Protective effect of ginsenoside Rg1 on glutamate-induced lung injury1
Introduction
Glutamate, the major excitatory neurotransmitters in the mammalian central nervous system, plays an important role in many physiological functions by acting on its receptors. At the same time, abnormally intense exposure to glutamate can be neurotoxic, primarily through the overactivation of the N-methyl-D-aspartate (NMDA) subtype of glutamate receptors, a phenomenon known as excitotoxicity, which has been implicated in neuronal degeneration and loss in some acute conditions and chronic neurodegenerative diseases[1]. Recently, sufficient evidence has been demonstrated for the presence of NMDA glutamate receptors in the lungs and some other non-neuronal tissues[2]. Said et al reported that the activation of NMDA receptors in perfused, ventilated rat lungs by NMDA (1×10-3 mol/L), a synthetic agonist that selectively activates NMDA receptors, triggered acute injury, marked by high-permeability edema[2]. Our previous study demonstrated that glutamate (0.5 g/kg, ip) in vivo also provoked acute lung injury. Both injuries were attenuated by the NMDA receptor antagonist, MK801 (dizocilpine maleate), which suggests that the activation of NMDA receptors leads to acute lung injury[2]. Ginsenoside Rg1 is an important active component of ginseng and they share many pharmacological effects. It was proved that ginsenoside Rg1 has a partial neurotrophic and neuroprotective role against the toxic effects of glutamate in cultured dopaminergic cells[3], hippocampal neurons[4], and the neurons in the spinal cord culture[5]. We speculate that ginsenoside Rg1 also ameliorates glutamate-induced lung injuries. In the current study, we first investigated the in vivo effect of ginsenoside Rg1 on acute lung injury induced by glutamate to afford a theoretical basis of clinical administration of ginsenoside Rg1 for the treatment of lung diseases associated with the activation of the NMDA receptors.
Materials and methods
Materials Kunming male mice (clean grade), weighing 25±5 g, were obtained from the Experimental Animal Center of Xiangya School of Medicine, Central South University (Changsha, China). Ginsenoside Rg1 and glutamate were obtained from the School of Pharmacy, Jilin University (Jilin, China) and Sigma (St Louis, MO, USA), respectively. MS302 bioinstrumentation was from Guangzhou Long-fei-da Technology Ltd Co (Guangzhou, China). Detection kits for nitric oxide (NO), total nitric oxide synthetase (NOS), xanthine oxidase (XOD), superoxide dismutase (SOD), catalase (CAT), and malonaldehyde (MDA) were all from Nanjing Jiancheng Bioengineering Institute (Nanjing, China). All other chemicals were up to the analytical chemistry standard.
Experimental groups The animals were housed at room temperature and kept on an unlimited supply of standard diet and tap water. All studies were performed in accordance with the National Institute of Health Criteria for the Care of Laboratory Animals. The mice were randomly divided into 4 groups and were given normal saline alone (group NS), glutamate (group Glu), ginsenoside Rg1 (group Gin), or both ginsenoside Rg1 and glutamate (group Gin+Glu), respec-tively. Ginsenoside Rg1 was given intraperitoneally at a dose of 0.03 g/kg for 30 min before the injection of glutamate (0.5 g/kg, ip).
All the mice were anesthetized 2 h after the insult of glutamate; the heart rate (HR) and breathing rate (BR) were recorded by MS302 bioinstrumentation. After that, the mice were sacrificed immediately for determinations as below.
Lung wet weight/body weight (LW/BW), lung wet weight/lung dry weight (W/D) After sacrificing each mouse by femoral artery bleeding, both lungs were carefully taken out and weighed immediately in a weighing bottle. Then the lungs were dried in a microwave oven with progressively increasing power[6], cooled in the bottle, and weighed again until no further weight loss occurred. After that, the weight deducted from the bottle weight was reported as dry weight.
Histological examination After sacrifice, the lung samples were immersed in 10% buffered formalin. Formalin-preserved specimens were then embedded in paraffin, cut into 5 µm thick sections, and stained with hematoxylin and eosin for histological examination. This experiment was repeated 5 times.
