Discovery of a novel competitive inhibitor of PTP1B by high-throughput screening1
Introduction
Protein tyrosine phosphatase 1B (PTP1B) is a member of the family of protein tyrosine phosphatase (PTPase) and is further classified as a non-receptor PTPase for its location to the cytoplasmic face of the endoplasmic reticulum through the C-terminal 35 residues. Considerable progress has been made recently in the understanding of the relationship between PTP1B and type 2 diabetes. In vitro, PTP1B associates with tyrosine residues 1162 and 1163 of the insulin receptor (IR)[1,2]. Many other studies have shown that PTP1B can directly interact with the activated IR or IR substrate-1 (IRS-1) to dephosphorylate phosphotyrosine residues, resulting in the downregulation of insulin action[1–4]. Compelling data also came from PTP1B knockout mice, which displayed increased insulin sensitivity in a tissue-specific manner[5,6]. Enhanced tyrosine phosphorylation of the IR was observed in the muscle and liver, suggesting that the receptor might be a direct substrate of PTP1B[5].
The increased expression of PTP1B in the adipose tissue and muscle of obese humans and rodents is thought to be related to insulin resistance[7], whereas the increased insulin sensitivity from weight loss is accompanied by reduced PTP1B activity[8].
Further evidence for the involvement of PTP1B in insulin resistance was provided by cell line studies. PTP1B overexpression in rat primary adipose tissues and 3T3/L1 adipocytes has been shown to decrease insulin-sensitive Glut4 translocation[9] and IR and IRS-1 phosphorylation[10], respectively.
PTP1B has been widely recognized as an attractive target for therapy of type 2 diabetes. The development of PTP1B inhibitors has become a promising way to treat type 2 diabetes, even though it has been demonstrated that it is not easy to find a selective, safe, and effective PTP1B inhibitor[11].
We developed a molecular level, high-throughput screening assay for PTP1B inhibitors. After the screening of our compound library, we identified LGH00081 as a novel inhibitor of PTP1B.
Materials and methods
Materials and instruments The plasmid pGEX-KG was a kind gift from Dr Kun-liang GUAN of the University of Michigan (Ann Arbor, MI, USA). The restriction enzymes and Ex Taq polymerase were from TaKaRa (Dalian, China). Escherichia coli strain BL21-CodonPlus (DE3) was purchased from Stratagene (La Jolla, CA, USA). GSTrap FF was obtained from Amersham Pharmacia Biotech (Uppsala, Sweden). Substrate p-nitrophenyl phosphate (pNPP) was from Calbiochem (San Diego, CA, USA) and 3-O-methylfluorescein phosphate (OMFP) was from Sigma Aldrich (St Louis, MO, USA). Other reagents and solvents used in experiments were of analytical grade. Ham’s F12 medium (F12) was from Invitrogen (Carlsbad, CA, USA). Fetal bovine serum (FBS) was purchased from Hyclone (Logan, UT, USA). pY20 and anti-IR (IRβ) were from Santa Cruz Biotechnology (Santa Cruz, CA, USA). Anti-β-actin, horseradish peroxidase(HRP)-linked antimouse immunoglobulin (IgG) and antirabbit IgG antibodies were from Cell Signal Technology (Danvers, MA, USA). The polyvinylidene fluoride (PVDF) membranes were from Millipore (Billerica, MA, USA) and the enhanced chemiluminescence (ECL) reagents were from Calbiochem (USA).
