Anti-inflammatory effect of honokiol is mediated by PI3K/Akt pathway suppression1
Introduction
Macrophages and lymphocytes play important roles in the host immune defense mechanism composed of innate and adaptive immunity[1]. The functional roles of these cells are known to be mediated by various soluble factors, such as cytokines and toxic molecules[2,3], having various defensive roles against pathogens and tumors[3,4]. However, certain conditions with the overproduction of inflammatory molecules can give the host severe immunopathological symptoms, such as acute and chronic inflammatory diseases[5]. Because of this, the effective modulation of the overproduction state has been considered a therapeutic target. The production of inflammatory molecules is triggered by mitogenic stimulation with various bacterial products, such as lipopolysaccharide (LPS). Owing to numerous studies, the molecular events of the inflammatory process have been widely understood. In particular, various signaling cascades composed of pattern recognition receptors [eg Toll-like receptor (TLR)-4], non-receptor type tyrosine kinases (eg Src and Syk), serine/threonine kinases [protein kinase C (PKC), protein kinase A (PKA), phosphatidylinositol-3-kinase (PI3K), and Akt], mitogen-activated protein kinases (MAPK), and various redox-sensitive transcription factors, such as NF-κB, are regarded as critical components participating in the processes[6,7].
Honokiol and magnolol (isomers of hydroxylated biphenolic compounds [C18H18O2, molecular weight =266.33]; Figure 1) are major components isolated from the bark of the root and stem of various Magnolia species[8]. In particular, since these plants have been used in traditional herbal medicines against a variety of inflammatory and neuronal diseases, these compounds were initially thought of as major active compounds of the plants[9,10]. Indeed, these compounds have been demonstrated to possess various pharmacological effects, such as neuroprotective, anticancer, cardiotonic, and anti-inflammatory activities[10–13]. How these compounds are able to modulate various pharmacological actions has not yet been fully elucidated yet, but upregulating a signaling cascade composed of Ras, Raf, and the extracellular signal-regulated kinase (ERK)[14,15], downregulating the activation of NF-κB by the inhibition of its upstream kinases (IκB kinase [IKK]) under TNF-α stimulation and ERK kinase kinase-1 under LPS stimulation[16–18], and blocking NF-κB activation mediated by CD40 and latent membrane protein 1[19] are speculated to be the action mechanism of the compound.
Nonetheless, the exact pathways and molecular target of honokiol under inflammatory conditions are still controversial. Therefore, in this study, we carefully examined the inhibitory effect of honokiol (and magnolol) on various inflammatory responses mediated by mitogenic stimulation as well as its molecular target in the process of immunopharmacological actions.
Materials and methods
Materials Honokiol and magnolol (Figure 1) were obtained from Nacalai Tesque (Kyoto, Japan). Dibutyryl cyclic adenosine monophosphate (dbcAMP), phorbol 12-myrostate-13-acetate (PMA), N-monomethyl-L-arginine, curdlan, peptidoglycan (PGN), recombinant human interferon (IFN)-γ, and LPS (Escherichia coli 0111:B4) were purchased from Sigma (St Louis, MO, USA). U0126 and LY294002 were obtained from Calbiochem (La Jolla, CA, USA). Fetal bovine serum, penicillin, streptomycin, and RPMI-1640 were obtained from GIBCO (Grand Island, NY, USA). RAW264.7 cells, a murine macrophage cell line, U937 cells, a human promonocytic cell line, and CTLL-2 cells, a mouse cytotoxic interleukin (IL)-2-dependent T cell line, were purchased from American Type Culture Collection (Rockville, MD, USA). All other chemicals were of Sigma grade. CD29 [MEM 101A, immunoglobulin G (IgG)1], CD43 (161-46, IgG1), CD62L (Dreg56, IgG1)[20] were used for the cell–cell adhesion assays and flow cytometric analyses. Phospho-specific antibodies to Src, p85, Akt, IκBα, p38, ERK, and an antibody to β-actin were purchased from Cell Signaling (Beverly, MA, USA).
Cell culture The RAW264.7 and U937 cells were maintained in RPMI-1640 supplemented with 100 U/mL penicillin, 100 µg/mL streptomycin, and 10% fetal bovine serum. In the case of CTLL-2 cell maintenance, the cells were cultured with the same medium containing 25 U/mL IL-2. The cells were grown at 37 °C and 5% CO2 in humidified air.
