Multipotent natural agents to combat Alzheimer’s disease. Functional spectrum and structural features1
Introduction
Since the discovery of Alzheimer’s disease (AD) in 1907, considerable effort has been devoted to combating the disease. However, up to now, there is no effective therapeutics. With the accelerated aging of human society, AD is becoming one of the biggest threats to human health[1–3]. Although the etiology of AD is not very clear, multiple pathogenetic factors have been identified for the disease, which include amyloid-β (Aβ) peptide and/or τ protein aggregation, excessive metal ions (eg, Cu2+, Zn2+, Fe3+), oxidative stress and reduced acetylcholine (ACh) level, etc[1–8]. Besides, genetic factors and lifestyles, such as diet, exercise and cognitive stimulation, are also associated with AD development[9,10].
Despite the diverse pathogenetic factors involved in AD, the current anti-AD strategy depends largely on single-targeted drugs, especially acetylcholinesterase (AChE) inhibitors. As these drugs’ effects are quite limited[11], more and more attention is given to fin d multiple-targeted agents to hit more than one target implicated in AD[12,13]. Although the new anti-AD strategy may be fulfilled by combining different anti-AD drugs in one pill (cocktail therapeutics)[14,15], an alternative approach that aims at multiple AD-targets with a single structure (termed multipotent agent) is also attractive, because of its advantages in reducing risks of drug-drug interactions and controlling pharmacokinetic behaviors[15].
Thanks to the continuing effort of medicinal chemists in the past decade, many multipotent anti-AD agents have been rationally designed by incorporating two or more pharmacophores in one scaffold, in which the pharmacophores for inhibiting AChE were most widely used[12,13]. For instance, Rosini et al[16] designed a hybrid compound (lipocrine) (Figure 1) by linking tacrine, an AChE inhibitor, and lipoic acid, a universal antioxidant. Rodríguez-Franco et al coupled tacrine to melatonin, a pineal neurohormone and a preventive antioxidant (Figure 1)[17]. These hybrid molecules exhibited markedly enhanced activity with respect to AChE inhibition and antioxidant properties compared with either of the original molecules[16,17]. By joining together the pharmacophores for inhibiting AChE and monoamine oxidases B (MAO-B), ie, carbamate and propargyl group, Sterling et al[18] also obtained novel dual inhibitors of AChE and MAO.
Despite the preliminary successes of synthetic hybrid agents, the latent risks in safety and bioavailability is a big concern in their further development. Thus, finding multipotent natural agents to combat AD is attracting more and more attention.
Multipotent anti-AD agents derived from foods
In the past few years, some epidemiological investigations revealed that high consumption of some foods (or beverages) were inversely associated with AD incidence[19–25]. These foods include fruit and vegetable juices, green tea, wine, Mediterranean diet, curry spice turmeric and even cigarettes, all of which contain antioxidants, especially polyphenols. As it is well known that polyphenols are excellent antioxidants both as reactive oxygen species (ROS) scavengers and transition metal chelators[26,27], the anti-AD effects of these foods were naturally linked to their antioxidant potential. Nevertheless, accumulating evidence indicates that the excellent in vitro antioxidant activity of phenols can not necessarily be translated into in vivo therapeutic effects[28,29]. Thus, it is interesting to note that some antioxidants derived from these foods go beyond modulating ROS. Some representative examples are given as below.
Flavonoids Flavonoids are the most extensively studied polyphenols derived from fruit and vegetable juices and green tea. Multiple pharmacological effects have been identified for flavonoids, many of which are beneficial to combat AD. For instance, quercetin (Figure 2), the representative component of fruit and vegetable juices, can block Aβ- or τ-aggregation (IC50s < 1 µmol/L)[30], inhibit monoamine oxidases A and B (MAO A and MAO B) with IC50s of 0.01 µmol/L and 10.89 µmol/L, respectively[31,32]. In addition, quercetin can efficiently inhibit butyrylcholinesterase (BChE) (with an IC50 of 1 µmol/L)[33], a recently recognized potential target for treating AD[34]. As quercetin is highly bioavailable and can pass through the blood-brain barrier (BBB)[35,36], it seems partially responsible for the benefits of fruit and vegetable juices to AD.