Construction, expression, and purification of the PTP1B catalytic domain The nucleotide fragment encoding the human PTP1B catalytic domain was amplified by RT-PCR using RNA from human placenta. The cDNA of the PTP1B catalytic domain (91–1053 according to gi190741) was cloned into the pGEX-KG expression vector with EcoR I/Sac I. The nucleotide sequence cloned into the recombinant plasmid was confirmed by DNA sequencing. The recombinant plasmid was then transformed into Escherichia coli BL21-CodonPlus (DE3) for expression. BL21-CodonPlus (DE3) cells containing the recombinant plasmid were grown in 1 L Luria-Bertani (LB) medium in the presence of ampicillin (100 mg/L) by shaking at 37 °C, and the protein expression was induced by adding isopropyl β-D-thiogalac-topyranoside (IPTG) to 500 nmol/L when the cell density reached optical density at 600 nm (OD600) of 0.4–0.6. The cells were harvested after 4 h by centrifugation for 2 min at 7000×g and washed twice with phosphate-buffered saline (PBS) buffer (140 mmol/L NaCl, 2.7 mmol/L KCl, 10 mmol/L Na2HPO4, and 1.8 mmol/L KH2PO4), and resuspended in 30 mL PBS including 0.1% Triton X-100, 1 mmol/L EDTA, and 2 mmol/L dithiothreitol (DTT). The cells were lysated by sonication for 3 min on ice. After centrifugation at 12 000×g for 15 min, the supernatant was loaded onto a GSTrap FF column equilibrated previously with PBS buffer with 1 mmol/L EDTA and 2 mmol/L DTT. The loaded column was washed with PBS buffer, and then the bound active protein was eluted with elution buffer (50 mmol/L Tris-HCl, pH 8.0, 10 mmol/L glutathione, 1 mmol/L EDTA, and 2 mmol/L DTT). The protein samples from the purification procedure were analyzed by 10% reducing SDS-PAGE gel and the protein concentration was determined by the Bradford method with bovine serum albumin (BSA).
PTP1B enzymatic assay The enzymatic activities of the PTP1B catalytic domain were determined at 30 °C by monitoring the hydrolysis of pNPP. Dephosphorylation of pNPP generates product pNP, which was monitored at an absorbance of 405 nm by the EnVision multilabel plate reader (PerkinElmer Life Sciences, Boston, MA, USA). In a typical 100 µL assay mixture containing 50 mmol/L 3-[N-morpholino] propane-sulfonic acid (MOPs), pH 6.5, 2 mmol/L pNPP, and 30 nmol/L recombinant PTP1B, activities were continuously monitored and the initial rate of the hydrolysis was determined using the early linear region of the enzymatic reaction kinetic curve.
PTP1B inhibitor screening High-throughput screening (HTS) optimization and validation were undertaken according to our standard operation process before the primary screening. A total of 48 000 pure chemicals collected from different sources with wide structural diversity were screened. Two microliters of stock solution of compounds in DMSO was transferred into the wells of a 96-well flat-bottom plate (Greiner, Frickenhausen, Germany); this yielded a final compound concentration of 2 µg/mL and 2% DMSO. After initializing the enzymatic reaction, the plate was then read every 20 s for 2 min in the EnVision multilabel plate reader at 405 nm. All screening operations were performed by SAGIAN core integrated robotic system (Beckman Coulter, Fullerton, CA, USA). We used Na3VO4 as the positive control and DMSO as the negative control to evaluate our HTS system. The slope of the linear portion of the kinetic curve generated from each well was used to determine the activity of PTP1B. Both the negative and positive controls were set in each plate for calculating. The inhibition effect of the compound was represented by the percentage of the slope of the linear portion of its kinetic curve relative to that of the negative control. Quality control parameters involving coefficient of variation (CV) and Z´ factor were calculated in real time[12].
For the 50% percentage inhibition concentration (IC50) caculation, inhibition assays were performed with 30 nmol/L recombinant enzyme, 2 mmol/L pNPP in 50 mmol/L MOPS at pH 6.5, and the inhibitors diluted around the estimated IC50 values. The IC50 was calculated with Prism 4 software (Graphpad, San Diego, CA, USA) from the non-linear curve fitting of the percentage of inhibition (% inhibition) versus the inhibitor concentration [I] by using the following equation: % Inhibition = 100/(1+[IC50/[I]]k), where k is the Hill coefficient.
Characterization of the PTP1B inhibitor identified from the screening To characterize the hit identified from the HTS, the assay was carried out in a 100 µL system containing 50 mmol/L MOPS, pH 6.5, 30 nmol/L PTP1B, pNPP in 2-fold dilution from 80 mmol/L, and different concentrations of the inhibitor. In the presence of the competitive inhibitor, the Michaelis-Menten equation is described as 1/v=(Km/[Vmax[S]])(1+[I]/Ki)+1/Vmax, where v is the initial rate, Vmax is the maximum rate, and [S] is the substrate concentration. The Ki value was obtained by the linear replot of apparent Km/Vmax (slope) from the primary reciprocal plot versus the inhibitor concentration [I] according to the equation Km/Vmax=1+[I]/Ki.