Determination of LPS-induced TNF-α production The inhibitory effects of honokiol and magnolol on TNF-α production from the LPS-treated RAW264.7 cells was determined as described previously[21]. The amount of TNF-α was assayed by ELISA.
Determination of prostaglandin E2 production The inhibitory effects of honokiol on prostaglandin E2 (PGE2) production from the LPS-treated RAW264.7 cells was determined as described previously[21]. The amount of PGE2 was assayed by ELISA.
Determination of nitric oxide production The inhibitory effects of honokiol on nitric oxide (NO) production from the LPS-treated RAW264.7 cells was determined as described previously[21]. The amount of NO was assayed by a Griess assay, as reported previously.
MTT assay (colorimetric assay) for the measurement of cell viability and proliferation Cell proliferation was measured by conventional 3-(4,5-dimethylthiazol-2-yl)-2,5-diphenyltetrazolium bromide (MTT) assay, as reported previously. The culture was stopped by the addition of 15% sodium dodecyl sulfate into each well for the solubilization of formazan. The optical density (OD) at 570 nm (OD570–630) was measured by a Spectramax 250 microplate reader (Molecular Devices, Sunnyvale, CA, USA).
Determination of LPS-inducible gene expression (inducible NO synthase, TNF-α, MCP-1, IL-12, p40, cyclooxygenase-2, and GAPDH) For the evaluation of the cytokine mRNA expression levels, the total RNA from the LPS-treated RAW264.7 cells was prepared by adding TRIzol Reagent (GIBCO, USA), according to manufacturer’s protocol. Semiquantitative RT reactions were conducted, as reported previously. The primers (Bioneer, Seoul, Korea) used in this experiment are indicated in Table 1.
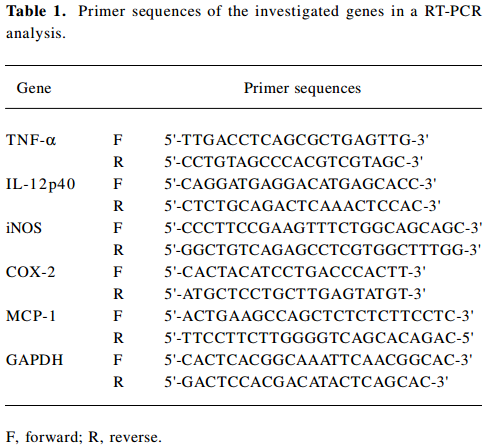
Full table
Flow cytometric analysis The expression of the cell surface adhesion and costimulatory molecules in U937 (CD29 and CD43) and RAW264.7 (CD80) cells was determined by a flow cytometric analysis, as reported previously[22]. Stained cells were analyzed on a FACScan (Beckton-Dickinson, San Jose, CA, USA).
Cell-cell or cell-extracellular matrix protein (fibronectin) adhesion assay The inhibitory effect of honokiol on cell–cell adhesion was determined by a quantitative U937 cell–cell adhesion assay with function-activating (agonistic) antibodies (1 μg/mL) as described previously[22,23]. For a cell–fibronectin adhesion assay, the U937 cells (5×105 cells/well) were seeded on a fibronectin (50 μg/mL)-coated plate and incubated for 3 h[24]. After removing the unbound cells with phosphate-buffered saline, the attached cells were treated with 0.1% crystal violet for 15 min. The OD value at 570 nm was measured by a Spectramax 250 microplate reader.
Lymphocyte proliferation assay The splenocytes were prepared from the spleens of mice killed by cervical dislocation under sterile conditions, as described previously[21]. Briefly, the splenocytes were released by teasing into RPMI-1640 medium supplemented with 20 mmol/L N-[2-hydroxyethyl]piperazine-N'-[2-ethanesulfonic acid] (HEPES) buffer. The splenocytes (5×106 cells/mL) were cultured in 96-well plates in the presence and absence of T or B lymphocyte mitogens [concanavalin A (Con A), phytohemaglutinin A (PHA), and LPS] with honokiol in a total volume of 200 μL/well at the same conditions for 48 h. The proliferation assay was performed by MTT assay.
