Expression of vascular endothelial growth factor in cultured human dental follicle cells and its biological roles1
Introduction
Tooth eruption is a complex and tightly regulated process involved in both odontogenic and osteogenic cells. The dental follicle, a loose connective tissue sac surrounding each unerupted tooth, has been demonstrated to play an important role in this process[1–3]. A major reason for its requirement is that it provides the eruption molecules, such as colony-stimulating factor-1 and monocyte chemotactic protein-1 to initiate and regulate osteoclastogenesis[4–6].
Vascular endothelial growth factor (VEGF), also known as vascular permeability factor or vasculotropin, is a main regulator of blood vessel formation during embryogenesis and a potent inducer of neovascularization during adult life[7]. The angiogenic property of VEGF has been attributed to its several distinct functions. VEGF is an endothelial cell mitogen and permeability-enhancing factor that influences the egress of plasma proteins and cells that both directly and indirectly stimulate angiogenesis[8,9]. VEGF may also act as a survival factor for endothelial cells[10,11]. VEGF mediates its activity mainly through specific binding to 2 tyrosine kinase receptors, the fms-like tyrosine kinase [Flt-1, VEGF receptor (VEGFR)-1] and the kinase insert domain-containing receptor (KDR, VEGFR-2). Ligand-receptor interaction induces the activation of the tyrosine kinase domain of the VEGFR, which finally leads to the activation of intracellular signaling transduction pathways that are involved in regulating cellular proliferation and survival, such as the Raf/mitogen-activated protein kinase-extracellular signal-regulated kinase (MEK)/extracellular signal-regulated kinase (ERK), and the phosphatidylinositol 3' kinase/protein kinase B pathways[12,13]. Agents directed either against VEGF or VEGFR have been developed and anti-angiogenic therapies based on the inhibition of VEGF/VEGFR signalling have been reported to be powerful clinical strategies in oncology and ophthalmology[14,15].
The role of VEGF in bone remodeling has also been extensively studied during the past decade. Many authors demonstrated that VEGF was implicated in normal physiological processes such as osteoclastogenesis and bone resorption[16–19]. VEGF has also been shown to induce osteoblast chemotaxis, proliferation, differentiation, and 3',5'-cyclic adenosine monophosphate (cAMP) production[20–22], indicating that it might be involved in bone formation. Since substantial evidence indicates that VEGF is involved in bone remodeling, which is required for tooth eruption, the question as to whether VEGF is expressed in dental follicle cells is particularly worthy of attention. Recently, Wise et al originally reported that VEGF was expressed in cultured rat dental follicle cells. VEGF was also regarded as one of the molecules responsible for the minor burst of osteoclastogenesis at d 10 in the rat first mandibular molar, indicating it might be an important molecule involved in tooth eruption[23]. However, whether VEGF can be expressed in human dental follicle cells (HDFC) is not clear. Moreover, previous studies have focused on the role of VEGF in a signaling cascade that initiated tooth eruption. Its biological effect on dental follicle cells has never been reported. We conducted an in vitro study to determine whether VEGF is normally expressed in cultured HDFC and to examine the roles of VEGF in the proliferation, differentiation, and apoptosis of HDFC.
Materials and methods
Reagents Dulbecco’s modified Eagle’s medium (DMEM) was purchased from Hyclone Lab (Logan, UT, USA). Fetal bovine serum (FBS) and RPMI-1640 medium were purchased from Gibco BRL (Grand Island, NY, USA). The rabbit polyclonal antibody against human VEGF and non-immune normal rabbit IgG were purchased from Santa Cruz Biotechnology (Santa Cruz, CA, USA). Goat anti-rabbit IgG conjugated with horseradish peroxidase and 3-(4,5-dimethylthiazol-2-yl)-2,5-diphenyltetrazolium bromide (MTT) were purchased from Sigma-Aldrich (St Louis, MO, USA). The Quantikine human VEGF ELISA kit was purchased from R&D Systems (Minneapolis, MN, USA). Trizol was purchased from Invitrogen (Carlsbad, CA, USA). The First-strand cDNA synthesis kit was purchased from Toyobo (Osaka, Japan). Premix Taq (Ex Taq version) was purchased from TaKaRa (Otsu, Shiga, Japan). The agarose gel was purchased from Amresco (Solon, OH, USA). VEGF was purchased from PeproTech (Rocky Hill, NJ, USA). 2-(2-amino-3-methoxy-phenyl)-4H-1-benzopyran-4-one (PD98059) and 1,4-diamino-2,3-dicyano-1,4-bis(2-aminophenylthio) butadiene (U0126) were purchased from Calbiochem (San Diego, CA, USA). The AnaSpec EnzoLyte pNPP alkaline phosphatase assay kit was purchased from AnaSpec (San Jose, CA, USA). The BCA protein assay kit was purchased from Pierce (Rockford, IL, USA). The Annexin V-FITC apoptosis detection kit was purchased from Biovision (Mountain View, CA, USA).