Analysis of lung homogenates
Preparation of lung homogenate samples In some experiments, the left lung was removed, washed in 0.9% NaCl, and homogenized (1/10, w/v) in a glass homogenizer in ice-cold NaCl solution. The homogenates were centrifuged, and the supernatant was stored at 4 °C and used for the following determination. All biochemical parameters in the homogenates were studied on the same day. Tissue concentrations of the respective parameters were related to the protein content in the samples. The protein concentration was determined by the Lowry method[7].
NO and NOS activity Tissue concentrations of NO were measured through its stable metabolites nitrate and nitrite. Nitrate was first reduced by nitrate reductase to nitrite and then nitrite was determined spectrophotometrically at 550 nm by the Griess reaction using commercial kits. NOS activities were measured spectrophotometrically at 530 nm by enzymatic methods using commercial kits.
XOD activity determination XOD activity was assayed spectrophotometrically at 37 °C with the corresponding substrate using commercial kits, and the red-violet product of the reaction was measured in the visible range at 530 nm.
Antioxidase activity measurement Tissue SOD activities were determined by the inhibition of nitroblue tetrazolium (NBT) reduction with xanthine/XOD used as a superoxide generator using commercial kits. One unit of SOD was defined as the amount of protein that inhibits the rate of NBT reduction by 50%.
CAT activity was measured by the breakdown of hydrogen peroxide catalysed by catalase enzymes using commercial kits.
MDA determination MDA, an end product of fatty acid peroxidation, was measured in lung homogenates by the thiobarbituric acid reactivity assay using commercial kits.
Statistical analysis All statistical analyses were carried out using SPSS statistical software (SPSS for Windows version 11.0, SPSS Inc, Chicago, USA,). Data were analyzed by ANOVA, and the Student Newman-Keuls method was used to estimate the level of significance of differences between means. The data are expressed as mean±SD. The criterion for significance was P<0.05.
Results
Heart rate and breathing rate Glu (0.5 g/kg) treatment elevated HR and BR (P<0.01). Gin (0.03 g/kg) pretreatment abolished the enhancing effect of Glu on HR and BR (P<0.01; Table 1).
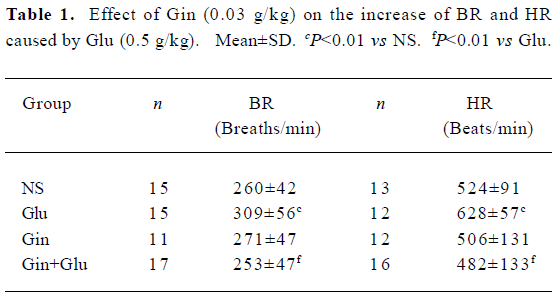
Full table
LW/BW and W/D The addition of Gin (0.03 g/kg) 30 min before Glu treatment prevented an increase of lung water induced by Glu (0.5 g/kg). Both LW/BW and W/D of the Glu group were elevated (P<0.05), while those of group Gin+Glu decreased to the same level as group NS (P<0.01; Table 2).
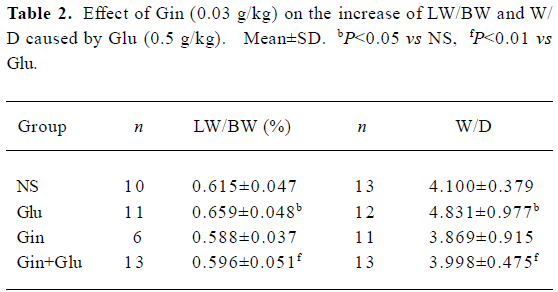
Full table
Histological examination Significant lung injury was present in the animals of the Glu group compared with the NS group. There was edematous thickening of the alveolar walls with occasional alveoli containing coagulated edema fluid. The alveolar interstitium showed marked sequestration of inflammatory cells, which were predominantly neutrophils. Gin treatment reduced lung injurious pathology change caused by the administration of Glu (Figure 1).