Preparation of cell lysates and immunoblotting The total cell lysates were prepared from the cells, as reported previously[22]. The protein concentrations were determined by the Bradford method[25]. The lysates were clarified by centrifugation at 16 000×g for 10 min at 4 ℃. Soluble cell lysates were immunoblotted, and the phospho forms of p38, ERK, Akt, and IκBα were visualized, as reported previously.
Statistical analysis Data were expressed as mean±SEM. For the statistical comparison, the results were analyzed using ANOVA and the Kruskal-Wallis test. A P-value <0.05 was considered a statistically significant difference. All statistical tests were carried out using the computer program STATISTICA version 4.5 (StatSoft, Microsoft, Oklahoma City, OK, USA).
Results
Effect of honokiol on the viability of RAW264.7 cells It was necessary to assess the cytotoxic effect of honokiol before further in vitro tests were carried out. The MTT assay using RAW264.7 and U937 cells is a suitable model for this purpose, as the cells are representative immune cells. Figure 2 shows that honokiol was significantly non-cytotoxic up to 20 μmol/L in both RAW264.7 (left panel) and U937 cells (right panel) under our 12 or 24 h incubation conditions, while more than 40 μmol/L concentrations time-dependently altered the viability of RAW264.7 and U937 cells. Thus, 40 μmol/L of this compound was non-cytotoxic in both cell lines until 3 h, but long exposure (12 and 24 h) significantly induced cytotoxic activity. Therefore, it was regarded that the anti-inflammatory effects of honokiol at less than 20 μmol/L could be due to the selective pharmacological action of the drug without any interference with normal cell function. However, for the immunoblotting and cell–fibronectin experiments, which require 30 min or 3 h incubation with honokiol, 40 μmol/L conditions were used in these experiments to obtain remarkable effects.
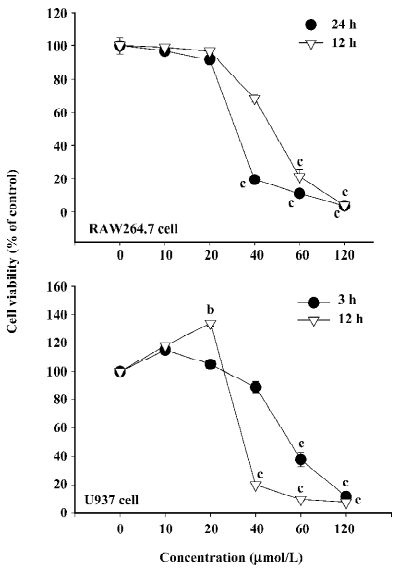
Effect of honokiol on LPS-mediated pro-inflammatory responses by macrophages To evaluate the inhibitory effect of honokiol (and magnolol) on LPS-mediated pro-inflammatory responses by macrophages, the production of pro-inflammatory mediators, surface levels of the costimulatory molecule CD80, and the mRNA expression of the pro-inflammatory genes were determined under LPS and honokiol treatment. Honokiol strongly inhibited the release of TNF-α (Figure 3A), PGE2 (Figure 3B), and NO (Figure 3C) in a dose-dependent manner. However, magnolol, its structural analog, suppressed NO and TNF-α production more weakly than honokiol. Furthermore, honokiol significantly diminished the LPS-induced surface upregulation of the costimulatory molecule CD80, indicating that honokiol may commonly affect LPS-mediated pro-inflammatory responses. Indeed, this compound blocked the mRNA expression of pro-inflammatory genes, such as inducible NO synthase (iNOS), MCP-1, cyclooxygenase (COX)-2, and TNF-α (Figure 3E), suggesting that the immunomodulatory effect of honokiol may be occurring at the transcriptional level.