Cell culture The human dental follicle was separated from an impacted mandibular third molar extracted for orthodontic reasons from a 12-year-old boy suffering class III malocclusion. Informed written consent was obtained from the boy and his parents. The study protocol was approved by the medical ethic committee of Fourth Military Medical University. The dental follicle samples were placed in the DMEM supplemented with 100 kU/L penicillin and 100 mg/L streptomycin. The culture took place within 2 h after the collection.
The HDFC were cultured as previously described by Morsczeck et al with minor modifications[24]. Briefly, after the dental follicle was separated, the surfaces of the follicle tissues were cleaned and minced by using a sterilized scalpel; the dental papilla tissue was discarded. Tissues were digested in a solution of 100 U/L collagenase type I for 1 h at 37 °C. Minced and digested tissues of dental follicle explants were seeded into 6-well plates in DMEM containing 10% FBS, 0.1 g/L sodium pyruvate, 100 mg/L streptomycin, and 100 kU/L penicillin at 37 °C in a humidified atmosphere with 5% CO2. The single cell attached to the plastic surface remained, while non-adherent cells were removed by changing the medium every 3 d. Confluent cells were collected by trypsinization and were subcultured. The HDFC between the third and seventh passage were used in this study.
Human gastric cancer cell line SGC7901, which is known to express VEGF, was used in some experiments as a reference cell[25,26]. SGC7901 cells were obtained from the Institute of Digestive Diseases of Fourth Military Medical University. The cells were cultured as previously described by Meng et al[25].
Immunocytochemistry staining for VEGF The HDFC and SGC7901 cells (that served as a positive control) were cultured on coverslips for 2 d and then transferred into serum-free medium for 5 h. Then the cells were immediately fixed in 10% neutral-buffered formalin for 5 min and permeabilized with 0.2% Triton X-100 for 5 min. Thereafter, immunocytochemistry assay was performed right away. After rinsing with phosphate buffered saline (PBS), the cells were incubated in 3% H2O2 for 5 min and then treated with a blocking agent of 10% goat serum for 30 min. For immunostaining, the cells were incubated with 5 mg/L rabbit polyclonal antibody to human VEGF at 4 °C overnight. Goat anti-rabbit IgG conjugated with horseradish peroxidase was used as a secondary antibody for 1.5 h, and colour development was visualized with 3',3'-diaminobenzidine tetrahydrochloride. The nuclei were slightly counterstained with hematoxylin. The HDFC, in which the primary antibody was replaced with the same concentration of non-immune normal rabbit IgG, were used as a negative control.
VEGF detection using ELISA The HDFC were seeded into 24-well plates at a density of 2×104/mL in DMEM containing 5% FBS (1 mL/well). After 24 h of incubation, the medium was replaced by 1 mL fresh DMEM containing 5% FBS. When the culture reached 80% confluence, the medium was replaced with 1 mL fresh serum-free DMEM containing 0.3% bovine serum albumin (BSA) and the cells were further incubated for 24 h. Then the cell-free medium was collected and stored at -20 °C until assayed.
VEGF in the culture supernatant was measured by sandwich ELISA. Briefly, 200 µL of cell culture supernate samples diluted in 50 µL buffer solution or serially diluted standard solution (recombinant human VEGF) were added to a 96-well microtiter plate precoated with a mouse anti-human VEGF monoclonal antibody and incubated at room temperature for 2 h. After washing, 200 µL of the secondary antibody solution, a VEGF-specific polyclonal goat antibody, was incubated for 2 h at room temperature. After 3 washes, 200 µL of substrate solution was added to each of the wells. After incubation at room temperature for 20 min (to protect it from light), 50 µL of stop solution was added. The absorbance of each well was detected at 490 nm with the correction wavelength set at 570 nm with a microspectrophotometer (Bio-Tek, Winooski, VT, USA). Recombinant human VEGF diluted in calibrator diluent was used as a calibration standard, ranging from 7.8 ng/L to 2000 ng/L. A standard curve was drawn by plotting absorbance versus the concentration of recombinant human VEGF. The concentration of the VEGF protein was calculated according to the standard value.
RNA extraction and RT-PCR The total RNA was extracted from the cultured HDFC or SGC7901 cells (that served as a positive control) with Trizol. The pellet of the total RNA was washed briefly with 75% ethanol, resuspended in 20 µL diethylpyrocarbonate (DEPC)-treated water, and stored at -80 °C. The total RNA concentration was determined by measuring the optical density at 260 nm with a microspectro-photometer. The ratio of A260/A280 was greater than 1.8.