Molecular docking The docking was completed by the Discovery Studio program (Accelrys, San Diego, CA, USA). The crystal structure of PTP1B (Protein Data Bank code 2hb1) was defined as the receptor, and the active site of PTP1B was regarded as the binding site of our competitive inhibitor LGH00081. LGH00081 was docked in a flexible manner, and conformations are determined by the stochastic Monte Carlo (MC) conformation search method. The number of MC trials was fixed to 50 000.
Selectivity of LGH00081 on other PTPase family members We evaluated the inhibitory effects of LGH00081 on other PTPase family members, T-cell PTPase (TCPTP), Src homology domain 2 (SH2) –containing tyrosine phosphatase-1 (SHP1), SHP2, leukocyte antigen-related phosphatase D1 (LAR D1), protein tyrosine phosphatase a (PTPa) and vaccinia virus VH-1-related dual-specific protein phosphatase (VHR). TCPTP (41–1075 according to BC008244), SHP1 (244–570 according to BC002523), SHP2 (1116–2162 according to NM002834.3), LAR D1 (1275–1613 according to gi18860871), PTPα D1 (697–1707 according to gi20073056), and VHR (56–613 according to BC002682) cDNA were cloned into pGEX-KG. GST-PTPase were overexpressed as GST-fusion proteins in Escherichia coli BL21-CondenPlus (DE3) and purified by affinity chromatography. Assays were performed for the majority of PTPase using 2 mmol/L pNPP as the substrate around their Km value (TCPTP: 1.12 mmol/L, SHP1: 11.76 mmol/L, SHP2: 7.82 mmol/L, LAR D1: 0.87 mmol/L, and PTPα D1: 1.25 mmol/L) and for VHR, which is insensitive to pNPP, using 2.5 µmol/L OMFP as the substrate with a Km value of 20 µmol/L at their optimal pH, respectively.
Cell culture The Chinese hamster ovary (CHO) cell line transfected with an expression plasmid-encoding human IR (CHO/hIR) was a kind gift from Dr Michel TREMBLAY of McGill University (Montreal, Canada). The cells were grown in F12 medium supplemented with 10% (v/v) FBS, 2 mmol/L L-glutamine, 50 units/mL penicillin and 50 µg/mL streptomycin.
Western blotting The cells were rinsed twice with PBS, terminated immediately by liquid nitrogen, then lysed with 1× SDS loading buffer. The samples were electrophoresed on 10% SDS-polyacrylamide gels and transferred to PVDF membranes. The membranes were blocked for 1 h with 5% (w/v) BSA and incubated with the primary antibodies overnight at 4 ºC and the secondary antibodies for 1 h at room temperature. Antigen-antibody complexes were detected by the ECL kit.
Results
HTS and quality control To find novel, small molecular inhibitors, we developed a HTS with PTP1B. The screening assay included 30 nmol/L GST-fusion PTP1B protein and 2 mmol/L pNPP substrate in order to obtain a good signal-to-noise ratio. After optimization, the intraplate and interplate CV were both less than 10%, suggesting that the liquid handling and compound transfer procedure was precise, and the Z´ factor, a standard statistical measure of assay quality, reached 0.69. A total of 48 000 compounds were screened in automated format in 1 d. The assay performed well with an averaged Z´ factor of 0.63; fifty compounds with an inhibition rate higher than 50% at a final concentration of 2 µg/mL were identified.
Discovery of a novel inhibitor of PTP1B from HTS After the hit validation and dose-response curve determination, compound LGH00081 with a novel structure (Figure 1A, chemical name: 5-(3-((Z)-((Z)-2-(4-chlorophenylimio)-4-oxothiazolidin-5-ylidene) methyl)-2,5-dimethyl-1H-pyrrol-1-yl) isophthalic acid) was discovered to potently inhibit PTP1B with an IC50 of 1.6 μmol/L (Figure 1B).
Characterization of compound LGH00081 LGH00081 demonstrated a time-independent inhibition of PTP1B (Figure 2A). We further determined the inhibition modality of LGH00081 for PTP1B; it inhibited PTP1B with the characteristics typical of a competitive inhibitor, as indicated by increased Km values and constant kcat values when the inhibitor concentration was increased (Figure 2B). Meanwhile, the result of the Lineweaver-Burk plot confirmed LGH00081 as a competitive inhibitor of PTP1B for intersecting at the y-axis of a nest of lines with increased inhibitor concentration (Figure 2C). The Ki value was calculated as 309 nmol/L from Figure 2D.
