Cytotoxic effect of CdSe quantum dots on mouse embryonic development
Introduction
Quantum dots (QD) are colloidal nanocrystalline semiconductors that have unique light-emitting properties and can be used as a novel luminescent material. Typical QD are 1–12 nm in diameter and contain a small number of atoms in a discrete cluster[1]. QD absorb irradiated energy at wavelengths greater than their lowest energy transition, and then convert the irradiated energy to a narrow bandwidth emission. QD are ideal for development as luminescent probes due to the advantages of broadband excitation, narrow bandwidth emission, high intensity of emitted light, resistance to quenching, and photochemical stability. These properties make QD applicable for biochemical assays, in particular immunofluorescence staining. A previous study showed that CdSe-core QD induce cell death[2,3], but the regulatory mechanisms underlying this effect have not been elucidated.
Apoptosis, which is widely observed in different cells of various organisms, is the process of programmed cell death involving several morphological patterns, including chromatin condensation, membrane blebbing, and DNA fragmentation[4]. Apoptosis plays an important role in embryogenesis and homeostasis of multicellular organisms. The impairment of apoptotic function has been associated with several human diseases, including neurodegenerative disorders and cancer[5]. Although apoptosis contributes to normal embryonic development[6–8], teratogens can induce abnormal apoptosis during early embryogenesis, leading to disruption of the developmental process[9–13]. We recently reported the capacity of several apoptotic inducers to trigger apoptosis in embryonic stem cells and/or embryos of ICR Swiss Webster mice[10,11,14–16]. The effects of CdSe-core QD on human embryonic development are unclear. Our findings provide important new insights regarding the use of CdSe-core QD as biological tracers in mouse embryos.
To examine the effect of CdSe-core QD on pre-implantation development, we exposed mouse blastocysts to CdSe-core QD and examined apoptosis and cell proliferation in the inner cell mass (ICM) and trophectoderm (TE). We also investigated the effect of CdSe-core QD on the development of morulas into blastocysts. The effect of CdSe-core QD on post-implantation development was investigated by means of in vivo analyses of transferred embryos.
Materials and methods
Chemicals and reagents Pregnant mare’s serum gonadotropin (PMSG) and sodium pyruvate were purchased from Sigma (St Louis, MO, USA). Human chorionic gonadotropin (hCG) was obtained from Serono (NV Organon Oss, the Netherlands). The Annexin V-FLUOS staining kit and TUNEL in situ cell death detection kit were obtained from Roche (Mannheim, Germany) and the CMRL-1066 medium was from Gibco Life Technologies (Grand Island, NY, USA).
QD preparation Nanocrystals comprising a CdSe core and a ZnS shell were synthesized by Prof Chuan-hsin LU and coworkers at the Department of Chemical Engineering, National Taiwan University. Briefly, appropriate amounts of trioctylphosphine oxide (TOPO), cadmium oxide, and tetradecylphosphonic acid were heated to 180 °C under zargon, and dried and degassed under a vacuum. The reaction temperature was then increased to 330 °C; selenium (Se) precursor solution in trioctylphosphine (TOP) was injected into the reaction flask, and the mixture was allowed to cool to 240 °C. Zn and S stock solutions prepared with bis(trimethylsilyl)sulfide in TOP, along with a dimethyl zinc solution, were added drop-wise with vigorous stirring until a final mole ratio of 1:4 (Cd/Se:Zn/S) was achieved in the reaction. The reaction mixture was cooled to room temperature, and the nanocrystals were precipitated with anhydrous methanol, collected by centrifugation, and washed 3 times with anhydrous methanol for the removal of residual TOPO and the unreacted reagents. The precipitate was dissolved in anhydrous chloroform or tetrahydrofuran for the experiments. For water solubilization, the CdSe QD were surface coupled with mercaptoacetic acid and then suspended in phosphate-buffered saline (PBS) (the modification was performed by Prof Ruoh-chyu RUANN and coworkers at the Department of Chemical and Materials Engineering, National Central University, Taiwan). A particle sizer was used to measure the CdSe QD, which were found to be approximately 3.5 nm in diameter.