For each reverse transcription reaction, 1 µg total RNA was mixed with first-strand buffer, dNTP (1 mmol/L), oligo dT (0.5 µmol/L), RNase inhibitor (500 kU/L), ReverTra Ace reverse-transcriptase (5000 kU/L), and RNase-free water to make a total reaction volume of 20 µL for the generation of the first-strand cDNA. The reactions were carried out at 42 °C for 20 min, 99 °C for 5 min, and chilled to 4 °C for 5 min. The PCR primers were designed based on the published human overall VEGF and GAPDH mRNA sequences and were commercially synthesized by TaKaRa (Japan)[27,28]. Specifically, the overall VEGF forward primer was 5'-TCTT-GGGTGCATTGGAGCCTC-3' and the reverse primer was 5'-AGCTCATCTCTCCTATGTGC-3', yielding an amplicon of 349 bp. The GAPDH forward primer was 5'-ACCACAGTCCA-TGCCATCAC-3' and the reverse primer was 5'-TCCACCA-CCCTGTTGCTGTA-3', yielding an amplicon of 452 bp. For each PCR, 1 uL of template cDNA generated in the above RT reaction was mixed with 0.5 µmol/L each primer, 25 kU/L premix Taq mixture, and distilled water to make a total reaction volume of 20 µL. A total of 35 cycles of PCR was performed with the amplification protocol of 94 °C for 30 s (denaturation), 55 °C for 30 s (annealing), and 72 °C for 45 s (extension). PCR was completed by a final extension at 72 °C for 10 min. For the loading controls, identical amplification procedures were done with GAPDH primers. Amplified products were separated by electrophoresis in a 1.5% agarose gel and visualized with ethidium bromide staining under UV light.
In vitro biological activity detection
Incubation experiments For the cell proliferation and ALP activity assays, the HDFC were seeded into 96-well plates at a density of 2×104/mL (unless otherwise stated) in DMEM containing 5% FBS (100 µL/well). After 24 h incubation, the medium was replaced by 100 µL serum-free DMEM containing 0.3% BSA for the designated studies. For the concentration-dependent studies, the cells were incubated with the medium plus various concentrations of VEGF for 3 d. For the time-course studies, the cells were incubated with the medium in the presence or absence of 100 µg/L VEGF for 0, 1, 3, 5, and 7 d (the medium was changed every 4 d). To determine whether the mitogen-activated protein kinase (MAPK) signaling pathway was involved in the VEGF-mediated HDFC proliferation, the cells were evenly distributed into seven groups and incubated with the reagents as follows respectively: 0 µg/L VEGF, 50 µmol/L PD98059, 50 µmol/L U0126, 100 ug/L VEGF with the pre-addition of 50 µmol/L PD98059 for 30 min, 100 µg/L VEGF with the pre-addition of 50 µmol/L U0126 for 30 min, 100 µg/L VEGF with the pre-addition of both 50 µmol/L PD98059 and 50 µmol/L U0126 for 30 min, and 100 µg/L VEGF alone. Cell proliferation was measured after 3 d of incubation with these reagents. In the groups for of the cell proliferation assay, each group had 8 replications, while each group for the determination of ALP activity had 5 replications.
For the Annexin V/propidium iodide (PI) staining assay, the HDFC were seeded into 75 cm2 T-flasks at a density of 2×104/mL in DMEM containing 5% FBS (12 mL/T-flask). One day before a given experiment, the medium in each T-flask was replaced with 12 mL fresh DMEM containing 5% FBS. On the day of the treatment, the cells were starved in 12 mL serum-free DMEM containing 0.3% BSA for 5 h. Then the HDFC were incubated with the medium in the presence of VEGF (100 µg/L) as the experimental groups or in the absence of VEGF as the controls for 3 d. The experiments were replicated 3 times.
MTT assay for cell proliferation determination At the end of the culture period, 20 µL MTT stock solution (5 g/L) was added into each well and incubated for a further 4 h. Then the supernatant was removed and 150 µL DMSO was added. After shaking at room temperature for 10 min, the absorbance of each well was detected at 490 nm with a microspectrophotometer.
Determination of ALP activity At the end of the culture period, the HDFC were gently washed twice with 1×lysis buffer and scraped immediately upon the addition of 0.2% Triton X-100 (50 µL/well). After incubation at 4 °C for 10 min under agitation, the cell suspension was centrifuged at 2500×g for 10 min at 4 °C. Then the supernatant was collected for the alkaline phosphatase (ALP) assay according to the EnzoLyte pNPP alkaline phosphatase assay kit instructions. 50 µL of the supernatant and 50 µL of pNPP reaction mixture were mixed and incubated at 37 °C for 30 min. The reaction was arrested by the addition of 50 µL stop solution. After shaking on a plate shaker for 1 min, the absorbance of each well was detected at 405 nm with a microspectrophotometer. The cellular protein concentration in the supernatant was determined according to BCA protein assay kit instructions. The absorbance was measured at 560 nm. The percentages of changes of ALP activity with respect to the value found in the control were calculated according to the formula: M=value of absorbance at 405 nm/value of absorbance at 560 nm. The percentage of change=[(M of the testM of the control)/M of the control]×100[29].