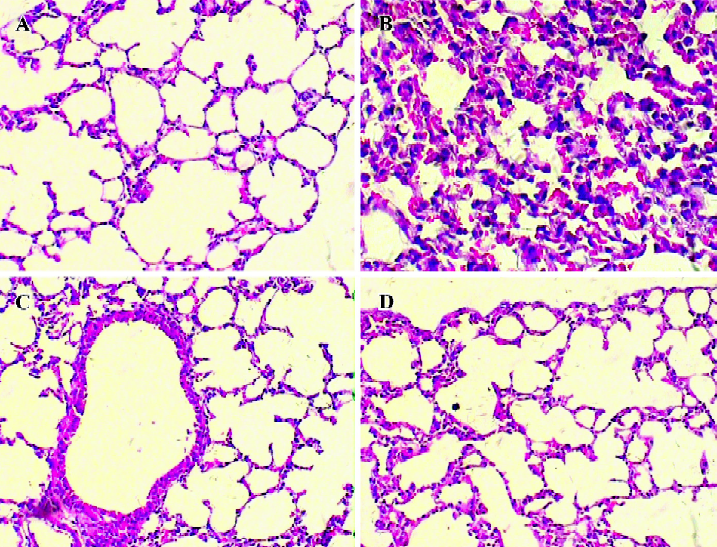
Activity of XOD, SOD, CAT, and levels of MDA in lung tissues Treatment of Glu (0.5 g/kg) increased XOD activity (P<0.01) and MDA (P<0.05) in lung tissues. Gin (0.03 g/kg) abolished the activation of XOD and increased MDA levels induced by Glu (Table 3).
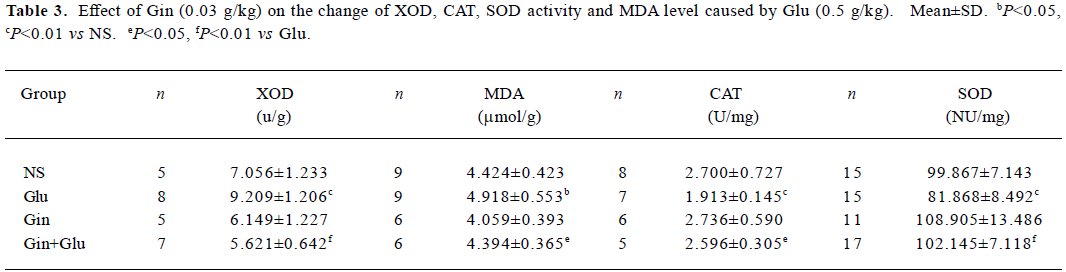
Full table
The lung SOD and CAT activities in the Glu group were lower than in the NS group (P<0.01), and SOD and CAT activities in the Gin+Glu group increased to the same level as group NS. No significant difference of tissue SOD and CAT activities was observed between the Gin and NS groups (Table 3).
NOS activity and NO production The injection of Glu (0.5 g/kg) led to the activation of NOS, following the elevation of NO content (P<0.01). With the pretreatment of Gin (0.03 g/kg), NOS activity and the NO level decreased to the control level (Table 4).
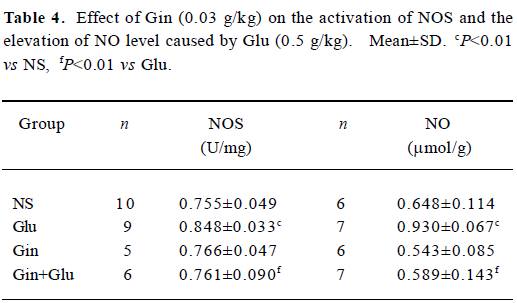
Full table
Discussion
Acute respiratory distress syndrome (ARDS) is a common clinical syndrome. The pathogenesis of ARDS has been undefined up to now. The neutrophils sequestration and activation of neutrophils and alveolar macrophages play key roles in the development of ARDS[8]. Neutrophils[9] and macrophages[10] can release glutamate when stimulated. The glutamate concentrations in the pulmonary veins of rat model septicemia are higher than in the pulmonary artery[11]. Our previous study demonstrated that the administration of glutamate via intraperitoneal injection provoked acute lung injury characterized by edema, neutrophil sequestration, and increased alveolar-microvascular membrane permeability. This injury was attenuated by MK801, a NMDA receptor antagonist, which suggests that glutamate in vivo causes lung injury through the activation of NMDA receptors. These results raise the possibility of new therapeutic uses of MK-801 which have potential protective effects against glutamate-induced toxicity in related lung impairment. Although there is promising therapeutic potential of MK-801, the delivery remains a challenge due to its side effects[12].