Animals and collection of embryos ICR mice were purchased from the National Laboratory Animal Center (Taiwan). This research was also approved by the Animal Research Ethics Board of Chung Yuan Christian University (Taiwan). ICR virgin albino mice (6–8 weeks old) were induced to superovulate by an injection of 5 IU PMSG followed by an injection of 5 IU hCG 48 h later. The mice were then mated overnight with a single fertile male of the same strain. The female mice with vaginal plugs were separated and used for the experiments. All of the mice were maintained on breeder chow and kept under a 12-h day/12-h night regimen with food and water available ad libitum. All of the animals received humane animal care, as outlined in the Guidelines for Care and Use of Experimental Animals (Canadian Council on Animal Care, Ottawa, Canada, 1984). The day a vaginal plug was found was defined as d 0 of pregnancy. Morulas were obtained by flushing the fallopian tubes on the afternoon of d 3, and blastocysts were obtained by flushing the uterine horn on d 4; in both cases, the flushing solution consisted of CMRL-1066 culture medium containing 1 mmol/L glutamine and 1 mmol/L sodium pyruvate.
QD treatment The embryos were collected in uncoated plastic 35 mm culture dishes and washed at least 3 times. Expanded blastocysts from different females were pooled and randomly selected for the experiments. The blastocysts were incubated at 37 °C for 24 h in CMRL-1066 medium with and without 0–500 nmol/L QD, and were then used for further experiments as described later.
Blastocyst cell counting To investigate the effect of CdSe-core QD on cell proliferation in embryos, we treated the mouse blastocysts (180 blastocysts in each group) with or without CdSe-core QD and then analyzed proliferation using differential staining. The blastocysts were incubated with culture medium containing 0–500 nmol/L QD for 24 h, washed with QD-free medium, and then dual differential staining was used to facilitate counting of cell numbers in the ICM and TE[17]. The blastocysts were incubated in 0.4% pronase in M2 medium containing 0.1% bovine serum albumin (M2-BSA medium) for the removal of the zona pellucida. The denuded blastocysts were exposed to 1 mmol/L trinitro-benzenesulphonic acid in BSA-free M2 medium containing 0.1% polyvinylpyrrolidone at 4 °C for 30 min, and then washed with M2 medium[18]. The blastocysts were further treated with 30 µg/mL antidinitrophenol-BSA complex antibody in M2-BSA at 37 °C for 30 min, and then with M2 medium supplemented with 10% whole guinea pig serum as a source of complement, 20 µg/mL bisbenzimide, and 10 µg/mL propidium iodide (PI) at 37 °C for 30 min. The immunolysed blastocysts were gently transferred to slides and protected from light before observation. Under UV light excitation, the ICM cells (which take up bisbenzimidine, but exclude PI) appeared blue, whereas the TE cells (which take up both fluorochromes) appeared orange-red. As multinucleated cells are not common in pre-implantation embryos[19], the number of nuclei was considered to represent an accurate measure of the cell number.
Detection of cell apoptosis To study the apoptotic effects of CdSe-core QD on embryos, we treated mouse blastocysts (200 blastocysts in each group) with 0–500 nmol/L CdSe-core QD at 37 °C for 24 h, and then measured cell apoptosis. For terminal deoxynucleotidyl transferase-mediated digoxigenin-dUTP nick-end labeling (TUNEL) staining, the embryos were washed in QD-free medium, fixed, permeabilized, and subjected to TUNEL labeling using an in situ cell death detection kit (Roche Molecular Biochemicals, Mannheim, Germany) according to the manufacturer’s protocol. Photographic images were taken using a fluorescence microscope under bright-field illumination (Olympus BX 51, Tokyo, Japan). For Annexin V staining, the blastocysts were incubated in 0–500 nmol/L QD for 24 h, washed with QD-free culture medium, and then stained with an Annexin V-FLUOS staining kit (Roche, Germany), according to the manufacturer’s instructions. Briefly, the blastocysts were incubated in M2-BSA for the removal of the zona pellucida, washed well with PBS plus 0.3% BSA, and then incubated for 60 min with a mixture of 100 µL binding buffer, 1 µL fluorescein isothiocyanate-conjugated Annexin V, and 1 µL PI. After incubation, the embryos were washed and photographed using a fluorescence microscope under fluorescent illumination. The cells staining Annexin V+/PI– were considered apoptotic, while those staining Annexin V+/PI+ were considered necrotic.