Annexin V/PI staining assay After 3 d of incubation, the cells were harvested. The single cell suspension samples (approximately 5×105 cells in each sample) were analyzed within 2 h. The procedure was done according to Annexin V-FITC apoptosis detection kit protocol. In brief, the HDFC were washed twice with PBS. Then the cells were resuspended in binding buffer (500 µL). After that, 5 µL Annexin V-FITC and 5 µL PI were added to each sample and incubated at room temperature for 5 min in the dark. Then the stained cells were analyzed by a flow cytometer (Beckman-Coulter, Fullerton, CA, USA).
Statistical analysis All the experiments were replicated at least 3 times. All numerical results were expressed as mean±SD. Statistical analysis was carried out using SPSS 10.0 software package for windows (SPSS Inc, Chicago, IL, USA). The statistical significance between the 2 groups was determined by Student’s t-test. The significant level was set at P<0.05.
Results
Immunostaining, ELISA, and RT-PCR Immunostaining for VEGF revealed that it was localized in cultured HDFC. The stain was more evident in the perinuclear region of the cytoplasm (Figure 1A). The negative control in which the primary antibody was replaced with non-immune normal rabbit IgG did not stain (Figure 1B).
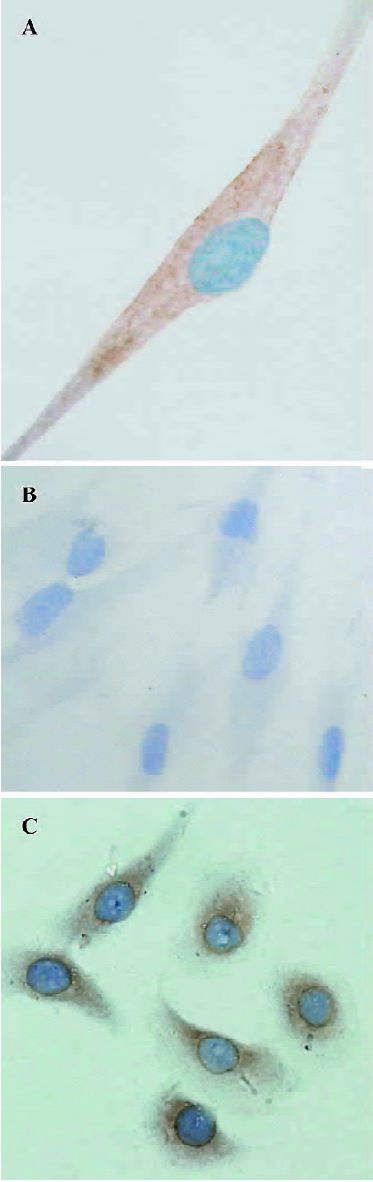
After 24 h incubation, the concentration of VEGF protein in the HDFC culture supernatants was 35.1±4.2 ng/L.
As detected by RT-PCR, the VEGF gene was expressed in the cultured HDFC (Figure 2).
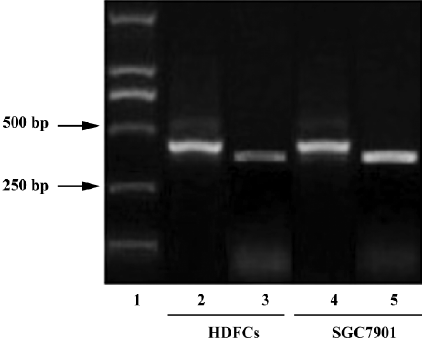
Effect of VEGF on HDFC proliferation The growth-stimulative effect of VEGF on cultured HDFC was examined by MTT assay. In the concentration-dependent pattern, compared with the control group (VEGF at 0 µg/L), VEGF at 1 µg/L or 400 µg/L induced a small increase in HDFC proliferation, but the difference did not reach statistical significance (P=0.233 and 0.087, respectively). At 10–300 µg/L, VEGF significantly increased HDFC proliferation (P<0.01, Figure 3A).
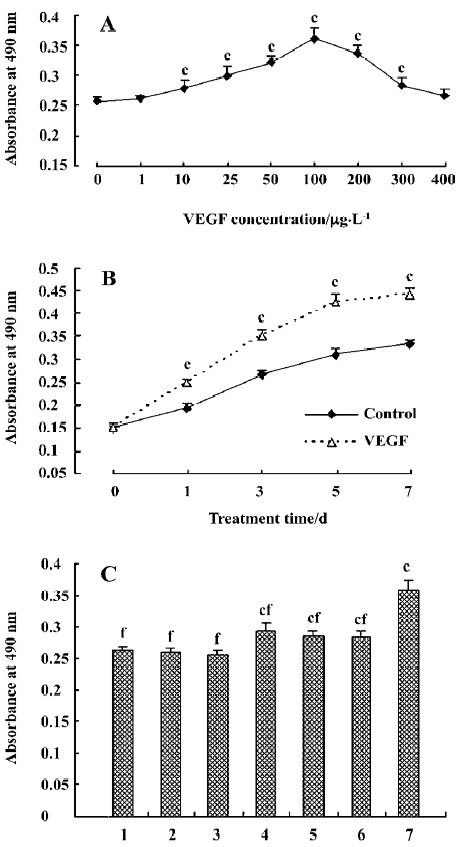
In the time-course pattern, following 1, 3, 5, or 7 d stimulation, VEGF induced a significant increase in HDFC proliferation compared with the corresponding control (P<0.01, Figure 3B).