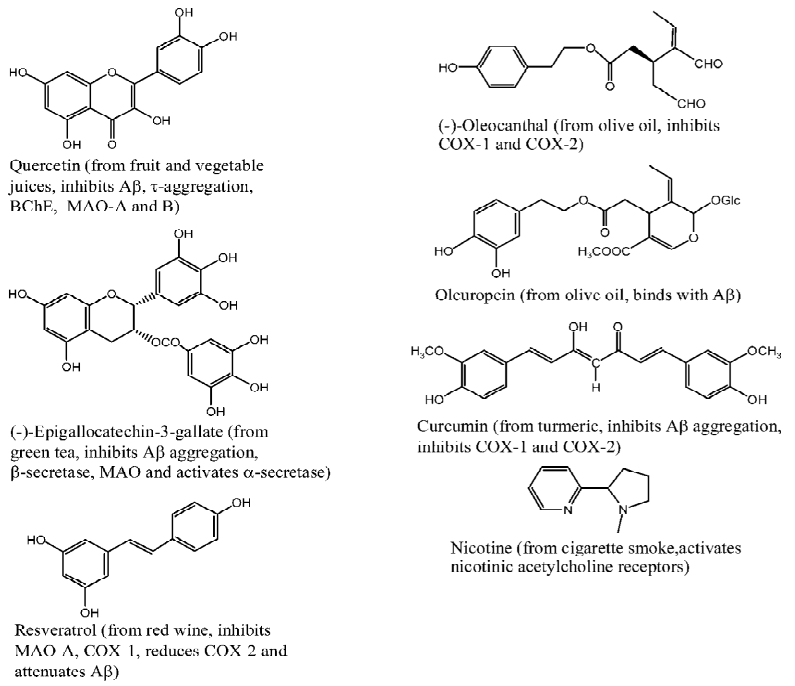
(-)-Epigallocatechin gallate (EGCG) (Figure 2) is the representative component of green tea. Some potential anti-AD effects of EGCG have been identified as follows: i) it is a powerful Aβ-aggregation inhibitor with an IC50 of 0.18 µmol/L[30]; ii) it attenuates Aβ generation through activating α-secretase[37,38], inhibiting β-secretase (BACE1, with an IC50 of 1.6 µmol/L)[39] and reducing iron-regulated amyloid precursor protein expression[40]; iii) it inhibits MAO with an IC50 of 10 µmol/L[41]. All of these pharmacological effects are helpful to understand the preventive effects of green tea to AD.
Resveratrol Resveratrol (Figure 2) is a famous phenolic component extracted from red wine, which has been extensively studied in the past 15 years. Some pharmacological effects that are associated with AD treatment have been revealed. First, resveratrol can lower the levels of secreted and intracellular Aβ by promoting protease degradation of the peptide[42]. Second, resveratrol inhibits monoamine oxidase A (MAO-A) with an IC50 of 26.6 µmol/L[43]. Third, resveratrol can inhibit cyclooxygenase-1 (COX-1) with an IC50 of 24 µmol/L[44] and reduce cyclooxygenase-2 (COX-2) at mRNA level[45].
Olive oil phenols Mediterranean diet consists of olive oil, fruits, vegetables and fish, of which olive oil is of special interest and has been a research focus for decades. Many phenolic compounds have been identified from olive oil[46], some of which exhibit nonsteroidal anti-inflammatory drug-like activities that are beneficial to prevent AD. For instance, Beauchamp et al revealed that 25 µmol/L (-)-oleocanthal (Figure 2) inhibited 56.1%±3.2% and 56.6%±9.5% COX-1 and COX-2 activity, respectively[47]. In addition, Bazoti et al found that oleuropein (Figure 2) can form noncovalent complex with Aβ peptide or its oxidized form[48].
Curcumin Curcumin (Figure 2), a yellow-orange pigment extracted from curry spice turmeric, has long been used as a food additive in India. Many pharmacological effects have been identified for this pigment[49]. Besides its famous transition metal-chelating ability and anti-inflammatory activity[50–52], curcumin holds Aβ aggregation-blocking potential (with an IC50 of < 1 µmol/L)[30] and COX-1-, COX-2-inhibiting activities (with IC50s of 18.8 µmol/L and 15.9± 7.9 µmol/L, respectively).[53,54]
Nicotine Nicotine (Figure 2) is the predominant component of cigarette smoke, which is considered responsible for the cigarettes’ benefits to AD. Recently, Zhao and co-workers revealed that nicotine attenuated the β-amyloid neurotoxicity through regulating metal (copper and zinc) homeostasis[55] and activating nicotinic acetylcholine receptors[56,57]. Combining experimental findings and theoretical calculation results, we indicated that the copper (II)-nicotine chelates hold SOD-like activity, which may play a role in the neuroprotective effects of nicotine[58].
Multipotent anti-AD agents derived from herbs
It is not surprising to note that in addition to foods, some herbal medicines, such as G Biloba, Huperzia serrata, Salvia officinalis, Melissa officinalis, also hold anti-AD potential, as revealed by some preliminary clinical trials[59–64]. Some ingredients responsible for the anti-AD effects have been identified from these herbs.
Extract EGb761 (extract G biloba 761), prepared from the leaves of G biloba and comprising flavonoids and terpene lactones, was a hot spot of medicinal research in the past two decades. EGb761 has many pharmacological effects in favor of the fight against AD, which include inhibiting Aβ aggregation, attenuating apoptosis, preventing membrane lipid from oxidation and resisting inflammation[65].
