Salvianolic acid B promotes survival of transplanted mesenchymal stem cells in spinal cord-injured rats1
Introduction
Traumatic spinal cord injury (SCI) can result in severe damage, leading to paraplegia, tetraplegia, or worse. Many strategies, including surgical, pharmacological, neurophysiological, and technological approaches, have been used to allow patients to regain the use of their paralyzed limbs. One such strategy is cell transplantation into the damaged spinal cord. Cell transplantation not only has the ability to provide therapeutic agents, it also has the potential to repair and replace neuronal tissue. Stem cells have greatly increased the hope that treatments may be available for once untreatable central nervous system (CNS) diseases and injures, which has captured the attention of both the scientific community and the general public. Mesenchymal stem cells (MSC), a type of adult stem cell, can be easily acquired, maintained, expanded quickly, and differentiated into neural cell types in vitro and in vivo. It was reported that transplanted MSC could promote the functional recovery of spinal cord injury in animals, including primates and other mammals[1−3].
However, the adult CNS, especially the injured CNS, may provide a relatively non-permissive environment for transplanted MSC and other stem cells. Even under the best circumstances, cell survival in injured CNS has been estimated to be near 10%[4−6], and few cells differentiate into mature neuronal phenotypes[7−10]. One of the most important reasons why those cells could not survive in the injured CNS is probably due to the sustained inflammation in the injury sites or the immediate inflammatory response elicited by surgery and injection manipulation[8,11]. Methods to provide neuroprotection support to the transplanted cells, by suppressing the inflammation in the injured spinal cord and brain, may benefit long-term behavioral outcomes and the grafted cell survival.
Salvianolic acid B (Sal B) is a water-soluble compound extracted from Salvia miltiorrhiza (SM), a herb that was clinically used for thousands of years in traditional Chinese medicine. SM has been used as a common treatment medicine for stroke, and is now widely used for the treatment of cardiovascular diseases. Previous studies have demonstrated that SM is an anti-inflammatory, antioxidative, and apoptosis-inducing plant[12,13]. Also, Sal B has been reported to exert protective action against TNF-α injury in human aortic vascular endothelial cells[14]. Sal B offers an interesting possible approach for SCI treatment, because it plays an important cytoprotective role and reduces inflammatory responses, thereby affecting a variety of processes that contribute to secondary degeneration. Indeed, the neuroprotective effects of Sal B have been demonstrated in some experimental models of cerebral ischemia and brain injury[15−17].
Thus, the aim of the present study was to investigate the effect of Sal B on the recovery of motor function of SCI rats and whether Sal B could enhance MSC survival in an in vitro environment with TNF-α or in SCI rats.
Materials and methods
MSC isolation and culture MSC were collected from femurs of adolescent, male Sprague–Dawley (SD) rats (60–80g) as reported[18]. Briefly, the bilateral femurs and tibias were harvested and the marrow was flushed out by using a syringe filled with Dulbecco’s modified Eagle’s medium/F12 containing 10% fetal bovine serum (FBS). The bone marrow was plated in 25 cm2 culture flasks. The flasks were incubated at 37 °C with 5% CO2. Forty eight hours after plating, the supernatant containing non-adherent cells was removed and fresh medium was added. After growing near 80%–90% confluency, the cells were passaged 2 or 3 times by detachment (0.25% trypsin/0.02% EDTA for 2–3 min) and replated. The cells were then frozen in aliquots and stored in liquid nitrogen.
Analysis of the protective function of Sal B on MSC in vitro Sal B (molecular formula: C36H30O16, molecular weight: 746, purity: 98.5%, Green-Valley, Shanghai, China) was kept from direct exposure to light and air during the experiments. Sal B and recombinant rat TNF-α (PeproTechEC, London, UK) were dissolved in phosphate buffered saline (PBS).
Cell viability was determined by counting the number of attached cells on a culture plate. MSC (at passages 4–8), cultured in 12-well plates at 1×105 /plate for 2 d, were treated as follows. MSC were treated with 10 ng/mL TNF-α for 24 h. Sal B 100 ng/mL was added, along with the TNF-α, to protect MSC from injury resulting from10 ng/mL TNF-α in one group, while no Sal B was used in the other group. Cell death was observed under a microscope and characterized by rounding up the cells and detaching from the plates. After treatment, each well was photographed at least 5 times by a digital camera. The attached cell numbers were counted from 3 randomly-selected pictures.