Before evaluating the effect of the MAPK inhibitors on the VEGF-mediated HDFC proliferation, we first investigated the toxic effect of the 2 inhibitors on HDFC proliferation. Compared with the control group, PD98059 or U0126 could slightly inhibit HDFC proliferation, but the difference did not reach statistical significance (P=0.227 and 0.083, respectively).
The effect of the MAPK inhibitors on the VEGF-mediated HDFC proliferation was then evaluated. PD98059 and/or U0126 could significantly inhibit the VEGF-mediated HDFC proliferation when compared to the treatment group with VEGF alone (P<0.01). However, the proliferative levels of HDFC of the groups with the inhibitors plus 100 µg/L VEGF were still higher than those of the control group (P<0.01, Figure 3C).
Effect of VEGF on ALP activity in cultured HDFC The effect of VEGF on ALP activity in cultured HDFC was determined by colorimetric ALP assay kit. In a concentration-dependent pattern, compared with the control group (VEGF at 0 µg/L), VEGF at a concentration of 10–300 µg/L significantly increased ALP activity (P<0.01, Figure 4A).
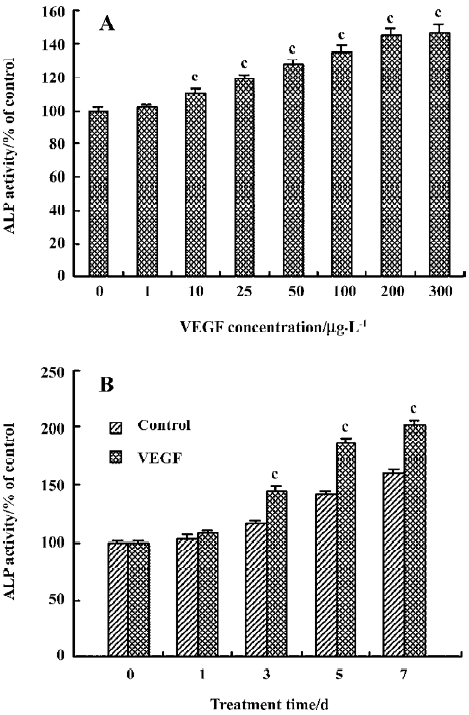
In a time-dependent pattern, following 3, 5, or 7 d stimula-tion, VEGF induced a significant increase in ALP activity when compared to the corresponding control (P<0.01, Figure 4B).
Effect of VEGF on cell apoptosis The results from the Annexin V/PI staining assay showed that fewer apoptotic cells were observed in the experimental groups after an incubation period of 3 d (Figure 5), but there was no significant difference in the apoptosis rate between the experimental groups and the control groups (P=0.057).
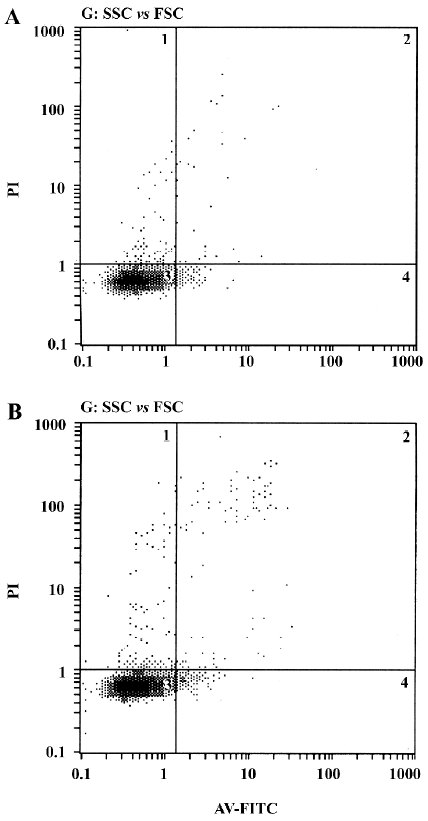
Discussion
Tooth eruption depends on the local changes in bone metabolism and these changes in turn depend upon the dental follicle[30]. The dental follicle cells, as the basic structure and functional unit of the dental follicle, have been the aim of intensive research for fully understanding the precise role of the dental follicles. It is not clear whether the conclusions obtained from rodentine dental follicle are applicable to the human dental follicle. Recently, Morsczeck et al reported the culturing and identification of dental follicle cells obtained from human wisdom teeth[24]. Their substantial report provides excellent support for HDFC culture.