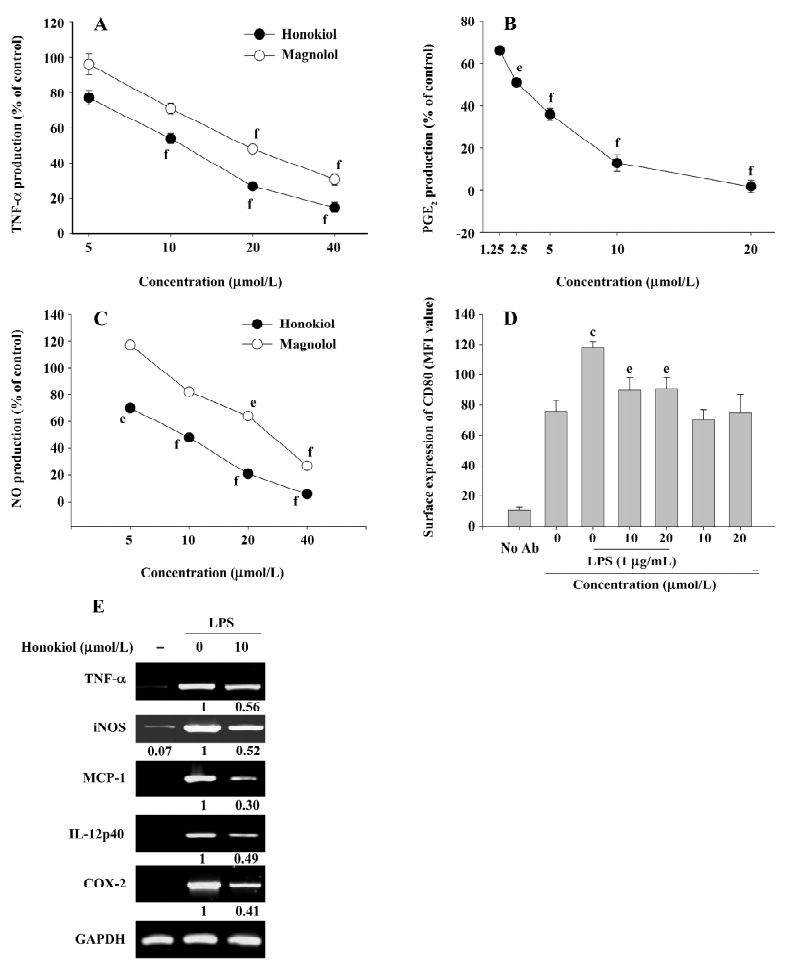
Positive control drugs for TNF-α production used in this experiment, prednisolone and dbcAMP, also significantly suppressed TNF-α production in a dose-dependent manner with IC50 values of 28.9 and 47.4 μmol/L, respectively. Indomethacin also strongly suppressed PGE2 production with an IC50 value of 3.3 μmol/L, as reported previously[26–28]. The control drug N-MMA inhibited NO release from LPS-stimulated RAW264.7 cells with an IC50 value of 193.3 µmol/L.
Effect of honokiol on CD29-mediated cell–cell and cell-fibronectin adhesion To assess the regulatory effect of honokiol on monocyte adhesion to other cells or the extracellular matrix (eg fibronectin) mediated by β1-integrins (CD29), quantitative homotypic U937 cell-cell and cell-fibronectin adhesion assays were employed. As shown in Figure 4A, honokiol dose-dependently blocked U937 cell–cell adhesion induced by the agonistic antibody to CD29, but not CD43. Furthermore, this compound also downregulated cell-fibronectin adhesion in a dose-dependent manner (Figure 4B). To determine whether the suppressive effect of honokiol is due to the downregulation of the surface level of CD29, a flow cytometric analysis was performed. However, there was no alteration of the surface levels of CD29 and CD43 after 3 h incubation (Figure 4C), indicating that the diminishment may occur by interrupting intracellular signaling levels, playing a critical role in cell–cell adhesion.