Animal group design A total of 42 adult, female SD rats (weight 220–250 g) were used. The experimental rats were divided depending on the treatment of rats after thoracic SCI: Sal B group, Sal B injected intraperitoneally on d 0, 1, 2, and 3, post-injury, n=6; PBS group, PBS injected intraperitoneally on d 0, 1, 2, and 3, post-injury, n=6; MSC group, MSC transplanted in situ on d 7, post-injury, and PBS injected intraperitoneally at d 7, 8, and 9, post-injury, n=9; MSC plus Sal B group, MSC transplanted in situ on d 7, post-injury, and Sal B injected intraperitoneally on d 7, 8, and 9, post-injury, n=9; and the PBS control group, PBS transplanted in situ on d 7, post-injury, n=6.
Thoracic SCI Laminectomy was performed at T8−T10 under 10% chloral hydrate anesthesia. The impact rod, weighing 10 g, was centered above T9 and dropped from a height of 25 mm to induce a consistent partial, incomplete SCI. Dural tear was not observed following the impact. Muscle and skin were sutured by layer after injury. Gentamicin (8 mg·kg-1·d-1, ip) was given to prevent urinary tract infection for 3 d, and lactated Ringer’s solution was administered to avoid dehydration (2 mL, ip, after surgery). Urinary bladders were emptied manually 2 times per day until recovery of urinary function. In the present study, the animal experiments were performed in accordance with the National Institute of Health animal care and use guidelines.
Sal B treatment and MSC transplantation For the Sal B and PBS groups, the rats were given Sal B (8 mg/kg) or PBS only, intraperitoneally, at different time points as mentioned earlier.
For the MSC group and the MSC plus Sal B group, the rats were treated with Sal B or MSC from d 7, post-injury as mentioned. Before the experimental manipulations, MSC were labeled with bromodeoxyuridine (BrdU; 100 µmol/L, Boster, Wuhan, China) for 48 h to permit later identification. This procedure led to 80%–90% BrdU labeling of the cultures. The flasks were washed and the cells were resuspended in PBS. Before transplantation, MSC were spun down and resuspended in a microcentrifuge tube in PBS, approximately 100 000 cells/µL, and kept on ice. A 25 gauge Hamilton syringe was lowered into the upper region central of the injured site at approximately 5 mm. MSC suspension or only PBS filled in the syringe in 10 µL was injected at rate of 2.5 µL/min. The syringe was allowed to remain in the position for several minutes after the injection to allow the cells to settle. The incision was then closed with nylon suture. Animal care was carried out until the rats were killed.
Tissue harvest and histological evaluation Histological evaluation was performed at the endpoints of the different groups in the experiment. The animals were anesthetized with 10% chloral hydrate and perfused transcardially with 4% paraformaldehyde. The T8-10 portion of the spinal cord was removed from the vertebral column and was then immersed for 24 h in the same fixative. The tissue was embedded in paraffin and sectioned sagittally (6 µm thickness).
For the Sal B and PBS groups, sections were collected on microscope slides, the paraffin was removed in xylene, and the slides were rehydrated through graded ethanol. All sections were stained with hematoxylin–eosin (HE) for general histology. The area of cavitation was measured by setting the optical density threshold to delineate regions lacking cellular components with the outlined lesion.
For the MSC group, MSC plus Sal B group, and the PBS control group, the paraffin of sections was removed in xylene, and the slides were rehydrated through graded ethanol. Half the sections were used for immunohistochemical staining to determine the survival of grafted MSC. Other sections were stained with HE for general histology.
Immunohistochemical detection of the survival of grafted MSC and quantification analysis After rinsing in PBS, the sections were exposed to 3% H2O2 for 30 min to quench endogenous peroxidase activity. Before the incubation of the primary antibodies, non-specific binding was blocked for 1 h with 3% normal serum from the species in which the secondary antibody was raised with 0.25% Triton X-100 in PBS. The primary antibodies used were directed against the rabbit anti-BrdU monoclonal antibody (1:100, Boster, China). The sections were incubated with secondary antibodies conjugated to biotin (1:100, Boster, China). Subsequently, the sections incubated with secondary antibodies conjugated to biotin were washed in PBS and incubated with a avidin-biotin-horseradish peroxidase complex (1:100, Boster, China). 3’,3’-Diaminobenzidine (Boster, China) was used as a chromogen. Control slides lacking primary or secondary antibodies were analyzed with each series. The sections were studied using light microscopy.