In the present study, the RT-PCR result demonstrated that the VEGF gene was transcribed in cultured HDFC. Moreover, as shown by immunostaining, the VEGF gene was translated. The VEGF protein was also detected in the HDFC culture supernatant by ELISA. Thus, the dental follicle served as an endogenous source for the VEGF protein. Considering its function in bone remodeling, the presence of VEGF in cultured HDFC strongly suggests that VEGF played a role in tooth eruption.
Among the numerous studies on the dental follicle, only a few have investigated whether the proliferation of HDFC is under the regulation of endogenous factors that are secreted by HDFC or that of the surrounding cells. The incubation of cultured rat dental follicle cells with the epidermal growth factor (EGF) over 6 d increased their growth 2-fold compared to the controls[31], while the platelet-derived growth factor (PDGF) and basic fibroblast growth factor seemed to stimulate mouse dental follicle cell DNA synthesis[32]. Since VEGF (a member of the PDGF family) was found to be expressed in cultured HDFC (in the first part of the study), the present study also explored the roles of VEGF in the proliferation, differentiation, and apoptosis of cultured HDFC. In our observation, VEGF at 10–100 µg/L could obviously promote HDFC proliferation in a concentration-dependent manner. At higher concentrations over 100 µg/L, the growth-stimulative effect of VEGF on cultured HDFC diminished proportionally to the increase in concentration. Thus, it seemed that 100 µg/L was the optimal concentration to stimulate HDFC proliferation. Moreover, VEGF at 100 µg/L could stimulate HDFC proliferation in a time-dependent manner. This suggests that VEGF at a proper concentration range might act as a mitogen for HDFC, just as it does for human dental pulp cells[33]. The results also indicate that HDFC has a sensitive response to the change of VEGF concentration.
Annexin V/PI reagents were widely used to measure apoptosis. Fluorescence marked Annexin V can bind to the phosphatidyl serine in the outer membrane of apoptotic cells, and thus the cells can be detected through a flow cytometer[34]. The results from the Annexin V/PI staining assay showed that VEGF could protect the HDFC from apoptosis. Coupled with the results of the MTT assay, it seemed that the effect of VEGF on the HDFC viability was mainly through promoting HDFC proliferation, but not inhibiting HDFC apoptosis.
MAPK are widespread and important signal transduction enzymes in eukaryotes. ERK, a key molecule in the MAPK pathway, is involved in several cellular processes such as proliferation, cell survival, differentiation, and cytokine-activated migration[35]. As demonstrated in other cell types, VEGF produced a time- and dose-dependent activation of ERK1/2. The specific ERK1/2 inhibitor PD98059 could abolish ERK1/2 activation and endothelial cell proliferation in a dose-dependent manner[36]. The present study showed that PD98059 attenuated the VEGF-mediated HDFC proli-feration. To confirm that the inhibitory effect of PD98059 was not due to its toxic effect, we investigated the toxic effect of PD98059 on HDFC proliferation. The data indicated that PD98059 could slightly inhibit HDFC proliferation compared with the control group, but the difference was not statistically significant. It suggested that the inhibitory effect of PD98059 on the VEGF-mediated HDFC proliferation was induced by blocking the MAPK signaling pathway and not by its toxic effect. However, the data also showed that PD98059 failed to completely block the VEGF-mediated HDFC proliferation. To exclude the possibility that PD98059 tested was not strong enough to completely inhibit ERK1/2, we tested U0126, another MEK-ERK specific inhibitor. U0126 inhibits both active and inactive MEK1/2 (the upstream protein of ERK1/2), unlike PD98059 which only inhibits activation of inactive MEK1/2. However, U0126 or U0126 plus PD98059 did not completely block cell proliferation induced by VEGF either. We suggest 2 possible explanations for the pheno-menon. One is that the MAPK signaling pathway is not the sole mechanism responsible for VEGF-mediated HDFC proliferation; there are other signaling pathways involved. The other is that VEGF might enhance the expression of other growth factors or cytokines in cultured HDFC, such as EGF. These growth factors or cytokines further stimulate HDFC proliferation. Thus, it is an indirect effect of VEGF on HDFC proliferation. Regardless, a more detailed study is required to elucidate the precise mechanism of VEGF on HDFC proliferation.
Previous studies based on the rodentine dental follicle show that the dental follicle cells could exhibit partial characteristics of a mineralized tissue-forming phenotype[37]. Recently, HDFC have been reported to share some of the biochemical features of osteoblastic phenotypes. Moreover, dexamethasone and insulin could upregulate the expression of some typical osteoblast- or cementoblast-related genes such as osteocalcin and nestin in cultured HDFC. This could indicate that dexamethasone and insulin can promote differentiation of HDFC into cementoblasts and osteoblasts[38]. ALP is an indication of early osteoblast differentiation. It is one of the enzymes necessary for bone formation and its secretion indicates that bone formation is occurring and differentiation is beginning. However, the change of the ALP level and activity in cultured HDFC induced by cytokines has not been investigated. The present study suggests that ALP was expressed in cultured HDFC. Moreover, VEGF could enhance ALP activity in a dose- and time-dependent manner. This suggests that VEGF also has the ability to promote HDFC differentiation along a “cementoblast/osteoblast” pathway. However, since the dental follicle cells, which are progenitors for cementoblasts, PDL fibroblasts and alveolar osteoblasts, have multipotential differentiation ability, we do not know whether all of the follicle cells respond to VEGF treatment or only a selected population (the progenitors for cementoblasts and alveolar osteoblasts) respond. Thus, these results need further detailed investi-gation.