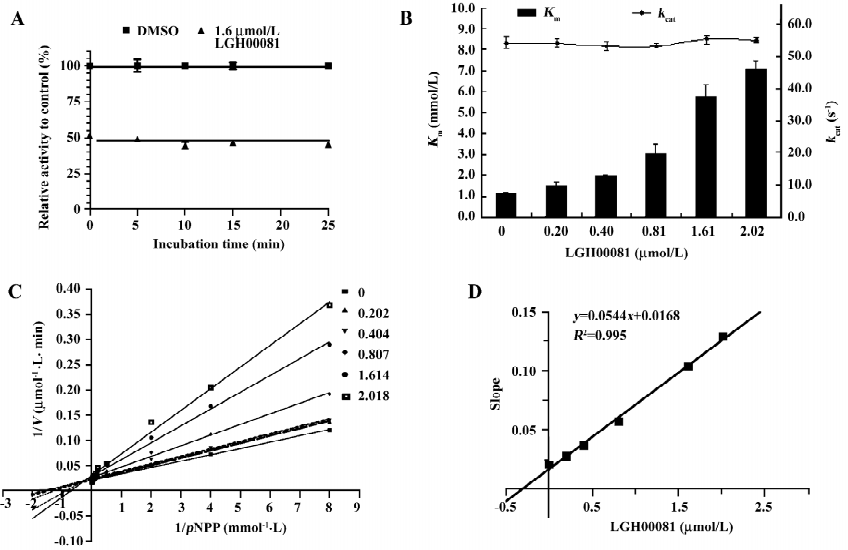
Interaction mode of LGH00081 and PTP1B by docking analysis As the docking result show (Figure 3), the compound could enter the catalytic pocket of PTP1B flexibly (Figure 3A) with the highest dock score of 64.724, and the predicted hydrogen bonds of LGH00081 were formed with Tyr46, Lys 120, and Gly220, respectively (Figure 3B).
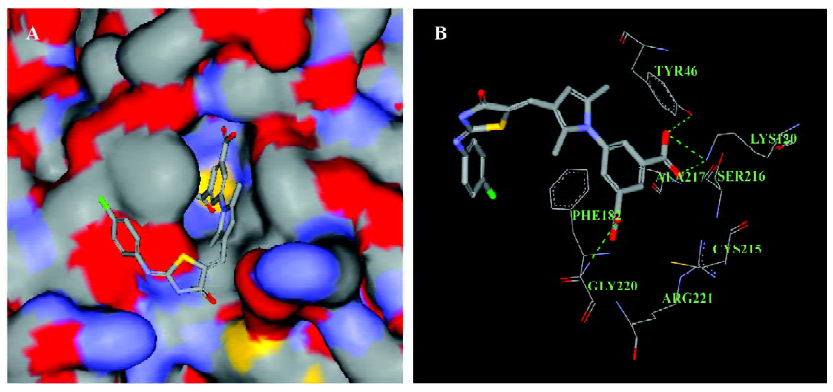
Inhibitory effect of LGH00081 on other PTPase family members To test the selectivity of the compound on other PTPase family members, we prepared human TCPTP, SHP1, SHP2, CD45D1D2, VHR, LAR D1, and PTPαD1 (Figure 4) and determined the IC50 values for these enzymes. The results are shown in Table 1. LGH00081 showed better inhibition on PTP1B, TCPTP, SHP1, and SHP2 than VHR, with no visible inhibitory effects to both receptor-type PTPase LAR and PTPα.
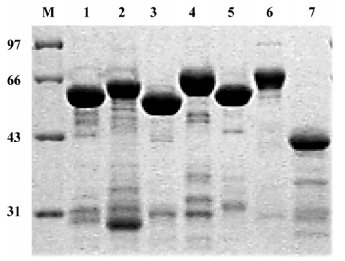
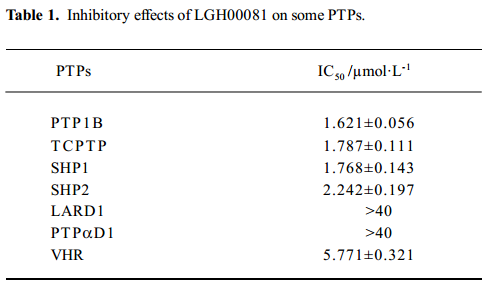
Full table
LGH00081 increased the IR tyrosine phosphorylation level in CHO/hIR cells To determine whether LGH00081 could exert its effect in the cell level to activate the insulin signaling pathway, we tested the enhanced effect of LGH00081 on IR phosphorylation in CHO/hIR cells by Western blotting. As shown in Figure 5, LGH00081 could synergistically increase the insulin–induced tyrosine phosphorylation level of IRβ in a dose-dependent manner and reached maximal effect at 10 µmol/L.