Behavioral testing The rats were tested behaviorally to evaluate the effect of MSC and Sal B on the recovery of motor function of the injured rats. Behavioral testing was performed for each hind limb using the Basso-Beatie-Bresnahan (BBB) scale at different time points (before injury and on d 1, 7, 14, 21, 28, and 35, post-injury)[9]. BBB scores categorize combinations of rat hind limb movements, trunk position and stability, stepping, coordination, paw placement, toe clearance, and tail position; a 0 score represents no locomotion and a 21 score represents normal motor function. The test was conducted by 2 independent observers, and the scores for the left and right leg were averaged to generate the actual score for each trial.
Statistical analysis The statistical analysis was calculated using SPSS software (SPSS, Chicago, IL, USA). All values are expressed as the mean±SEM. The results were analyzed by one-way ANOVA followed by a Bonferroni post-hoc test for multiple comparisons. A P-value of <0.05 was considered significant.
Results
Effects of Sal B on rats with spinal cord injuries A representative bright-field photomicrograph of the spinal cord sections following PBS control or Sal B treatments were selected to express the effect of Sal B treatment on SCI (Figure 1A,1B). For the measurement of cavities, sagittal sections were stained with HE, and the area of the cavity in the spinal cord was measured. The areas of the cavities were measured from 20 sections in every rat in the Sal B and PBS groups. The cavity area was then calculated by the average area in the 2 groups (n=6 each group). Sal B treatment significantly reduced the cavity area from 0.26±0.05 mm2 to 0.15±0.03 mm2, compared with the control groups (P<0.01, Figure 1C).
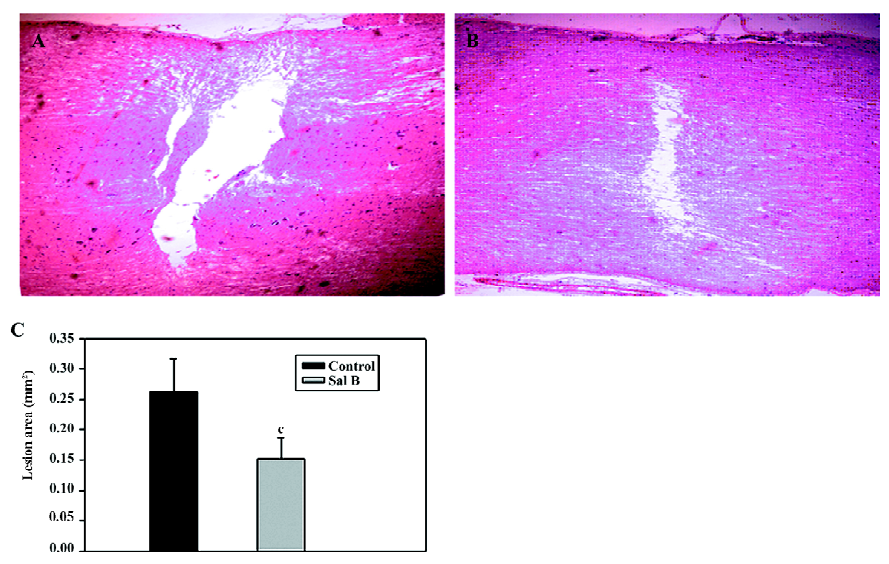
Performance in locomotor function was enhanced in the Sal B-treated animals. In contrast to the inability of the control animals to support their weight with their hind limbs, the Sal B-treated rats demonstrated partial weight-supported ambulation. A statistical difference in the BBB scores was achieved 2 weeks after injection. After 4 weeks, there was a significant difference of approximately 2 points on the BBB scale between the Sal B and PBS groups (Figure 5A).