Observation of in vitro implantation and post-implantation development The blastocysts were cultured according to a modification of the previously reported method[11]. Briefly, the embryos were cultured in 4-well multidishes at 37 °C. For group culture, 3 embryos were cultured per well. The basic medium consisted of CMRL-1066 supplemented with 1 mmol/L glutamine and 1 mmol/L sodium pyruvate plus 50 IU/mL penicillin and 50 mg/mL streptomycin (hereinafter called culture medium). For the treatments, the embryos were cultured with the indicated doses of QD for 24 h without serum supplementation. Thereafter, the embryos were cultured for 3 d in culture medium supplemented with 20% fetal calf serum, and for 4 d in culture medium supplemented with 20% heated-inactivated human placental cord serum for a total culture time of 8 d from the onset of treatment. The embryos were inspected daily under a phase contrast microscope (Olympus IMT-2, Tokyo, Japan), and the developmental stages were classified according to established methods[20]. Developmental parameters, such as hatching through the zona pellucida, attachment to the culture dish, trophoblastic outgrowth, and differentiation of the embryo proper were recorded daily. To decrease observer bias, all data were analyzed using the following previously published criteria[9]: an implanted blastocyst was defined by attachment to the culture dish, followed by outgrowth; an early egg cylinder (EEC) was defined as an embryo that had reached stage 9 or 10 by d 4; a late egg cylinder (LEC) was defined as an embryo that had reached stage 11, 12, or 13 by d 6 of culture; and an early somite (ESS) was defined as an embryo that had reached stage 14 or 15 by d 8[9].
Blastocyst development following the embryo transfer To examine the ability of expanded blastocysts to implant and develop in vivo, the generated embryos were transferred to recipient mice. ICR females (white skin color) were mated with vasectomized males (C57BL/6J; black skin color; from the National Laboratory Animal Center, China) to produce pseudopregnant dams as recipients for the embryo transfer. To ensure that all fetuses in the pseudopregnant mice came from the embryo transfer (white color) and not from fertilization by C57BL/6J (black color), we examined the skin color of the fetuses at d 18 post-coitus. To assess the impact of QD on post-implantation growth in vivo, the blastocysts were exposed to 0 and 500 nmol/L QD for 24 h, and then 8 embryos were transferred in parallel to the paired uterine horns of d 4 pseudopregnant mice. The surrogate mice were killed on d 18 post-coitus, and the frequency of implantation was calculated as the number of implantation sites per number of embryos transferred. The incidence rates of resorbed and surviving fetuses were calculated as the number of resorp-tions or surviving fetuses, respectively, per number of implantations. The weights of the surviving fetuses and placentae were measured immediately after dissection.
Statistics The data were analyzed using one-way ANOVA and t-tests, and are presented as the mean±SD. A P-value <0.05 was considered significant.
Results
Effect of CdSe-core QD on cell apoptosis in mouse blastocysts TUNEL staining revealed that 250 and 500 nmol/L CdSe-core QD induced apoptosis in mouse blastocysts in a dose-dependent manner (Figure 1A). Quantitative analyses revealed that 250 and 500 nmol/L CdSe-core QD increased the number of apoptotic cells in blastocysts 4.2- and 6.6-fold, respectively, above the controls (Figure 1B). Annexin V and PI staining revealed a higher ratio of Annexin V+/PI– cells in the ICM of blastocysts exposed to QD than in the ICM of the controls. The ratio of Annexin V+/PI– cells was similar in the TE and controls, suggesting that the TE is unaffected by exposure to QD (Figure 1C). These results show that CdSe-core QD potently induce apoptosis in the ICM of mouse blastocysts.
