to bronchoconstricting agents. We found for the first that the expression of AQP5 was attenuated throughout the bronchial tissues from patients with COPD. Moreover, the attenuated expression of AQP5 was related to the severity of airflow obstruction. Thus, AQP5 deletion may be one of the causes of hypertonic mucus and airway hyperresponsiveness in patients with COPD.
Smoking is a main factor in the pathogenesis of COPD. We evaluated the influence of smoking on the expressions of AQP5, MUC5AC, and mucus in submucosal glands of ex-smokers and non-smokers in the control and COPD groups, respectively. Interestingly, smoking attenuated the expressions of AQP5 and increased the staining of MUC5AC and mucin in submucosal glands in the COPD group, while there were no significant differences in the control group. Although all ex-smokers had given up smoking for more than 5 years, the influence of smoking continued to exist in patients with COPD. This might be the result of airway remodeling in these patients, but it seemed that smoking did not play any role in the control group. Only 15% of cigarette smokers develop clinically-significant COPD[19]. Whether AQP5 gene polymorphisms are involved in the pathogenesis of COPD needs further investigation.
In COPD patients, the impaired hypertonic mucus by AQP5 deletion may be a functional rather than anatomical defect as we found that greater areas of submucosal glands were noted in the COPD group than in the control group, and the expression levels of AQP5 mRNA significantly correlated with the patients’ data of FEV1/FVC and FEV1% predicted values, V50%, predicted value, and V25% predicted value. The AQP5 deletion and MUC5AC increase may reflect, at least in part, the airflow obstruction. Towne et al[20] showed that tumor necrosis factor-α (TNF-α) inhibited the expression of AQP5 mRNA and protein in mouse lung epithelia cells. Recently, Lora et al[21] demonstrated that TNF-α triggered mucus production in the epithelium airways through an IκB kinase β-dependent mechanism. So TNF-α may regulate mucus secretion in 2 ways. In a cultured human airway epithelial cell line, the expression of AQP3 was upregulated with the treatment of corticosteroid[22], although the use of corticosteroids in stable COPD patients is still controversial. It will be helpful to investigate the possible regulators, including TNF-α and corticosteroid, for the gene expression of AQP5, MUC5AC, and mucin secretion in the epithelium airways of COPD patients.
In summary, the current study demonstrates that attenuated gene expression of AQP5 existed and was complicated by mucus hypersecretion in the airways of Chinese COPD patients. The results suggest the importance of AQP5 for fluid secretion of submucosal gland airways. Treatment targeting AQP5 may be an effective approach to COPD therapy.
References
- Wang F, Feng XC, Li YM, Yang H, Ma TH. Aquaporins as potential drug targets. Acta Pharmacol Sin 2006;27:394-401.
- Verkman AS, Matthay MA, Song Y. Aquaporin water channels and lung physiology. Am J Physiol Lung Cell Mol Physiol 2000;278:L867-79.
- Verkman AS. Aquaporin water channels and endothelial cell function. J Anat 2002;200:617-27.
- Borok Z, Verkman AS. Lung edema clearance: 20 years of progress interview: role of aquaporin water channels in fluid transport in lung and airways. J Appl Physiol 2002;93:2199-206.
- Miller AL, Strieter RM, Gruber AD, Ho SB, Lukacs NW. CXCR2 regulates respiratory syncytial virus-induced airway hyperreactivity and mucus overproduction. J Immunol 2003;170:3348-56.
- Kuperman DA, Huang X, Koth LL, Chang GH, Dolganov GM., Zhu Z, et al. Direct effects of interleukin-13 on epithelial cells cause airway hyperreactivity and mucus overproduction in asthma. Nat Med 2002;8:885-9.
- Jayaraman S, Joo NS, Reitz B, Wine JJ, Verkman AS. Submucosal gland secretions in airways from cystic fibrosis patients have normal Na+ and pH but elevated viscosity. Proc Natl Acad Sci USA 2001;98:8119-23.
- Rose MC, Piazza FM, Chen YA, Alimam MZ, Bautista MV, Letwin N, et al. Model systems for investigating mucin gene expression in airway diseases. J Aerosol Med 2000;13:245-61.
- Zuhdi Alimam M, Piazza FM, Selby DM, Letwin N, Huang L, Rose MC, et al. Muc-5/5ac mucin messenger RNA and protein expression is a marker of goblet cell metaplasia in murine airways. Am J Respir Cell Mol Biol 2000;22:253-60.
- Hoshino M, Nakamura Y, Sim JJ, Shimojo J, Isogai S. Bronchial subepithelial fibrosis and expression of matrix metalloproteinase-9 in asthmatic airway inflammation. J Allergy Clin Immunol 1998;102:783-8.
- Desseyn JL, Buisine MP, Porchet N, Aubert JP, Laine A. Genomic organization of the human mucin gene MUC5B. cDNA and genomic sequences upstream of the large central exon. J Biol Chem 1998; 273: 30 157–64.
- Boucher RC. Molecular insights into the physiology of the ‘thin film’ of airway surface liquid. J Physiol 1999;516:631-8.
- Kreda SM, Gynn MC, Fenstermacher DA, Boucher RC, Gabriel SE. Expression and localization of epithelial aquaporins in the adult human lung. Am J Respir Cell Mol Biol 2001;24:224-34.
- King LS, Nielsen S, Agre P. Aquaporins in complex tissues. I. Developmental patterns in respiratory and glandular tissues of rat. Am J Physiol 1997;273:C1541-8.
- Jiao G, Li E, Yu R. Decreased expression of AQP1 and AQP5 in acute injured lungs in rats. Chin Med J 2002;115:963-7.
- Song Y, Verkman AS. Aquaporin-5 dependent fluid secretion in airway submucosal glands. J Biol Chem 2001;276:41288-92.
- Ma T, Song Y, Gillespie A, Carlson EJ, Epstein CJ, Verkman AS. Defective secretion of saliva in transgenic mice lacking aquapo-rin-5 water channels. J Biol Chem 1999;274:20071-4.
- Krane CM, Fortner CN, Hand AR, McGraw DW, Lorenz JN, Wert SE, et al. Aquaporin-5 deficient mouse lungs are hyper-responsive to cholinergic stimulation. Proc Natl Acad Sci USA 2001;98:1059-63.
- Bascom R. Differential susceptibility to tobacco smoke: possible mechanisms. Pharmacogenetics 1991;1:101-6.
- Towne JE, Krane CM, Bachurski CJ, Menon AG. Tumor necrosis factor-α inhabits aquaporin 5 expression in mouse lung epithelial cells. J Biol Chem 2001;276:18657-64.
- Lora JM, Zhang DM, Liao SM, Burwell T, King AM, Barker PA, et al. Tumor necrosis factor-alpha triggers mucus production in airway epithelium through an IkappaB kinase beta-dependent mechanism. J Biol Chem 2005;280:36510-7.
- Tanaka M, Inase N, Fushimi K, Ishibashi K, Ichioka M, Sasaki S, et al. Induction of aquaporin 3 by corticosteroid in a human airway epithelial cell line. Am J Physiol 1997;273:L1090-5.