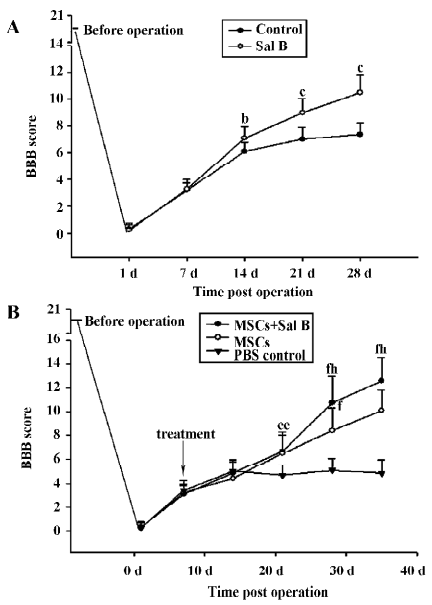
Sal B protects MSC from the injury of TNF-α in vitro The effect of Sal B on the cell death of MSC induced by TNF-α was investigated. MSC were killed with TNF-α, and cell death was determined by a microscopic observation of cell shrinkage and detachment from the culture plates. The cells began to shrink and detach from the culture plates 3 h after incubation with TNF-α. Within 24 h, a large number of cells were detached from the culture plates. However, TNF-α had no effect on MSC treated with Sal B (Figure 2A–2C).
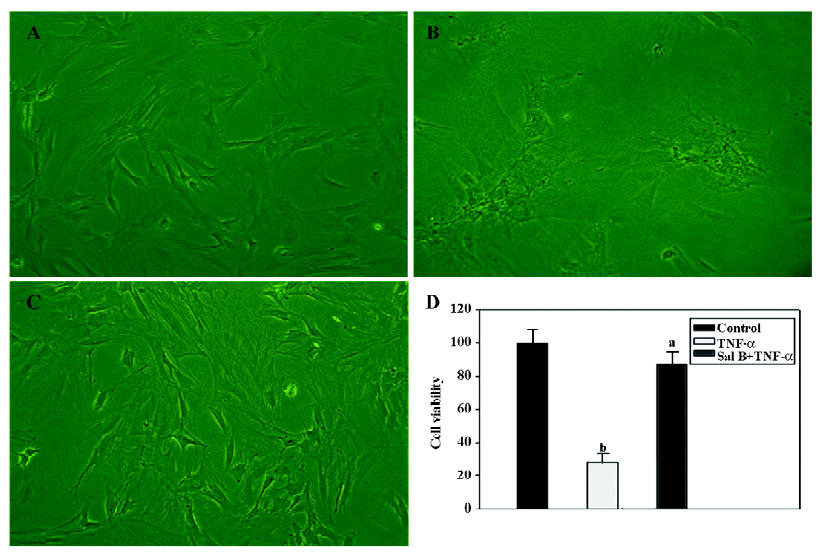
Cell viability (%) was determined by comparing the cell numbers of the control with those of the treated cells. Data are presented as mean±SEM from 3 separate experiments. According to the counting result, TNF-α significantly decreased the cell viability by 65% compared with the control level. In contrast, Sal B plus TNF-α treatment had no significant effect on the viability of the cells (Figure 2D).
Sal B promotes the survival of MSC in spinal cord-injured rats On d 7, post-transplantation, 4 animals from each group were used, and 5 animals from each group were used on d 28, post-transplantation. For the evaluation of the number of the grafted MSC, 2 representative sections at the center of the lesion were selected from each MSC-grafted animal in the MSC group and the MSC plus Sal B group. In the MSC group, few surviving MSC with intact BrdU nuclei were found and these were scattered throughout the graft at d 28, post-transplantation (Figure 3A). In contrast, in the MSC plus Sal B group, many more surviving MSC (dark brown or black) were densely packed throughout the transplantation site (Figure 3B).