In summary, the present study demonstrates that both the VEGF gene and protein were expressed in cultured HDFC. The present study also provides evidence that VEGF at a proper concentration range could stimulate HDFC proliferation, promote HDFC to differentiate along a “cementoblast/osteoblast” pathway, and protect HDFC from apoptosis. The MAPK signaling pathway might be involved in the VEGF-mediated HDFC proliferation, but the precise signal regulation mechanism remains to be further investigated in the future.
Acknowledgements
Sincere thanks are expressed to Prof Yan JIN (Tissue Engineering Center, Qindu Stomatological College, Fourth Military Medical University, Xi’an, China) for technical assistance and Dr Ye-fei ZHU (Department of Veterinary and Biomedical Sciences, University of Nebraska-Lincoln, Lincoln, USA) for critical reading of the manuscript.
References
- Cahill DR, Marks SC Jr. Tooth eruption: evidence for the central role of the dental follicle. J Oral Pathol 1980;9:189-200.
- Marks SC Jr, Cahill DR. Experimental study in the dog of the non-active role of the tooth in the eruptive process. Arch Oral Biol 1984;29:311-22.
- Marks SC Jr, Cahill DR. Regional control by the dental follicle of alterations in alveolar bone metabolism during tooth eruption. J Oral Pathol 1987;16:164-9.
- Wise GE, Lin F, Zhao L. Transcription and translation of CSF-1 in the dental follicle. J Dent Res 1995;74:1551-7.
- Que BG, Wise GE. Colony-stimulating factor-1 and monocyte chemotactic protein-1 chemotaxis for monocytes in the rat dental follicle. Arch Oral Biol 1997;42:855-60.
- Wise GE, Yao S, Odgren PR, Pan F. CSF-1 Regulation of osteo-clastogenesis for tooth eruption. J Dent Res 2005;84:837-41.
- Ferrara N. Vascular endothelial growth factor: basic science and clinical progress. Endocr Rev 2004;25:581-611.
- Dvorak HF, Brown LF, Detmar M, Dvorak AM. Vascular permeability factor/vascular endothelial growth factor, microvascular hyperpermeability, and angiogenesis. Am J Pathol 1995;146:1029-39.
- Mandriota SJ, Pepper MS. Vascular endothelial growth factor-induced in vitro angiogenesis and plasminogen activator expression are dependent on endogenous basic fibroblast growth factor. J Cell Sci 1997;110:2293-302.
- Spyridopoulos I, Brogi E, Kearney M, Sullivan AB, Cetrulo C, Isner JM, et al. Vascular endothelial growth factor inhibits endothelial cell apoptosis induced by tumor necrosis factor-α: balance between growth and death signals. J Mol Cell Cardiol 1997;29:1321-30.
- Gerber HP, McMurtrey A, Kowalski J, Yan M, Keyt BA, Dixit V, . VEGF regulates endothelial cell survival by the PI3-kinase/Akt signal transduction pathway. Requirement for Flk-1/KDR activation. J Biol Chem 1998; 273: 30 336–43.
- Shibuya M. Differential roles of vascular endothelial growth factor receptor-1 and receptor-2 in angiogenesis. J Biochem Mol Biol 2006;39:469-78.
- Morabito A, De Maio E, Di Maio M, Normanno N, Perrone F. Tyrosine kinase inhibitors of vascular endothelial growth factor receptors in clinical trials: current status and future directions. Oncologist 2006;11:753-64.
- Perry BN, Arbiser JL. The duality of angiogenesis: implications for therapy of human disease. J Invest Dermatol 2006;126:2160-6.
- Kiselyov A, Balakin KV, Tkachenko SE. VEGF/VEGFR signalling as a target for inhibiting angiogenesis. Expert Opin Investig Drugs 2007;16:83-107.
- Niida S, Kaku M, Amano H, Yoshida H, Kataoka H, Nishikawa S, et al. Vascular endothelial growth factor can substitute for macrophage colony-stimulating factor in the support of osteoclastic bone resorption. J Exp Med 1999;190:293-8.
- Kaku M, Niida S, Kawata T, Maeda N, Tanne K. Dose- and time-dependent changes in osteoclast induction after a single injection of vascular endothelial growth factor in osteopetrotic mice. Biomed Res 2000;21:67-72.