Huperzine A (HupA) (Figure 3), an alkaloid isolated from Chinese herb Huperzia serrata, is also a potent multipotent anti-AD agent, with activities of inhibiting AChE (with an IC50 of 0.082 µmol/L)[60], mitigating oxidative stress, regulating the expression of apoptotic proteins Bcl-2, Bax, p53, and caspase-3, interfering with amyloid precursor protein metabolism and so on, which definitely benefits the neuro-protection[60,61].
Rosmarinic acid (Figure 3) is likely to be one of the major active ingredients of Salvia officinalis and Melissa officinalis, which exhibits a combination of antioxidative, anti-Aβ aggregation and antiapoptotic effects[66].
Recently, xanthones, a special kind of flavonoids that spread widely in nature, were also found possessing high anti-AD potential. Indeed, some xanthone-containing herbs, such as Polygala tenuifolia, show benefits to prevent AD[67]. A representative of multipotent anti-AD xanthone is presented in Figure 3. It can inhibit MAO-A (with an IC50 of 0.04 µmol/L), MAO-B (with an IC50 of 33.0 μmol/L) and AChE (with a Ki of 16.0 µmol/L)[68,69]. Because of the perfect conjugation of the ring system and the electron-withdrawing property of 1,4-pyrone (the central ring)[70,71], xanthones are weaker electron donors than flavonoids[72], which implies that xanthones are safer than flavonoids with respect to the prooxidant potential.
Structural features of naturally occurring multipotent anti-AD agents
From the structures of rational designed and naturally occurring multipotent anti-AD agents, it can be found that both kinds of compounds are different in scaffold, that is, the latter shows a seamless framework (Figures 2 and 3), while the former is composed of two or more isolated parts, linked by spacers of different lengths, with each part aiming at a particular target (Figure 1). So, it is of significance to explore the natural strategy of “designing” multipotent anti-AD agents.
Through examining the structures of above-mentioned multipotent natural agents, we can find that most of them are phenolics. It is well known that phenolic hydroxyls are the most potent groups to neutralize ROS through donating H-atoms[73] and also effective to chelate transition metal ions[26]. However, the present description shows that natural phenolics go beyond scavenging ROS or chelating transition metals. They can inhibit various enzyme’s activities and prevent protein aggregation. The major underlying reason may be that phenolic hydroxyls are H-bond acceptors and H-bond donors simultaneously, which facilitates the binding with protein targets. This explanation is supported by a meta-analysis on phenol-protein binding patterns which revealed that more than 70% phenolic hydroxyls form intermolecular hydrogen bonds (IHBs) with targeted proteins[28]. Another feature of the naturally occurring multipotent anti-AD agents is that their structures contain more than one conjugated rings (most are phenolic rings) and most of the conjugated systems are still flexible. Thus, these molecules reach a good balance between rigidity and flexibility, which must benefit their binding with various targets. To illustrate the importance of these structural features in binding diverse target proteins, some multipotent agents were docked with corresponding targets. As shown in Figures 4,5,6,7,8, the phenolic hydroxyls indeed tend to form IHBs with surrounding residues and the flexible structures favor the binding between agents and proteins. Finally, since p-stacking plays an important role in protein amyloid formation[30,74], the aromaticity of phenolic ring is favorable to prohibit amyloid fibril formation[75].