Ginseng is the best-known and most popular herbal medicine. It serves as an important component of many Chinese prescriptions for thousands of years and is now popular in the world as a natural medicine. The molecular components responsible for ginseng actions are ginsenosides, which are also known as ginseng saponins[5]. Ginsenoside Rg1 is an important active component of ginseng and has been reported to protect neurons from excitotoxicity induced by glutamate[3–5]. Several recent studies have reported that ginsenoside Rg1 has some beneficial actions on lungs. It inhibits the releases of histamine and leukotrienes during the activation of guinea pig lung mast cells[13]. Ginsenoside Rg1 can enhance the synthesis of pulmonary surfactant of cultured rat lung explants[14]. Whether ginsenoside Rg1 has protective actions on the glutamate-induced lung injury has not been reported.
The simplest way to evaluate edema formation in lungs is to use a gravimetric approach. Because inbred strains have relatively uniform LW/BW ratios between animals at any given age, one can potentially compare LW/BW in treatment groups to that predicted based on body weight. The measure of W/D is a more useful tool since it accounts for changes in lung dry mass as well. This research shows that ginsenoside Rg1 attenuated the glutamate-induced elevation of LW/BW, W/D, HR, BR, and the histology changes of lung tissues. This study first proved that ginsenoside Rg1 could attenuate glutamate-induced lung injury in vivo.
There is general agreement about the mechanism of neuroexcitotoxicity induced by glutamate that it is Ca2+-dependent. It is also generally accepted that the NMDA receptors play a key role in mediating glutamate toxicity owing to its high Ca2+ permeability[1]. Excessive Ca2+ loading exceeding the capacity of Ca2+-regulating mechanisms could activate several cell death-related genes and pathways. These include the calcium-dependent activation of XOD[15]. XOD is known to generate deleterious oxygen-free radicals such as superoxide, and hydroxyl radicals such as hypoxanthine are metablized to uric acid in the final steps of purine degradation[16]. In this study, the impairment of SOD and CAT was also noted in the lungs of of the mice in the Glu group. SOD converts superoxide radical to H2O2, which is in turn broken down to water and oxygen by CAT[17]. Therefore XOD, SOD, and CAT play important roles in the balance between the oxidation and antioxidation of the organism[17]. The activation of XOD and the impairment of SOD and CAT lead to overproduction of reactive oxygen species. Higher doses of oxygen-derived free radicals destabilize cell membranes, increase membrane permeability, oxidize cyto-solic, and membrane-bound proteins[18]. These alterations of cellular function impact cellular defense mechanisms and membrane integrity, contributing to pulmonary edema accumulation. The augmentation of antioxidant defense mechanisms attenuates lung injury. It has been reported that ginsenoside Rg1 inhibits lipid peroxidation within the liver and brain by upregulating catalases[19] and suppresses oxidative stress in neurons by the activation SOD[20]. Our data indicates that the administration of ginsenoside Rg1 reversed the activation of XOD and reduced the impairment of SOD and CAT. As a result, cell injury mediated by free radicals was relieved in the Gin+Glu group, which was demonstrated by the drop in levels of MDA, an end product of fatty acid peroxidation. Ginsenosides are reported for the inhibition of Ca2+ over-influx into the mitochondria of the surviving cells, thus lowering free radical production by depolarized mitochondria and increasing energy production crucial for cell survival[3]. Additionally, the modulation effect of ginsenoside Rg1 on NMDA receptors binding in the rat brain was also reported[21]. Whether ginsenoside Rg1 influences the binding of NMDA receptors or Ca2+ over-influx in this model needs further research.