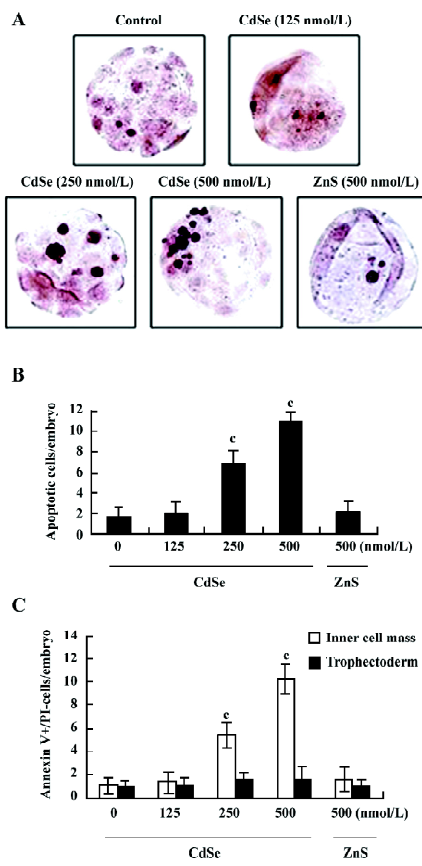
Effect of CdSe-core QD on blastocyst cell number The effect of CdSe-core QD on cell proliferation was investigated by differential staining. The results showed that blastocysts exposed to 250 or 500 nmol/L of CdSe-core QD contained fewer cells than the control blastocysts, an effect that was most pronounced in the ICM (Figure 2).
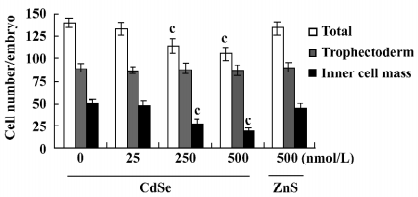
Effect of CdSe-core QD on implantation and post-implantation development The blastocysts treated with 125, 250, or 500 nmol/L CdSe-core QD (240, 200, and 250 blastocysts, respectively) showed reduced formation of the 2-layer ICM and ectoplacental cones. Also, fewer embryos developed to the advanced egg cylinder stages (LEC and ESS stages) compared to the controls (Figure 3A). When morulas were exposed to 500, 250, or 125 nmol/L CdSe-core QD, 41%, 55%, and 78.7% developed into blastocysts, respectively. By comparison, 85% of control morulas developed into blastocysts (Figure 3B).
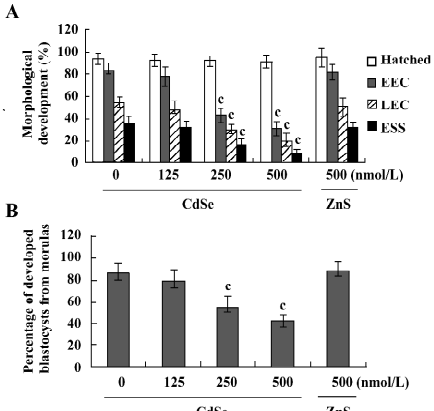
Determination of blastocyst developmental potential by embryo transfer The effect of CdSe-core QD on post-implantation development was investigated by transferring mouse blastocysts exposed to CdSe-core QD into a host mother and examining the embryos 13 d post-transfer (d 18 post-coitus). The implantation ratios for the CdSe-core QD-pretreated blastocysts and controls were ~65% (130 of 200 embryos in 25 recipients) and ~70% (140 of 200 embryos in 25 recipients), respectively (Figure 4A). The proportion of implanted embryos that failed to develop normally was significantly higher for the CdSe-core QD-pretreated blastocysts (105 of 130 implanted embryos; 80.8%) compared to the controls (50 of 140 implanted embryos; 35.7%). The embryos that implanted but fail to develop subsequently resorbed. The embryos pretreated with CdSe-core QD had a higher resorption rate and a lower surviving fetus rate than the controls (Figure 4A). There was no difference in placental weight between the CdSe-core QD-pretreated and the control groups (Figure 4B). By comparison, the fetal weight was lower in the CdSe-core QD-pretreated group versus the controls (477±61 mg vs 583±58 mg). Fetal weight is an important indicator of successful embryonic and fetal development. Of the fetuses in the CdSe-core QD-pretreated group, only 13% weighed over 600 mg. By comparison, 35% of the control fetuses exceeded this threshold (Figure 4C). Collectively, these results show that exposure to CdSe-core QD at the blastocyst stage reduces post-implantation development potential.