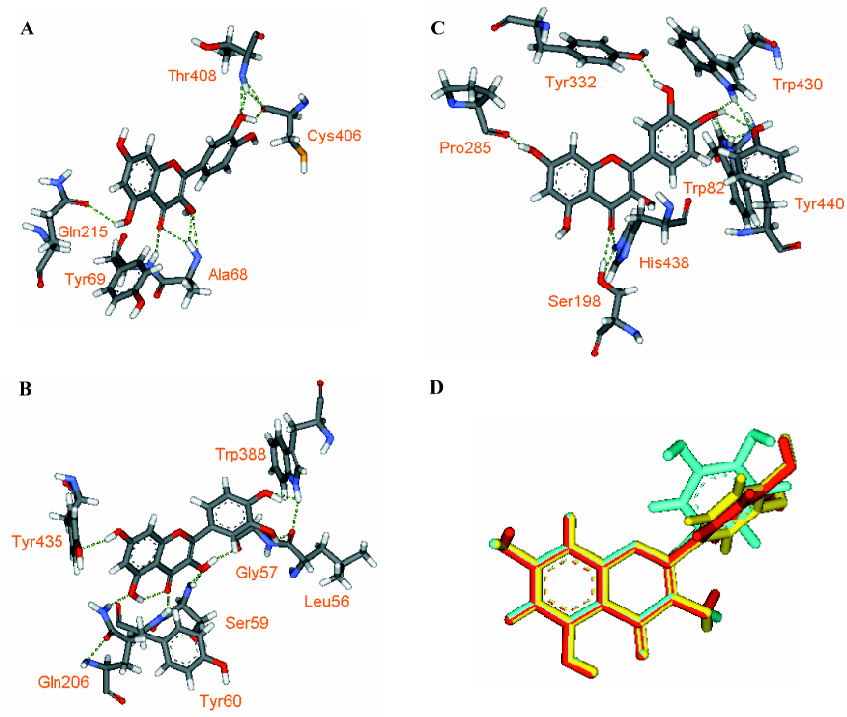
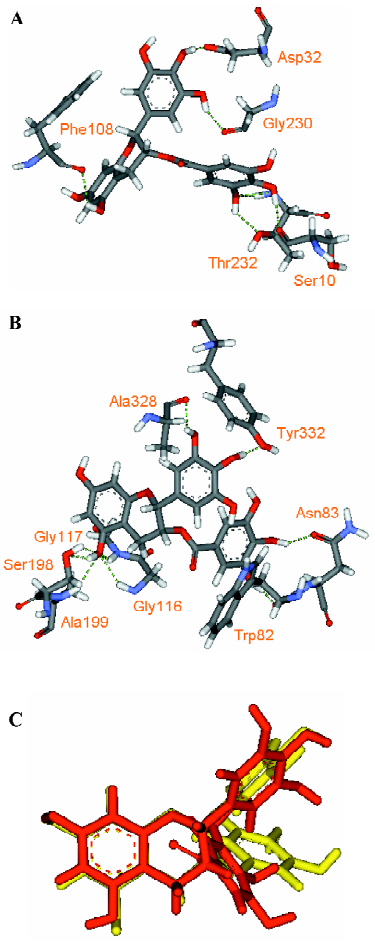
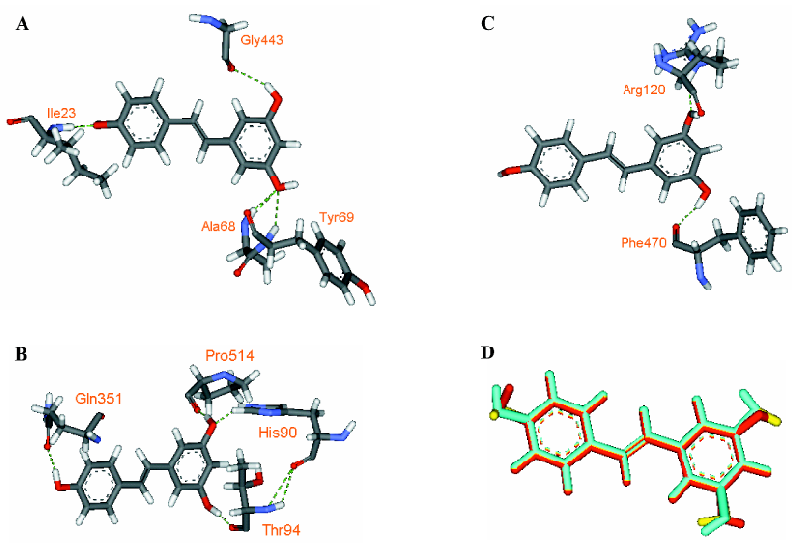
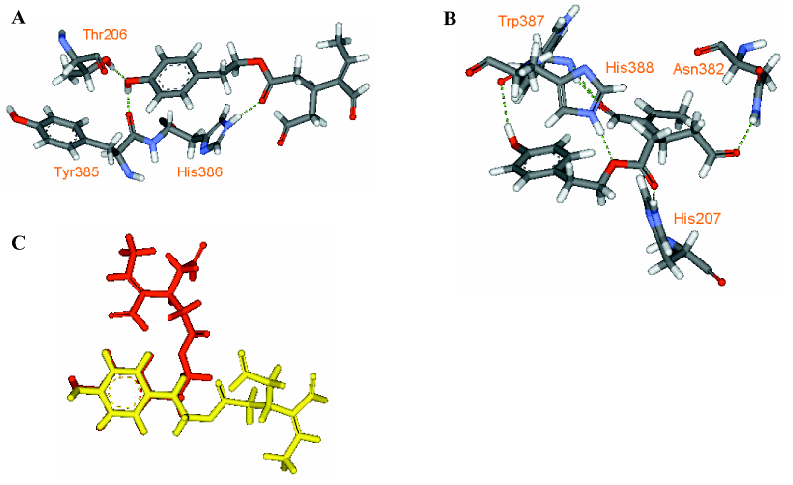
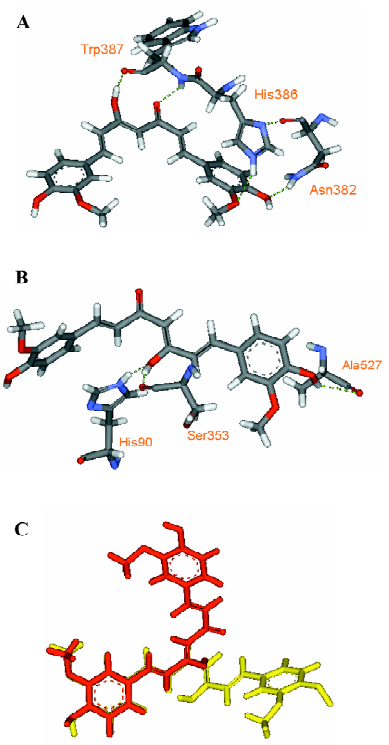
Conclusion
Thanks to the continuing efforts of medicinal chemists and pharmacologists in the past decade, more and more natural agents that can hit multiple targets implicated in AD (eg, Aβ, τ protein, AChE, BChE, MAO, COX, α-, and β-secretases, ROS and transition metals) were identified. As most of these agents are bioavailable and can penetrate blood-brain barrier (BBB) (at least in animal models)[35,36,76–79], they are likely responsible for the AD-preventing effects of the source plants, as revealed by epidemiological investigations and preliminary clinical trials. Thus, these agents are good starting points for finding novel anti-AD drugs. Through examining the structures of these agents, it was revealed that phenolics with certain flexibility are preferred by the naturally occurring multipotent anti-AD agents, which has important implications for screening and design of novel multipotent anti-AD drugs.
Considering the fact that current knowledge about natural products is very limited, we think that the presently identified natural multifunctional agents are the tip of the iceberg. With the increase of information on natural medicines, more and more pleiotropic anti-AD compounds will be discovered from medicinal plants in China and/or other geographical regions.
The present analysis also has significance to find drugs for other neurodegenerative diseases, such as prion diseases, Parkinson’s disease, and amyotrophic lateral sclerosis, because these diseases are also characterized by progressive neuronal loss and involve similar multiple pathogenetic factors (eg, protein aggregation, transition metals and excessive ROS)[5,6,76,80] and different types of soluble amyloid oligomers bear a common structure[81].
References