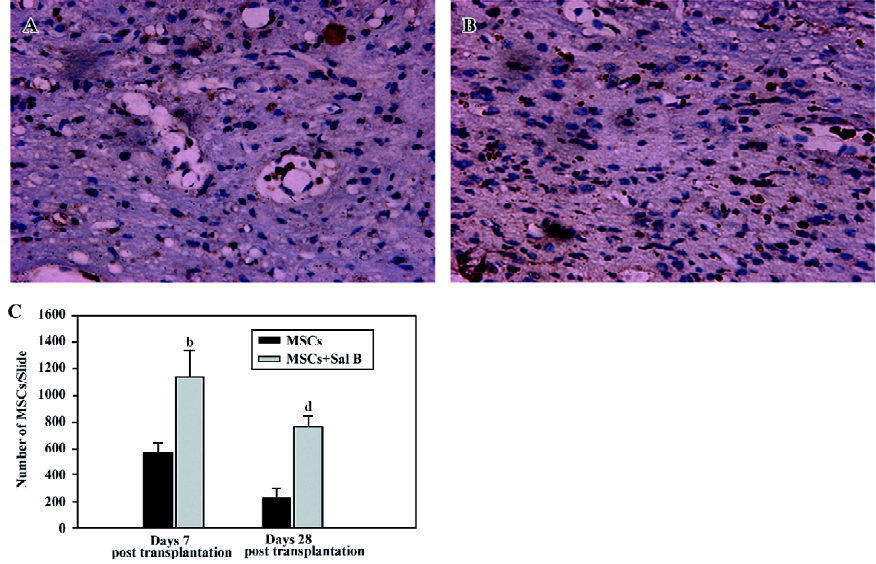
BrdU-labeled MSC were quantified from slides from 3 sections with the center of the lesion in each group (Figure 3C). The MSC plus Sal B group contained significantly more positive cells (1143.3±195.6) than the MSC group (569.3±72.3; (P<0.05), at d 7, post-transplantation. The quantification of MSC in the 2 groups at d 28, post-transplantation was also done. A greater number of surviving MSC (764.0±81.3) in the MSC plus Sal B group was found than that in the MSC group (237.0±61.3). The number of surviving MSC at d 28 was fewer than that at d 7 in the MSC plus Sal B group, but more MSC were still found in the MSC plus Sal B group compared with MSC in the MSC group (P<0.05).
The anatomical integrity of injured spinal cords in the PBS control group, MSC group, and MSC plus Sal B group were evaluated. Large cavities were found in the spinal cord of rats in the PBS control group (Figure 4A). Fewer cavities were found in the injured spinal cord of rats in the MSC group (Figure 4B), but tissue structure seemed to be porous. The anatomical integrity of the injured spinal cord of the rats in the MSC plus Sal B group (Figure 4C) was better than those in the MSC group, which suggested that the enhancement of MSC survival could promote the restoration of anatomical integrity of injured spinal cords.
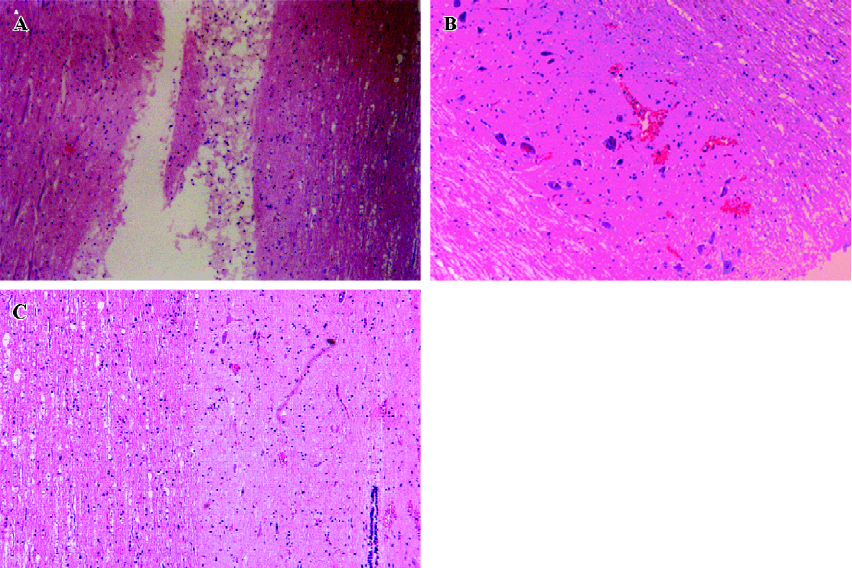
The effect of MSC with or without Sal B treatment on motor function recovery was assessed by the BBB scale (Figure 5B). Treated animals generally showed better performance of gait than the PBS control animals. MSC plus Sal B-treated rats showed remarkable recovery (weight supporting plantar steps and consistent coordination between the forelimbs and hind limbs) on d 35, post-operation (BBB score 12.6±2.0). In contrast, MSC- treated rats had a BBB score of 10.1±1.8. From d 28, post-operation, there were significant differences between the MSC-treated animals and the MSC plus Sal B-treated animals (P<0.05).