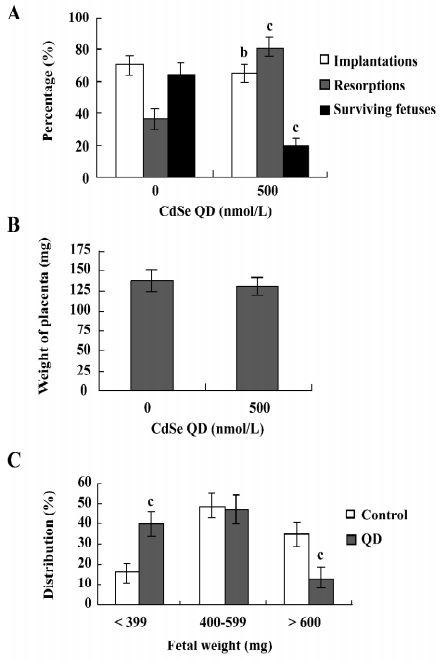
ZnS coating decreases the cytotoxic effect of CdSe QD on blastocysts The capacity of a ZnS coating on CdSe QD to reduce the cytotoxicity of CdSe QD on embryos was investigated. TUNEL assays and a proliferation analysis revealed that ZnS-coated CdSe QD had no significant cytotoxic effect on blastocysts (Figures 1,2). Furthermore, ZnS-coated CdSe QD did not inhibit post-implantation development (Figure 3). Collectively, these findings show that CdSe QD coated with ZnS have no significant cytotoxic effect on embryonic development.
Discussion
The induction of cell death by CdSe-core QD under certain conditions correlates with the release of free Cd2+ from the CdSe lattice. This effect is significantly reduced by adding a ZnS coating to the CdSe-core QD[2]. Photoluminescent semiconductor QD are novel nanometer-size probes for the bioimaging of immunostained cells[21], and fluorescent QD probes are useful bioimaging tools for tracing target cells over time in mouse models[22]. Fluorescent QD probes have the potential for development as biotracers for human disease diagnosis. For this reason, it is essential to fully investigate the cytotoxic capacity of QD. In the present study we show that CdSe-core QD-induced apoptosis and the inhibition of embryonic development is effectively reduced by a ZnS coating (Figures 1,2,3). Our results suggest that QD are likely to have latent cytotoxicity in the event of disruption of the ZnS coatings in vivo.
Cadmium (Cd) is a significant environmental and occupational toxic metal. Cd can have pro- or anti-apoptotic effects, depending on the cell type, dosage, and exposure period. Cd induces apoptosis in T lymphocytes[23], pig proximal tubule epithelial cells (LLC-PK1 cells)[24], canine proximal tubules[25], and rat testicular tissue[26]. The induction of apoptosis by Cd occurs by caspase-dependent[27] and -independent[28] pathways. Cd2+ also blocks apoptosis induced by a variety of agents[29]. Recent studies show that Cd2+ activates Ca2+/calmodulin-dependent protein kinase II in mouse mesangial cells and triggers both apoptotic and necrotic cell death[30]. Cd can also induce reactive oxygen species (ROS) generation (ie oxidative stress) and trigger apoptosis via a caspase-dependent pathway[31]. However, the mechanisms underlying Cd-induced apoptosis, cell cytotoxicity, and carcinogenesis remain unclear.
Se is an important dietary essential trace element for human life. There are at least 8 Se-containing proteins, including glutathione peroxidase and thioredoxin reductase. Dietary Se supplementation can protect cells from oxidative injury[32] and inhibit apoptosis[33,34]. Conversely, Se can also trigger cell apoptosis[34,35]. Further investigation is required to unravel the mechanisms underlying the protective and apoptotic effect of Se.
Embryonic development is a complex process during which chemical injury can lead to abortion or embryonic malformation. Thus, it is important to examine the possible teratogenic effects of commonly used nanoparticle fluorescence biotracers. The present study shows for the first time that CdSe-core QD decrease the viability of mammalian blastocysts by inducing apoptosis (Figure 1). Our results reveal that apoptosis in mouse blastocysts exposed to 250 and 500 nmol/L CdSe-core QD increases 4.2- and 6.6-fold, respectively, above the controls in a dose-dependent manner. CdSe-core QD at 125 nmol/L had no cytotoxic effect, as assessed by TUNEL staining (Figure 1A,1B). Annexin V/PI staining revealed significant CdSe-core QD-induced apoptosis in the ICM, but not in the TE (Figure 1C). While the blastocysts exposed to CdSe-core QD maintained the capacity to implant in vitro, post-implantation development was retarded, leading to embryonic death.