- Henriksen K, Karsdal M, Delaisse JM, Engsig MT. RANKL and vascular endothelial growth factor (VEGF) induce osteoclast chemotaxis through an ERK1/2-dependent mechanism. J Biol Chem 2003; 278: 48 745–53.
- Yao S, Liu D, Pan F, Wise GE. Effect of vascular endothelial growth factor on RANK gene expression in osteoclast precursors and on osteoclastogenesis. Arch Oral Biol 2006;51:596-602.
- Street J, Bao M, deGuzman L, Bunting S, Peale FV Jr, Ferrara N, et al. Vascular endothelial growth factor stimulates bone repair by promoting angiogenesis and bone turnover. Proc Natl Acad Sci USA 2002;99:9656-61.
- Zelzer E, McLean W, Ng YS, Fukai N, Reginato AM, Lovejoy S, et al. Skeletal defects in VEGF(120/120) mice reveal multiple roles for VEGF in skeletogenesis. Development 2002;129:1893-904.
- Mayr-Wohlfart U, Waltenberger J, Hausser H, Kessler S, Gunther KP, Dehio C, et al. Vascular endothelial growth factor stimulates chemotactic migration of primary human osteoblasts. Bone 2002;30:472-7.
- Wise GE, Yao S. Expression of vascular endothelial growth factor in the dental follicle. Crit Rev Eukaryot Gene Expr 2003;13:173-80.
- Morsczeck C, Götz W, Schierholz J, Zeilhofer F, Kühn U, Möhl C, et al. Isolation of precursor cells (PCs) from human dental follicle of wisdom teeth. Matrix Biol 2005;24:155-65.
- Meng F, Ding J, Liu N, Zhang J, Shao X, Shen H, et al. Inhibition of gastric cancer angiogenesis by vector-based RNA interference for Raf-1. Cancer Biol Ther 2005;4:113-7.
- Zhang J, Ding J, Zhang X, Shao X, Hao Z. Regulation of vascular endothelial growth factor (VEGF) production and angiogenesis by tissue Factor (TF) in SGC-7901 gastric cancer cells. Cancer Biol Ther 2005;4:769-72.
- Cho ML, Jung YO, Moon YM, Min SY, Yoon CH, Lee SH, et al. Interleukin-18 induces the production of vascular endothelial growth factor (VEGF) in rheumatoid arthritis synovial fibroblasts via AP-1-dependent pathways. Immunol Lett 2006;103:159-66.
- Shigeyama J, Yasumura Y, Sakamoto A, Ishida Y, Fukutomi T, Itoh M, et al. Increased gene expression of collagen Types I and III is inhibited by β-receptor blockade in patients with dilated cardiomyopathy. Eur Heart J 2005;26:2698-705.
- Morais S, Sousa JP, Fernandes MH, Carvalho GS. In vitro biomineralization by osteoblast-like cells. I. Retardation of tissue mineralization by metal salts. Biomaterials 1998;19:13-21.
- Gorski JP, Marks SC Jr. Current concepts of the biology of tooth eruption. Crit Rev Oral Biol Med 1992;3:185-206.
- Lin F, Zhao L, Wise GE. In vivo and in vitro effects of epidermal growth factor on its receptor gene expression in rat dental follicle cells. Arch Oral Biol 1996;41:485-91.
- Bsoul S, Terezhalmy G, Abboud H, Woodruff K, Abboud SL. PDGF BB and bFGF stimulate DNA synthesis and upregulate CSF-1 and MCP-1 gene expression in dental follicle cells. Arch Oral Biol 2003;48:459-65.
- Matsushita K, Motani R, Sakuta T, Yamaguchi N, Koga T, Matsuo K, et al. The role of vascular endothelial growth factor in human dental pulp cells: induction of chemotaxis, proliferation, and differentiation and activation of the AP-1-dependent signaling pathway. J Dent Res 2000;79:1596-603.
- Xu J, Xin SQ, Li H, Liu L, Xia WY, Li PF, et al. Involvement of caspase-3 pathway in anti-apoptotic action of methionine enkephalin on CEM×174 cells in prolonged infection with simian immunodeficiency virus in vitro. Cell Biol Int 2006;30:114-21.
- Chang L, Karin M. Mammalian MAP kinase signaling cascades. Nature 2001;410:37-40.
- Parenti A, Morbidelli L, Cui XL, Douglas JG, Hood JD, Granger HJ, et al. Nitric oxide is an upstream signal of vascular endothelial growth factor-induced extracellular signal-regulated kinase1/2 activation in postcapillary endothelium. J Biol Chem 1998;273:4220-6.
- Hou LT, Liu CM, Chen YJ, Wong MY, Chen KC, Chen J, et al. Characterization of dental follicle cells in developing mouse molar. Arch Oral Biol 1999;44:759-70.
- Morsczeck C, Moehl C, Götz W, Heredia A, Schäffer TE, Eckstein N, et al. In vitro differentiation of human dental follicle cells with dexamethasone and insulin. Cell Biol Int 2005;29:567-75.