Evidence has been presented for a key mediator role for NO in glutamate-stimulated neurotoxicity[22]. Based on the research of the isolated perfused and ventilated rat lung, Said et al reported that as in central neuronal glutamate toxicity, lung injury caused by the NMDA in the perfusate was NMDA receptor-mediated and NO dependent, and was associated with the increased production of NO[2]. The present study shows that glutamate in vivo leads to an increase in NOS activity and NO generation in mouse lungs, which suggests NO may contribute to lung injury caused by glutamate in vivo through some mechanism. NO, in high enough concentrations produced in pathological conditions, is known to effectively compete with SOD for superoxide[23]. When NO and superoxide anion are present in large amounts, peroxynitrite (ONOO–), a potent oxidant and nitrating reagent, is produced[24]. However, glutamate-induced NO generation by the upregulation of NOS activity may also promote transcription and the translation of numerous inflammatory cytokines, and as a result, more neutrophils and macrophages are recruited and activated, which produce a burst of free radicals[25–27]. Although these possible alterations could explain cell injury and the dysfunction of the alveolar capillary-epithelial permeability barrier, which lead to the leak of plasma into the pulmonary interstitium and alveolar spaces resulting in high-permeability pulmonary edema in the present study, further evidence is still required. In this study, pretreatment with ginsenoside Rg1 almost completely inhibited an increase of NOS activity, and subsequently NO generation and eventually relieved inflammatory responses and free-radical-mediated lipid peroxidation. Three isoforms of the enzyme responsible for NO production, NOS have been described: neuronal (nNOS, NOS I), inducible (iNOS, NOS II), and endothelial (eNOS, NOS III). All 3 isoforms of NOS are expressed in lungs[28]. The NOS isoenzymes and location involved in the protective effects of ginsenoside Rg1 against glutamate-induced lung injury require further investigation in the future.
In summary, the present study indicates that ginsenoside Rg1, an extract of ginseng, could attenuate glutamate-induced lung injury by interrupting the generation of reactive oxygen species and NO. Although other studies have demonstrated beneficial effects of ginsenoside Rg1 on neurotoxicity, this is the first study to our knowledge that examined the effect of ginsenoside Rg1 on the glutamate-induced impairment of pulmonary tissue in vivo. Given that ginseng has only minor side effects in animals and humans[5], our research might offer a potential means to rescue or protect lungs from some diseases associated with glutamate toxicity.
References
- Avignone E, Frenguelli BG, Irving AJ. Differential responses to NMDA receptor activation in rat hippocampal interneurons and pyramidal cells may underlie enhanced pyramidal cell vulnerability. Eur J Neurosci 2005;22:3077-90.
- Said SI, Berisha HI, Pakbaz H. Excitotoxicity in the lung: N-methyl-D-aspartate-induced, nitric oxide-dependent, pulmonary edema is attenuated by vasoactive intestinal peptide and by inhibitors of poly (ADP-ribose) polymerase. Proc Natl Acad Sci USA 1996;93:4688-92.
- Radad K, Gille G, Moldzio R, Saito H, Rausch WD. Ginsenosides Rb1 and Rg1 effects on mesencephalic dopaminergic cells stressed with glutamate. Brain Res. 2004;1021:41-53.
- Liu M, Zhang JT. Protective effects of ginsenoside Rb1 and Rg1 on cultured hippocampal neurons. Yao Xue Xue Bao 1995;30:674-8. Chinese..
- Liao B, Newmark H, Zhou R. Neuroprotective effects of ginseng total saponin and ginsenosides Rb1 and Rg1 on spinal cord neurons in vitro. Exp Neurol 2002;173:224-34.
- Peterson BT, Brooks JA, Zack AG. Use of microwave oven for determination of postmortem water volume of lungs. J Appl Physiol 1982;52:1661-3.
- Hartree EF. Determination of protein: a modification of the Lowry method that gives a linear photometric response. Anal Biochem 1972;48:422-7.
- Esper AM, Martin GS. Evolution of treatments for patients with acute lung injury. Expert Opin Investig Drugs 2005;14:633-45.
- Collard CD, Park KA, Montalto MC, Alapati S, Buras JA, Stahl GL, et al. Neutrophil-derived Glutamate Regulates Vascular Endothelial Barrier Function. J Biol Chem 2002;277:14801-11.