During embryonic development, cells are often poised between proliferation and apoptosis. While our previous studies showed that CdSe-core QD induced apoptosis, no studies have examined the apoptotic effects of CdSe-core QD during embryonic development. In the present study, we show that the exposure of mouse blastocysts to CdSe-core QD decreases cell number, induces apoptosis, delays pre-implantation development in vitro, and inhibits post-implantation development, perhaps due to a teratogenic effect.
The TE arises from the trophoblast at the blastocyst stage and subsequently develops into a sphere of epithelial cells surrounding the ICM and the blastocoel; these cells contribute to the placenta and are required for the development of the mammalian conceptus[36]. CdSe-core QD did not reduce cell numbers or induce apoptosis in the TE, and implantation in vitro was unaffected (Figures 1,2,3). Further work will be required to assess the effect of CdSe-core QD on differentiation and giant-cell formation in vitro and in vivo.
Mitochondria acts as important signaling conduits during programmed cell death, and loss of mitochondrial integrity can be promoted or inhibited by many key regulators of apoptosis[37,38]. Our previous study showed that CdSe-core QD induced the loss of mitochondrial membrane potential and the mitochondrial release of cytochrome c in IMR-32 cells in a dose-dependent manner[3]. That study also revealed a key role for ROS in CdSe-core QD-induced apoptosis in mitochondria, and that c-Jun N-terminal kinase (JNK) is an upstream regulator of the mitochondria-dependent apoptotic pathway[3]. These results indicate that the mechanism underlying CdSe-core QD-induced apoptosis involves ROS generation, JNK activation, and mitochondrial-dependent processes in IMR-32 cells. Our previous study also showed that CdSe-core QD-induced apoptosis was associated with reduced protein levels of heat shock protein 90 (HSP90) and the downstream targets, Ras, Raf-1, extracellular signal-regulated kinase-1 (ERK-1), and ERK-2 [3]. These findings suggest that a CdSe-core QD-induced decrease in HSP90 expression leads to an increase in the proteasome-dependent degradation of Ras and Raf-1, with the decrease in Raf-1 levels resulting in the subsequent downregulation of ERK-1 and ERK-2. Details of the regulatory mechanisms underlying CdSe-core QD-induced apoptosis in blastocysts are unclear and require further investigation.
Cd-induced cytotoxicity is associated with inflammation, fibrosis, organ dysfunction[39,40] and the development of various cancers[41]. Studies in Hep G2 cells implicate Cd in caspase-dependent apoptosis[31]. The cytotoxicity of CdSe QD correlates with the release of free Cd2+ from the CdSe lattice, which appears to be associated with surface oxidation[2]. The addition of a ZnS coating to CdSe QD significantly reduces any associated cytotoxicity[42]. For this reason, the capacity of a ZnS coating to reduce CdSe-core QD cytotoxicity during embryogenesis was investigated. Our study shows that a ZnS coating effectively reduces CdSe QD-induced cytotoxicity in blastocysts. We propose that the ZnS coating prevents CdSe-core QD-induced cell death and cytotoxicity by blocking surface oxidation and the subsequent release of Cd2+ ions. Further studies are required to comprehensively assess the mechanism by which a ZnS coating prevents CdSe-core QD-induced cell death and cytotoxicity.
In summary, the present study shows for the first time that CdSe-core QD decrease the viability of mammalian blastocysts by inducing apoptosis in the ICM. This is the first evidence that CdSe-core QD have a teratogenic effect via the induction of apoptosis. Further work is required to fully elucidate the mechanisms underlying CdSe-core QD-induced cell death and cytotoxicity and the relationship between these processes and teratogenicity.
Acknowledgments
We thank Dr Chuan-hsin LU (Department of Chemical Engineering, National Taiwan University, Taiwan) and Dr Ruoh-chyu RUAAN (Department of Chemical and Materials Engineering, National Central University, Taiwan) for providing the modified CdSe quantum dots.