Discussion
The present study provides evidence that Sal B improves the recovery of motor function after contusive spinal cord injury in rats, and significantly reduces the cavity area. The rats that received Sal B at a dose of 8 mg/kg displayed a more rapid recovery of BBB assessment performance than the control rats receiving PBS only. The ability of Sal B to improve motor function is probably partially due to its protective effect on neural cells injured by contusion. This could be because more neurons were left in the rats treated with Sal B than in the control rats. The study also showed that MSC were protected from TNF-α damage by the use of Sal B in vitro, and Sal B promoted the survival of MSC in vivo. The results in these studies suggested that Sal B may provide neuroprotection to the transplanted MSC in SCI rats.
Sal B treatment may inhibit the increase of the level of TNF-α in the damaged spinal cord after injury. TNF-α directly activates neutrophils and increases the expression of E-selectin, which contribute to the formation of cavities and tissue injury[20,21]. Whether Sal B treatment reduces the production of TNF-α should be detected by RT–PCR and Western blotting in future studies.
One possibility of the benefits of Sal B treatment is that Sal B induced the expression of a particular set of genes. Neuroprotective genes, such as brain-derived neurotrophic factors, are induced in a variety of conditions, such as brain injury, cerebral ischemia, and electrical stimulation[22,23]. Hence, if neuroprotective genes are directly induced by Sal B, the factors that mediate that induction remain to be defined. Any of these alterations in gene expression could play a role in enhancing locomotor behavior and protecting spinal cords against a progressive series of necrosis and cavitation after injury.
It is known that the non-permissive environment caused by immediate injury and second injury may have an adverse effect on MSC transplantation to the injured spinal cord. Because of this, many researchers selected d 7–10, post-injury, as the transplantation time, however, MSC survival was still not sufficient[6]. One of likely reasons for this, as discussed by Coyne et al[11], was that injections may elicit dangerous inflammatory reactions, which may cause the transplanted cells to die. In our study, few transplanted MSC survived on d 14, post-injury. Sal B treatment was conducted when MSC were transplanted into the injured rats in the present study. The results suggest Sal B promotes MSC survival on d 35, post-injury. It was estimated that injections may cause the production of TNF-α, and Sal B might protect MSC in vivo.
In conclusion, the study suggests that Sal B provides neuroprotection to SCI and can promote the survival of MSC in vitro and after their transplantation to the injured spinal cord. These findings suggest that a combination of Sal B and MSC may be an effective way in the treatment of SCI.
References
- Deng YB, Yuan QT, Liu XG, Liu XL, Liu Y, Liu ZG, et al. Functional recovery after rhesus monkey spinal cord injury by transplantation of bone marrow mesenchymal-stem cell-derived neurons. Chin Med J (Engl) 2005;118:1533-41.
- Deng YB, Liu XG, Liu ZG, Liu XL, Liu Y, Zhou GQ. Implantation of BM mesenchymal stem cells into injured spinal cord elicits de novo neurogenesis and functional recovery: evidence from a study in rhesus monkeys. Cytotherapy 2006;8:210-4.
- Urdzikova L, Jendelova P, Glogarova K, Burian M, Hajek M, Sykova E. Transplantation of bone marrow stem cells as well as mobilization by granulocyte-colony stimulating factor promotes recovery after spinal cord injury in rats. J Neurotrauma 2006;23:1379-91.
- Pincus DW, Keyoung HM, Harrison-Restelli C, Goodman RR, Fraser RA, Edgar M, et al. Fibroblast growth factor-2/brain-derived neurotrophic factor-associated maturation of new neurons generated from adult human subependymal cells. Ann Neurol 1998;43:576-85.