- Jiang ZG, Piggee C, Heyes MP, Murphy C, Quearry B, Bauer M, et al. Glutamate is a mediator of neurotoxicity in secretions of activated HIV-1-infected macrophages. J Neuroimmunol 2001;117:97-107.
- Ardawi MS. Glutamine and alanine metabolism in lungs of septic rats. Clin Sci (Lond) 1991;81:603-9.
- Danilczuk Z, Ossowska G, Lupina T, Cieslik K, Zebrowska-Lupina I. Effect of NMDA receptor antagonists on behavioral impairment induced by chronic treatment with dexamethasone. Pharmacol Rep 2005;57:47-54.
- Ro JY, Kim KH. The effects of single component of ginsenosides on the mechanism of mediator release in the allergic hypersen-sitivity. Korean J Pharmacol 1994;30:243-54.
- Luo ZQ, Feng DD, Yue SJ. Effect of shenfu injection on the synthesis of pulmonary surfactant in cultured lung explants. Bull Hu-nan Med Univ 2003;28:137-40. Chinese..
- Dutrait N, Culcasi M, Cazevieille C, Pietri S, Tordo P, Bonne C, et al. Calcium-dependent free radical generation in cultured retinal neurons injured by kainate. Neurosci Lett 1995;198:13-6.
- Maia L, Vala A, Mira L. NADH oxidase activity of rat liver xanthine dehydrogenase and xanthine oxidase-contribution for damage mechanisms. Free Radic Res 2005;39:979-86.
- Georgieva NV, Koinarski V, Gadjeva V. Antioxidant status during the course of Eimeria tenella infection in broiler chickens. Vet J 2005. Epub ahead of print.
- Vadasz I, Morty RE, Olschewski A, Konigshoff M, Kohstall MG, Ghofrani HA, et al. Thrombin impairs alveolar fluid clearance by promoting endocytosis of Na+,K+-ATPase. Am J Respir Cell Mol Biol 2005;33:343-54.
- Deng HL, Zhang JT. Anti-lipid peroxilative effect of ginsenoside Rb1 and Rg1. Chin Med J (Engl) 1991;104:395-8.
- Chen XC, Zhou YC, Chen Y, Zhu YG, Fang F, Chen LM. Ginsenoside Rg1 reduces MPTP-induced substantia nigra neuron loss by suppressing oxidative stress. Acta Pharmacol Sin 2005;26:56-62.
- Kim HS, Hwang SL, Nah SY, Oh S. Changes of [3H]MK-801, [3H]muscimol and [3H]flunitrazepam binding in rat brain by the prolonged ventricular infusion of ginsenoside Rc and Rg1. Pharmacol Res 2001;43:473-9.
- Dawson VL, Dawson TM, Bartley DA, Uhl GR, Snyder SH. Mechanisms of nitric oxide-mediated neurotoxicity in primary brain cultures. J Neurosci 1993;13:2651-61.
- Beckman JS, Koppenol WH. Nitric oxide, superoxide, and peroxynitrite: the good, the bad, and the ugly. Am J Physiol 1996;271:C1424-37.
- Wizemann TM. Production of nitric oxide and peroxynitrite in the lung during acute endotoxemia. J Leukoc Biol 1994;56:759-68.
- Kaminska B, Gaweda-Walerych K, Zawadzka M. Molecular mechanisms of neuroprotective action of immunosuppressants — facts and hypotheses. J Cell Mol Med 2004;8:45-58.
- Glezer I, Munhoz CD, Kawamoto EM, Marcourakis T, Avellar MC, Scavone C. MK-801 and 7-Ni attenuate the activation of brain NF-kappa B induced by LPS. Neuropharmacology 2003;45:1120-9.
- Deckel AW. Nitric oxide and nitric oxide synthase in Huntington’s disease. J Neurosci Res 2001;64:99-107.
- Fagan KA, Tyler RC. Relative contributions of endothelial, inducible, and neuronal NOS to tone in the murine pulmonary circulation. Am J Physiol Lung Cell Mol Physiol 1999;277:L472-8.