References
- Murray CB, Kagan CR, Bawendi MG. Synthesis and characterization of monodisperse nanocrystals and close packed nanocrystal assemblies. Annu Rev Mater Sci 2000;30:545-610.
- Derfus AM, Chan WCW, Bhatia SN. Probing the cytotoxicity of semiconductor quantum dots. Nano Lett 2004;4:11-8.
- Chan WH, Shiao NH, Lu PZ. CdSe quantum dots induce apoptosis in human neuroblastoma cells via mitochondrial-dependent pathways and inhibition of survival signals. Toxicol Lett 2006;167:191-200.
- Ellis RE, Yuan JY, Horvitz HR. Mechanisms and functions of cell death. Annu Rev Cell Biol 1991;7:663-98.
- Thompson CB. Apoptosis in the pathogenesis and treatment of disease. Science 1995;267:1456-62.
- Brill A, Torchinsky A, Carp H, Toder V. The role of apoptosis in normal and abnormal embryonic development. J Assist Reprod Genet 1999;16:512-9.
- Lotz K, Proff P, Bienengraeber V, Fanghaenel J, Gedrange T, Weingaertner J. Apoptosis as a creative agent of embryonic development of bucca, mentum and nasolacrimal duct. An in vivo study in rats. J Craniomaxillofac Surg 2006;34 Suppl 2:8-13.
- Weingaertner J, Proff P, Bienengraeber V, Gedrange T, Fanghaenel J, Lotz K. In vivo study of apoptosis as a creative agent of embryonic development of the primary nasal duct in rats. J Craniomaxillofac Surg 2006;34 Suppl 2:3-7.
- Huang FJ, Shen CC, Chang SY, Wu TC, Hsuuw YD. Retinoic acid decreases the viability of mouse blastocysts in vitro. Hum Reprod 2003;18:130-6.
- Hsuuw YD, Chang CK, Chan WH, Yu JS. Curcumin prevents methylglyoxal-induced oxidative stress and apoptosis in mouse embryonic stem cells and blastocysts. J Cell Physiol 2005;205:379-86.
- Chan WH. Ginkgolide B induces apoptosis and developmental injury in mouse embryonic stem cells and blastocysts. Hum Reprod 2006;21:2985-95.
- Shang EH, Wu RS. Aquatic hypoxia is a teratogen and affects fish embryonic development. Environ Sci Technol 2004;38:4763-7.
- Detmar J, Rabaglino T, Taniuchi Y, Oh J, Acton BM, Benito A, et al. Embryonic loss due to exposure to polycyclic aromatic hydrocarbons is mediated by Bax. Apoptosis 2006;11:1413-25.
- Chan WH. Ginkgolides induce apoptosis and decrease cell numbers in mouse blastocysts. Biochem Biophys Res Commun 2005;338:1263-7.
- Chan WH. Citrinin induces apoptosis via a mitochondria-dependent pathway and inhibition of survival signals in embryonic stem cells, and causes developmental injury in blastocysts. Biochem J 2007;404:317-26.
- Chan WH, Shiao NH. Effect of citrinin on mouse embryonic development in vitro and in vivo. Reprod Toxicol 2007;24:120-5.
- Pampfer S, de Hertogh R, Vanderheyden I, Michiels B, Vercheval M. Decreased inner cell mass proportion in blastocysts from diabetic rats. Diabetes 1990;39:471-6.
- Hardy K, Handyside AH, Winston RM. The human blastocyst: cell number, death and allocation during late preimplantation development in vitro. Development 1989;107:597-604.
- Gardner RL, Davies TJ. Lack of coupling between onset of giant transformation and genome endoreduplication in the mural trophectoderm of the mouse blastocyst. J Exp Zool 1993;265:54-60.
- Witschi E. Characterization of developmental stages. Part II. Rat. In Biology Data Book, 2nd ed. Federation of American Societies of Experimental Biologies: Washington, DC; 1972. p 178–80.
- Goldman ER, Balighian ED, Mattoussi H, Kuno MK, Mauro JM, Tran PT, et al. Avidin: a natural bridge for quantum dot-antibody conjugates. J Am Chem Soc 2002;124:6378-82.