- Mattson MP. Pathways towards and away from Alzheimer’s disease. Nature 2004;430:631-9.
- Cummings JL. Alzheimer’s disease. N Eng J Med 2004;351:56-67.
- Benson A. Alzheimer’s disease: a tangled issue. Drug Discov Today 2005;10:749-51.
- Barnham KJ, Masters CL, Bush AI. Neurodegenerative diseases and oxidative stress. Nat Rev Drug Discov 2004;3:205-14.
- Brown DR, Kozlowski H. Biological inorganic and bioinorganic chemistry of neurodegeneration based on prion and Alzheimer diseases. Dalton Trans 2004.1907-17.
- Citron M. Strategies for disease modification in Alzheimer’s disease. Nat Rev Neurosci 2004;5:677-85.
- Thorsett ED, Latimer LH. Therapeutic approaches to Alzheimer’s disease. Curr Opin Chem Biol 2000;4:377-82.
- Forman MS, Trojanowski JQ, Lee VMY. Neurodegenerative diseases: a decade of discoveries paves the way for therapeutic breakthroughs. Nat Med 2004;10:1055-63.
- Hardy J, Orr H. The genetics of neurodegenerative diseases. J Neurochem 2006;97:1690-9.
- Pope SK, Shue VM, Beck C. Will a healthy lifestyle help prevent Alzheimer’s disease? Annu Rev Public Health 2003;24:111-32.
- Maggini M, Vanacore N, Raschetti R. Cholinesterase inhibitors: drugs looking for a disease? PLoS Med 2006;3:e140.
- Zhang HY. One-compound-multiple-targets strategy to combat Alzheimer’s disease. FEBS Lett 2005;579:5260-4.
- Youdiim MB, Buccafusco JJ. Multi-functional drugs for various CNS targets in the treatment of neurodegenerative disorders. Trends Pharmacol Sci 2005;26:27-35.
- Prasad KN, Hovland AR, Cole WC, Prasad KC, Nahreini P, Edwards-Prasad J, et al. Multiple antioxidants in the prevention and treatment of Alzheimer disease: analysis of biologic rationale. Clin Neuropharmacol 2000;23:2-13.
- Youdim MBH, Buccafusco JJ. CNS Targets for multi-functional drugs in the treatment of Alzheimer’s and Parkinson’s diseases. J Neural Transm 2005;112:519-37.
- Rosini M, Andrisano V, Bartolini M, Bolognesi ML, Hrelia P, Minarini A, et al. Rational approach to discover multipotent anti-Alzheimer drugs. J Med Chem 2005;48:360-3.