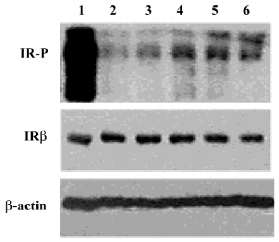
Discussion
Data from previous studies support the concept that PTP1B is a key negative regulator of insulin signal transduction. The termination of insulin signaling requires the dephosphorylation of IRβ, and its downstream effector is involved in insulin signal termination, thus the overactivation or overexpression of PTP1B can attenuate the insulin signal, resulting in insulin resistance[13,14]. Therefore reducing the activity of PTP1B, which dephosphorylates IRβ, would be expected to increase insulin sensitivity[15–18]. Consequently, compounds that inhibit PTP1B may have potential therapeutic use for the treatment of type 2 diabetes and obesity.
Since being developed approximately 20 years ago, HTS has become key technique used in drug discovery. HTS provides a fast, effective, and convenient tool to test hundreds of thousands of structural diverse compounds for their ability to modulate disease-relevant targets, and identifies novel compounds with interesting biological activities, which yields molecules that could be optimized into drugs. HTS assay for PTP1B was established to discover small-molecule inhibitors. The average Z´ factor is 0.63, which fulfills the demand for HTS quality control.
Through large-scale screening, several hits with good activity and novel structures were discovered. Here, compound LGH00081 was reported for the first time as a PTP1B inhibitor. LGH00081 shows an inhibition manner independent of incubation time, which may indicate that it is a fast-binding inhibitor to PTP1B. We demonstrated that it was a typical competitive inhibitor to PTP1B and the result was confirmed by the docking analysis in which LGH00081 was shown to enter into the catalytic pocket of PTP1B and behave as a competitor to the substrate.
Selectivity may be the biggest problem in the development of PTPase inhibitors. We measured the inhibitory effect of LGH00081 on different types of PTPase. We found that it inhibited non-receptor-type PTPase (such as TCPTP, SHP1, and SHP2 with an IC50 value around 2 µmol/L). For the dual-specific PTP VHR, LGH00081 showed similar inhibition, with an IC50 value of 5.77 µmol/L. No visible inhibitory effects on both receptor-type PTPase LAR and PTPα were found even at the highest concentration of 40 µmol/L in the test. Its effects on PTPase should be studied further to allow the modification on this scaffold to produce more potent and more selective inhibitors of individual PTPase.
The critical negative regulatory step in insulin signal transduction is the dephosphorylation of signaling molecules by PTPase, such as PTP1B, which helps to terminate insulin signaling. Changes in the expression levels or activities of specific PTPase have been reported to influence the insulin pathway and implicate insulin sensitivity, which is the most common inherent pathology of type 2 diabetes mellitus and obesity. IR phosphorylation elevation is a widely used experiment to validate PTP1B inhibitor bioactivity at the cellular level. Thus, the activity of LGH00081 was investigated in CHO/hIR cells. LGH00081 could elevate the tyrosine phosphorylation level of IRβ after stimulation by 10 nmol/L insulin.
In summary, using well-controlled HTS techniques, we discovered a PTP1B inhibitor with a novel structure, named LGH00081. We identified it as a competitive PTP1B inhibitor, and its interaction mode with PTP1B was predicted by docking analysis. Furthermore, it enhanced the tyrosine phosphorylation level of IR in CHO/hIR cells. The ongoing bioactivity-guided structure modification may lead to the discovery of more potent and selective PTPase inhibitors.
References
- Lee JH, Reed DR, Li WD, Xu W, Joo EJ, Kilker RL, et al. Genome scan for human obesity and linkage to markers in 20q13. Am J Hum Genet 1999;64:196-209.