- Hoshino A, Hanaki K, Suzuki K, Yamamoto K. Applications of T-lymphoma labeled with fluorescent quantum dots to cell tracing markers in mouse body. Biochem Biophys Res Commun 2004;314:46-53.
- el Azzouzi B, Tsangaris GT, Pellegrini O, Manuel Y, Benveniste J, Thomas Y. Cadmium induces apoptosis in a human T cell line. Toxicology 1994;88:127-39.
- Matsuoka M, Call KM. Cadmium-induced expression of immediate early genes in LLC-PK1 cells. Kidney Int 1995;48:383-9.
- Hamada T, Tanimoto A, Iwai S, Fujiwara H, Sasaguri Y. Cytopathological changes induced by cadmium-exposure in canine proximal tubular cells: a cytochemical and ultrastructural study. Nephron 1994;68:104-11.
- Xu C, Johnson JE, Singh PK, Jones MM, Yan H, Carter CE. In vivo studies of cadmium-induced apoptosis in testicular tissue of the rat and its modulation by a chelating agent. Toxicology 1996;107:1-8.
- Kim MS, Kim BJ, Woo HN, Kim KW, Kim KB, Kim IK, et al. Cadmium induces caspase-mediated cell death: suppression by Bcl-2. Toxicology 2000;145:27-37.
- Shih CM, Wu JS, Ko WC, Wang LF, Wei YH, Liang HF, et al. Mitochondria-mediated caspase-independent apoptosis induced by cadmium in normal human lung cells. J Cell Biochem 2003;89:335-47.
- Yuan C, Kadiiska M, Achanzar WE, Mason RP, Waalkes MP. Possible role of caspase-3 inhibition in cadmium-induced blockage of apoptosis. Toxicol Appl Pharmacol 2000;164:321-9.
- Liu Y, Templeton DM. Cadmium activates CaMK-II and initiates CaMK-II-dependent apoptosis in mesangial cells. FEBS Lett 2007;581:1481-6.
- Oh SH, Lim SC. A rapid and transient ROS generation by cadmium triggers apoptosis via caspase-dependent pathway in HepG2 cells and this is inhibited through N-acetylcysteine-mediated catalase upregulation. Toxicol Appl Pharmacol 2006;212:212-23.
- Sun Y, Mu Y, Ma S, Gong P, Yan G, Liu J, . The molecular mechanism of protecting cells against oxidative stress by 2-selenium-bridged beta-cyclodextrin with glutathione peroxidase activity. Biochim Biophys Acta 2005; 1743: 199–204.
- Tang R, Liu H, Wang T, Huang K. Mechanisms of selenium inhibition of cell apoptosis induced by oxysterols in rat vascular smooth muscle cells. Arch Biochem Biophys 2005;441:16-24.
- Tang R, Huang K. Inhibiting effect of selenium on oxysterols-induced apoptosis of rat vascular smooth muscle cells. J Inorg Biochem 2004;98:1678-85.
- Hiraoka K, Komiya S, Hamada T, Zenmyo M, Inoue A. Osteosarcoma cell apoptosis induced by selenium. J Orthop Res 2001;19:809-14.
- Cross JC, Werb Z, Fisher SJ. Implantation and the placenta: key pieces of the development puzzle. Science 1994;266:1508-18.
- Green DR, Reed JC. Mitochondria and apoptosis. Science 1998;281:1309-12.
- Kroemer G, Zamzami N, Susin SA. Mitochondrial control of apoptosis. Immunol Today 1997;18:44-51.
- Falck FY Jr, Fine LJ, Smith RG, McClatchey KD, Annesley T, England B, et al. Occupational cadmium exposure and renal status. Am J Ind Med 1983;4:541-9.
- Driscoll KE, Maurer JK, Poynter J, Higgins J, Asquith T, Miller NS. Stimulation of rat alveolar macrophage fibronectin release in a cadmium chloride model of lung injury and fibrosis. Toxicol Appl Pharmacol 1992;116:30-7.
- Waalkes MP. Cadmium carcinogenesis in review. J Inorg Biochem 2000;79:241-4.
- Chan WH, Lu HY, Shiao NH. Effect of genistein on mouse blastocyst development in vitro. Acta Pharmacol Sin 2007;28:238-45.