- Rodríguez-Franco MI, Fernández-Bachiller MI, Pérez C, Hernández-Ledesma B, Bartolomé B. Novel tacrine-melatonin hybrids as dual-acting drugs for Alzheimer disease, with improved acetylcholinesterase inhibitory and antioxidant properties. J Med Chem 2006;49:459-62.
- Sterling J, Herzig Y, Goren T, Finkelstein N, Lerner D, Goldenberg W, et al. Novel dual inhibitors of AChE and MAO derived from hydroxy aminoindan and phenethylamine as potential treatment for Alzheimer’s disease. J Med Chem 2002;45:5260-79.
- Dai Q, Borenstein AR, Wu Y, Jackson JC, Larson EB. Fruit and vegetable juices and Alzheimer’s disease: The Kame project. Am J Med 2006;119:751-9.
- Kuriyama S, Hozawa A, Ohmori K, Shimazu T, Matsui T, Ebihara S, et al. Green tea consumption and cognitive function: a cross-sectional study from the Tsurugaya Project. Am J Clin Nutr 2006;83:355-61.
- Truelsen T, Thudium D, Grønbæk M. Amount and type of alcohol and risk of dementia: the Copenhagen city heart study. Neurology 2002;59:1313-9.
- Scarmeas N, Stern Y, Tang MX, Mayeux R, Luchsinger JA. Mediterranean diet and risk for Alzheimer’s disease. Ann Neurol 2006;59:912-21.
- Ng TP, Chiam PC, Lee T, Chua HC, Lim L, Kua EH. Curry consumption and cognitive function in the elderly. Am J Epidemiol 2006;164:898-906.
- Brenner DE, Kukull WA, van Belle G, Bowen JD, McCormick WC, Teri L, et al. Relationship between cigarette smoking and Alzheimer’s disease in a population-based case-control study. Neurology 1993;43:293-300.
- Salib E, Hillier V. A case–control study of smoking and Alzheimer’s disease. Int J Geriatr Psychiatry 1997;12:295-300.
- Rice-evans CA, Miller NJ, Paganga G. Structure-antioxidant activity relationships of flavonoids and phenolic acids. Free Radic Biol Med 1996;20:933-56.
- Zhang HY. Structure-activity relationships and rational design strategies for radical-scavenging antioxidants. Curr Comput-Aided Drug Design 2005;1:257-73.
- Shen L, Ji HF, Zhang HY. How to understand the dichotomy of antioxidants? Biochem Biophys Res Commun 2007;362:543-5.
- Azzi A. Oxidative stress: A dead end or a laboratory hypothesis? Biochem Biophys Res Commun 2007;362:230-2.
- Porat Y, Abramowitz A, Gazit E. Inhibition of amyloid fibril formation by polyphenols: structural similarity and aromatic interactions as a common inhibition mechanism. Chem Biol Drug Des 2006;67:27-37.
- Lee MH, Lin RD, Shen LY, Yang LL, Yen KY, Hou WC. Monoamine oxidase B and free radical scavenging activities of natural flavonoids in Melastoma candidum D. Don. J Agric Food Chem 2001;49:5551-5.
- Chimenti F, Cottiglia F, Bonsignore L, Casu L, Casu M, Floris C, et al. Quercetin as the active principle of Hypericum hircinum exerts a selective inhibitory activity against MAO-A: extraction, biological analysis and computational study. J Nat Prod 2006;69:945-9.
- Salvi A, Brülmann C, Migliavacca E, Carrupt PA, Hostettmann K, Testa B. Protein protection by antioxidants: development of a convenient assay and structure-activity relationships of natural polyphenols. Helv Chim Acta 2002;85:867-81.
- Greig NH, Utsuki T, Ingram DK, Wang Y, Pepeu G, Scali C, et al. Selective butyrylcho-linesterase inhibition elevates brain acetylcholine, augments learning and lowers Alzheimer β-amyloid peptide in rodent. Proc Natl Acad Sci USA 2005;102:17213-8.
- O’reilly JD, Mallet AI, McAnlis GT, Young IS, Halliwell B, Sanders TA, et al. Consumption of flavonoids in onions and black tea: lack of effect on F2-isoprostanes and autoantibodies to oxidized LDL in healthy humans. Am J Clin Nutr 2001;73:1040-4.
- Youdim KA, Shukitt-Hale B, Josephy JA. Flavonoids and the brain: interactions at the blood-brain barrier and their physiological effects on the central nervous system. Free Radic Biol Med 2004;37:1683-93.
- Rezai-Zadeh K, Shytle D, Sun N, Mori T, Hou H, Jeanniton D, et al. Green tea epigallocatechin-3-gallate (EGCG) modulates amyloid precursor protein cleavage and reduces cerebral amyloidosis in Alzheimer transgenic mice. J Neurosci 2005;25:8807-14.