- Vescovi AL, Snyder EY. Establishment and properties of neural stem cell clones: plasticity in vitro and in vivo. Brain Pathol 1999;9:569-98.
- Zompa EA, Cain LD, Everhart AW, Moyer MP, Hulsebosch CE. Transplant therapy: recovery of function after spinal cord injury. J Neurotrauma 1997;14:479-506.
- Park KI, Liu S, Flax JD, Nissim S, Stieg PE, Snyder EY. Transplantation of neural progenitor and stem cells: developmental insights may suggest new therapies for spinal cord and other CNS dysfunction. J Neurotrauma 1999;16:675-87.
- Fricker-Gates RA, Winkler C, Kirik D, Rosenblad C, Carpenter MK, Bjorklund A. EGF infusion stimulates the proliferation and migration of embryonic progenitor cells transplanted in the adult rat striatum. Exp Neurol 2000;165:237-47.
- Studer L, Tabar V, McKay RD. Transplantation of expanded mesencephalic precursors leads to recovery in Parkinsonian rats. Nat Neurosci 1998;1:290-5.
- Svendsen CN, Clarke DJ, Rosser AE, Dunnett SB. Survival and differentiation of rat and human epidermal growth factor-responsive precursor cells following grafting into the lesioned adult central nervous system. Exp Neurol 1996;137:376-88.
- Coyne TM, Marcus AJ, Woodbury D, Black IB. Marrow stromal cells transplanted to the adult brain are rejected by an inflammatory response and transfer donor labels to host neurons and glia. Stem Cells 2006;24:2483-92.
- Lei XL, Chiou GC. Studies on cardiovascular actions of Salvia miltiorrhiza. Am J Chin Med 1986;14:26-32.
- Lay IS, Hsieh CC, Chiu JH, Shiao MS, Lui WY, Wu CW. Salvianolic acid B enhances in vitro angiogenesis and improves skin flap survival in Sprague–Dawley rats. J Surg Res 2003;115:279-85.
- Chen YH, Lin SJ, Ku HH, Shiao MS, Lin FY, Chen JW, et al. Salvianolic acid B attenuates VCAM-1 and ICAM-1 expression in TNF-alpha-treated human aortic endothelial cells. J Cell Biochem 2001;82:512-21.
- Chen YH, Du GH, Zhang JT. Salvianolic acid B protects brain against injuries caused by ischemia-reperfusion in rats. Acta Pharmacol Sin 2000;21:463-6.
- Du GH, Qiu Y, Zhang JT. Salvianolic acid B protects the memory functions against transient cerebral ischemia in mice. J Asian Nat Prod Res 2000;2:145-52.
- Tang M, Feng W, Zhang Y, Zhong J, Zhang J. Salvianolic acid B improves motor function after cerebral ischemia in rats. Behav Pharmacol 2006;17:493-8.
- Nagaya N, Fujii T, Iwase T, Ohgushi H, Itoh T, Uematsu M, et al. Intravenous administration of mesenchymal stem cells improves cardiac function in rats with acute myocardial infarction through angiogenesis and myogenesis. Am J Physiol Heart Circ Physiol 2004;287:H2670-6.
- Basso DM, Beattie MS, Bresnahan JC. A sensitive and reliable locomotor rating scale for open field testing in rats. J Neurotrauma 1995;12:1-21.
- Mulligan MS, Varani J, Dame MK, Lane CL, Smith CW, Anderson DC, et al. Role of endothelial-leukocyte adhesion molecule 1 (ELAM-1) in neutrophil-mediated lung injury in rats. J Clin Invest 1991;88:1396-406.
- Taoka Y, Okajima K. Spinal cord injury in the rat. Prog Neurobiol 1998;56:341-58.
- Taoka Y, Okajima K, Uchiba M, Murakami K, Harada N, Johno M, et al. Activated protein C reduces the severity of compression-induced spinal cord injury in rats by inhibiting activation of leukocytes. Neurosci 1998;18:1393-8.
- Tsuchiya D, Hong S, Matsumori Y, Kayama T, Swanson RA, Dillman WH, et al. Overexpression of rat heat shock protein 70 reduces neuronal injury after transient focal ischemia, transient global ischemia, or kainic acid-induced seizures. Neurosurgery 2003;53:1179-87.