- Di Paola R, Frittitta L, Miscio G, Bozzali M, Barratta R, Centra M, et al. A variation in 30 UTR of hPTP1B increases specific gene expression and associates with insulin resistance. Am J Hum Genet 2002;70:806-12.
- Mok A, Cao H, Zinman B, Hanley AJ, Harris SB, Kennedy BP, et al. A single nucleotide polymorphism in protein tyrosine phosphatase PTP1B is associated with protection from diabetes or impaired glucose tolerance in Oji-Cree. J Clin Endocrinol Metab 2002;87:724-7.
- Echwald SM, Bach H, Vestergaard H, Richelsen B, Kristensen K, Drivsholm T, et al. A P387L variant in protein tyrosine phosphatase-1B (PTP1B) is associated with type 2 diabetes and impaired serine phosphorylation of PTP1B in vitro. Diabetes 2002;51:1-6.
- Elchebly M, Payette P, Michaliszyn E, Cromlish W, Collins S, Loy AL, et al. Increased insulin sensitivity and obesity resistance in mice lacking the protein tyrosine phosphatase-1B gene. Science 1999;283:1544-8.
- Klaman LD, Boss O, Peroni OD, Kim JK, Martino JL, Zabolotny JM, et al. Increases energy expenditure, decreased adiposity, and tissue-specific insulin sensitivity in protein-tyrosine phosphatase 1B-deficient mice. Mol Cell Biol 2000;20:5479-89.
- Wu X, Hoffstedt J, Deeb W, Singh R, Sedkova N, Zilbering A, et al. Depot-specific variation in protein tyrosine phosphatases activities in human omental and subcutaneous adipose tissue: a potential contribution to differential insulin sensitivity. J Clin Endocrinol Metab 2001;86:5973-80.
- Ahmad F, Considine RV, Bauer TL, Ohannesian JP, Marco CC, Goldstein BJ, et al. Improved sensitivity to insulin in obese subjects following weight loss is accompanied by reduced protein tyrosine phosphatase in adipose tissue. Metabolism 1997;46:1140-5.
- Chen H, Cong LN, Li Y, Yao ZJ, Wu L, Zhang ZY, et al. A phosphotyrosyl mimetic peptide reverses impairment of insulin-stimulated translocation of GLUT4 caused by overexpression of PTP-1B in rat adipose cells. Biochemistry 1999;38:384-9.
- Venable CL, Frevert EU, Kim YB, Fisher BM, Kamatkar S, Nee BG, . Overexpression of protein tyrosine phosphatase 1B in adipocytes inhibits insulin-stimulated phosphoinositide 3-kinase activity without altering glucose transport or Akt/protein kinase B activation. J Biol Chem 2000; 275: 18 318–26.
- Ukkola O, Santaniemi M. Protein tyrosine phosphatase 1B: a new target for the treatment of obesity and associated co-morbidities. J Intern Med 2002;251:467-75.
- Zhang JH, Chung TD, Oldenburg KR. A simple statistical parameter for use in evaluation and validation of high throughput screening assays. J Biomol Screen 1999;4:67-73.
- Egawa K, Maegawa H, Kashiwagi A. Protein-tyrosine phosphatase-1B negatively regulates insulin signaling in L6 myocytes and Fao hepatoma cells. J Biol Chem 2001; 276: 10 207–11.
- Zabolotny JM, Haj FG, Kahn BB. Transgenic overexpression of PTP1B in muscle causes insulin resistance but overexpression with LAR does not additively impair insulin action. J Biol Chem 2004; 279: 24 844–51.
- Clampit JE, Meuth JL, Rondinone CM. Reduction of protein-tyrosine phosphatase-1B increase insulin signaling in FAO hepatoma cells. J Biol Chem 2003;300:261-7.
- Ahmad F, Li PM, Goldstein BJ. Osmotic loading of neutralizing antibodies demonstrates a role for protein-tyrosine phosphatase 1B in negative regulation of the insulin action pathway. J Biol Chem 1995; 170: 20 503–8.
- Issad T, Boute N, Boubekeur S, Lacasa D. Interaction of PTP1B with the insulin receptor precursor during its biosynthesis in the endoplasmic reticulum. Biochemie 2005;87:111-6.
- Xie LP, Lee SY, Zhang ZY. Cellular effects of small molecule PTP1B inhibitors on insulin signaling. Biochemistry 2003; 42: 12 792–804.