- Obregon DF, Rezai-Zadeh K, Bai Y, Sun N, Hou H, Ehrhart J, et al. ADAM10 activation is required for green tea-EGGC-induced -secretase cleavage of amyloid precursor protein. J Biol Chem 2006;281:16419-27.
- Jeon SY, Bae K, Seong YH, Song KS. Green tea catechins as a BACE1 (β-secretase) inhibitor. Bioorg Med Chem Lett 2003;13:3905-8.
- Reznichenko L, Amit T, Zheng H, Avramovich-Tirosh Y, Youdim MBH, Weinreb O, et al. Reduction of iron-regulated amyloid precursor protein and β-amyloid peptide by (-)-epigallo-catechin-3-gallate in cell cultures: implications for iron chelation in Alzheimer’s disease. J Neurochem 2006;97:527-36.
- Mazzio EA, Harris N, Soliman KF. Food constituents attenuate monoamine oxidase activity and peroxide levels in C6 astrocyte cells. Planta Med 1998;64:603-6.
- Marambaud P, Zhao H, Davies P. Resveratrol promotes clearance of Alzheimer’s disease amyloid-β peptides. J Biol Chem 2005;280:37377-82.
- Zhou CX, Kong LD, Ye WC, Cheng CHK, Tan RX. Inhibition of xanthine and monoamine oxidases by stilbenoids from Veratrum taliense. Planta Med 2001;67:158-61.
- Shin NH, Ryu SY, Lee H, Min KR, Kim Y. Inhibitory effects of hydroxystilbenes on cyclooxygenase from sheep seminal vesicles. Planta Med 1998;64:283-4.
- Baur JA, Sinclair DA. Therapeutic potential of resveratrol: the in vivo evidence. Nature Rev Drug Discov 2006;5:493-506.
- Saija A, Uccella N. Olive biophenols: functional effects on human wellbeing. Trends Food Sci Tech 2001;11:357-63.
- Beauchamp GK, Keast RSJ, Morel D, Lin J, Pika J, Han Q, et al. Phytochemistry: ibuprofen-like activity in extra-virgin olive oil. Nature 2005;437:45-6.
- Bazoti FN, Bergquist J, Markides KE., Tsarbopoulos A. Noncovalent interaction between amyloid-β-peptide (1-40) and oleuropein studied by electrospray ionization mass spectrometry. J Am Soc Mass Spectrom 2006;17:568-75.
- Aggarwal BB, Sundaram C, Malani N, Ichikawa H. Curcumin: the Indian solid gold. Adv Exp Med Biol 2007;595:1-75.
- Frautschy SA, Hu W, Kim P, Miller SA, Chu T, Harris-White ME, et al. Phenolic anti-inflammatory antioxidant reversal of Abeta-induced cognitive deficits and neuropathology. Neurobiol Aging 2001;22:993-1005.
- Barik A, Mishra B, Shen L, Mohan H, Kadam RM, Dutta S, et al. Evaluation of new copper-curcumin complex as superoxide dismutase mimic and its free radical reactions. Free Radic Biol Med 2005;39:811-22.
- Shen L, Zhang HY, Ji HF. A theoretical study on Cu(II)-chelating properties of curcumin and its implications for curcumin as a multipotent agent to combat Alzheimer’s disease. J Mol Struct (Theochem) 2005;757:199-202.
- Gafner S, Lee SK, Cuendet M, Barthélémy S, Vergnes L, Labidalle S, et al. Biologic evaluation of curcumin and structural derivatives in cancer chemoprevention model systems. Phytochemistry 2004;65:2849-59.
- Ramsewak RS, DeWitt DL, Nair MG. Cytotoxicity, antioxidant and anti-inflammatory activities of curcumins I-III from Curcuma longa. Phytomedicine 2000;7:303-8.
- Zhang J, Liu Q, Chen Q, Liu NQ, Li FL, Lu ZB, et al. Nicotine attenuates the β-amyloid neurotoxicity through regulating metal homeostasis. FASEB J 2006;20:E399-E408.
- Liu Q, Zhao BL. Nicotine attenuates beta-amyloid peptide-induced neurotoxicity, free radical and calcium accumulation in hippocampal neuronal cultures. Br J Pharmacol 2004;141:745-54.
- Liu Q, Zhang J, Zhu H, Qin C, Chen Q, Zhao BL. Dissecting the signaling pathway of nicotine-mediated neuroprotection in a mouse Alzheimer disease model. FASEB J 2007;21:61-73.
- Shen L, Zhang HY, Ji HF. Computational note on the SOD-like antioxidant potential of nicotine-copper(II) complexes. J Mol Struct (Theochem) 2007;817:161-2.
- Mazza M, Capuano A, Bria P, Mazza S. Ginkgo biloba and donepezil: a comparison in the treatment of Alzheimer’s dementia in a randomized placebocontrolled double-blind study. Eur J Neurol 2006;13:981-5.
- Wang R, Yan H, Tang XC. Progress in studies of huperzine A, a natural cholinesterase inhibitor from Chinese herbal medicine. Acta Pharmacol Sin 2006;27:1-26.
- Zhang HY, Tang XC. Neuroprotective effects of huperzine A: new therapeutic targets for neurodegenerative disease. Trends Pharmacol Sci 2006;27:619-25.
- Akhondzadeh S, Noroozian M, Mohammadi M, Ohadinia S, Jamshidi AH, Khani M. Salvia officinalis extract in the treatment of patients with mild to moderate Alzheimer’s disease: a double blind, randomized and placebo-controlled trial. J Clin Pharm Ther 2003;28:53-9.
- Perry NS, Bollen C, Perry EK, Ballard C. Salvia for dementia therapy: review of pharmacological activity and pilot tolerability clinical trial. Pharmacol Biochem Behav 2003;75:651-9.
- Akhondzadeh S, Noroozian M, Mohammadi M, Ohadinia S, Jamshidi AH, Khani M. Melissa officinalis extract in the treatment of patients with mild to moderate Alzheimer’s disease: a double blind, randomised, placebo controlled trial. J Neurol Neurosurg Psychiatry 2003;74:863-6.
- Luo Y. Alzheimer’s disease, the nematode Caenorhabditis elegans, and Ginkgo biloba leaf extract. Life Sci 2006;78:2066-72.
- Izzo AA, Capasso F. Herbal medicines to treat Alzheimer’s disease. Trends Pharmacol Sci 2006;28:47-8.
- Wang CJ, Lu JD, Liu QF. 51 dementia cases treated by Yizhiling. Acta Med Sin 1993;8:33-4.
- Zhang HY, Sun YM, Wang XL. Substituent effects on O-H bond dissociation enthalpies and ionization potentials of catechols: a DFT study and its implications in rational design of phenolic antioxidants and elucidation of structure-activity relationships for flavonoid antioxidants. Chem Eur J 2003;9:502-8.
- Zhang HY. Theoretical elucidation of structure-activity relationship of flavonoid antioxidants. Sci China 1999;42:106-12. (series B).
- Gnerre C, Thull U, Gaillard P, Carrupt PA, Testa B, Fernandes E, et al. Natural and synthetic xanthones as monoamine oxidase inhibitors: biological assay and 3D-QSAR. Helv Chim Acta 2001;84:552-70.
- Brühlmann C, Marston A, Hostettmann K, Carrupt PA, Testa B. Screening of non-alkaloidal natural compounds as acetylcholinesterase inhibitors. Chem Biodivers 2004;1:819-29.
- Ji HF, Tang GY, Zhang HY. Theoretical elucidation on structure-activity relationships of antioxidant xanthones. QSAR Combin Sci 2005;24:826-30.
- Antunes F, Barclay LRC, Ingold KU, King M, Norris JQ, Scaiano JC, et al. On the antioxidant activity of melatonin- association with nuclear grade and estrogen receptor status. Free Radic Biol Med 1999;26:117-28.
- Gazit E. A possible role for π-stacking in the self-assembly of amyloid fibrils. FASEB J 2002;16:77-83.
- Zhang HY. Same causes, same cures. Biochem Biophys Res Commun 2006;351:578-81.
- Breuer C, Wolf G, Andrabi SA, Lorenz P, Horn TFW. Blood-brain barrier permeability to the neuroprotectant oxyresveratrol. Neurosci Lett 2006;393:113-8.
- Lin LC, Wang MN, Tseng TY, Sung JS, Tsai TH. Pharmacokinetics of (-)-epigallocatechin-3-gallate in conscious and freely moving rats and its brain regional distribution. J Agric Food Chem 2007;55:1517-24.
- Suganuma M, Okabe S, Oniyama M, Tada Y, Ito H, Fujiki H. Wide distribution of [3H](-)-epigallocatechin gallate, a cancer preventive tea polyphenol, in mouse tissue. Carcinogenesis 1998;19:1771-6.
- Yang FS, Begum A, Lim G, Ambegaokar S, Ubeda O, Chen PP, et al. Curcumin binds Aβ, inhibits Aβ aggregation and fibril formation in vitro and crosses the BBB to label plaques and reduces Aβ accumulation in aged APPSW transgenic mice. Neurobiol Aging 2004;25 Suppl 2:S158.
- Ji HF, Zhang HY, Chen LL. Why are prion diseases precluded by non-mammals? Trends Biochem Sci 2007;32:206-8.
- Kayed R, Head E, Thompson JL, McIntire TM, Milton SC, Cotman CW, et al. Common structure of soluble amyloid oligomers implies common mechanism of pathogenesis. Science 2003;300:486-9.