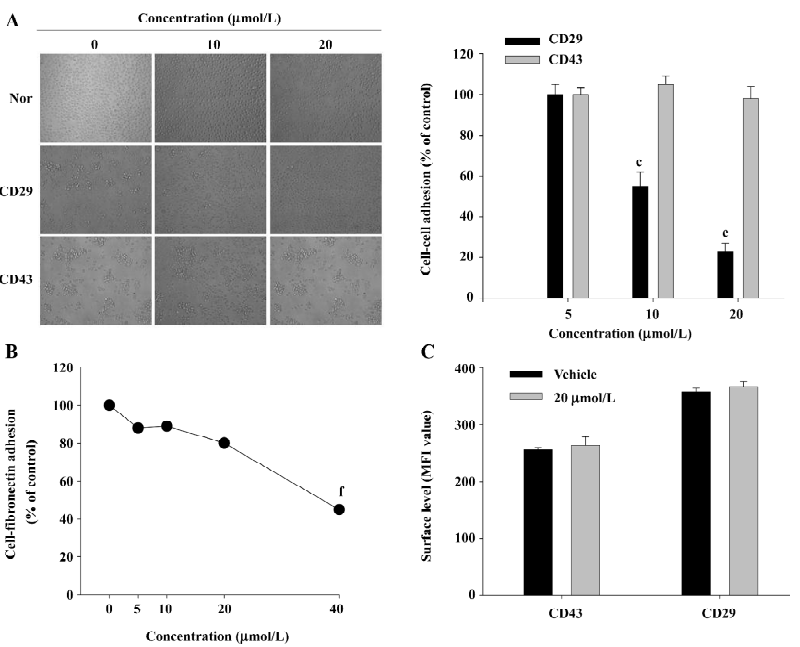
Effect of honokiol on mitogenic response of splenic lymphocytes and IL-2-dependent CTLL-2 cells To assess whether honokiol is able to modulate the mitogenic cellular events of other immune cells, such as lymphocytes, splenic lymphocytes, IL-2-dependent CD8+CTLL-2 cells, and mitogens (such as LPS, Con A, PHA, and IL-2) were employed. As shown in Figure 5A, honokiol clearly suppressed splenic lymphocyte proliferation induced by all mitogens tested when they were treated with 12.5 μmol/L for 48 h, whereas magnolol showed weak effects and enhanced the proliferation at 6.25 μmol/L. In particular, the inhibitory pattern was also similarly observed in the IL-2-dependent proliferation of CTLL-2 cells. Thus, honokiol, but not magnolol, strongly inhibited CD8+CTLL-2 cell proliferation triggered by IL-2 in a dose-dependent manner.
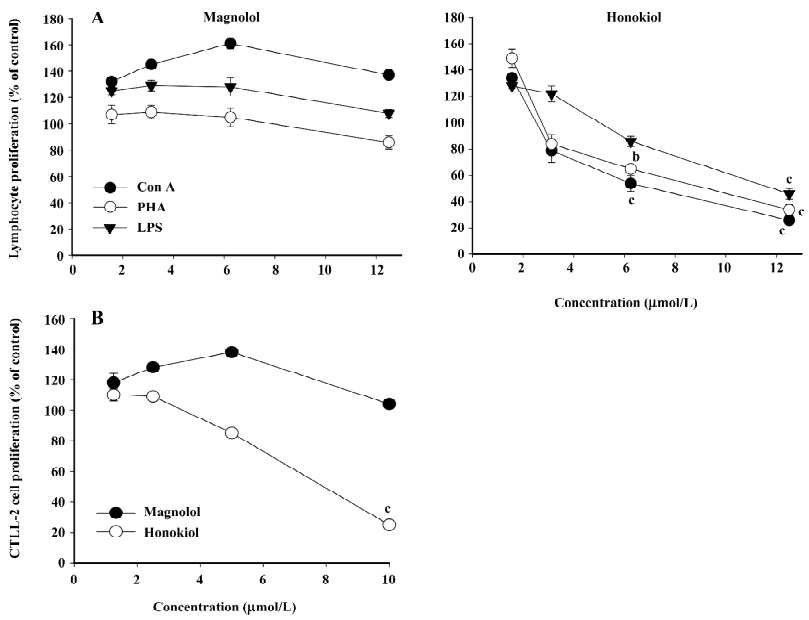
Mechanism of honokiol inhibition in inflammatory responses To understand the molecular mechanism by which honokiol blocked various inflammatory processes performed by macrophages and lymphocytes, the effects of honokiol and signaling enzyme inhibitors (LY294002 and U0126) on LPS-induced signaling and cellular events were first investigated. Since the compound has been reported to suppress a signaling cascade composed of IKK, IκB, and NF-κB, we focused on the upstream signaling events for NF-κB activation. Figure 6A shows that LPS stimulation triggered the activation of several upstream signaling molecules (Src, p85 [a regulatory subunit of PI3K p110], Akt, IκBα, p38, and ERK), as assessed by their phosphorylation levels using phospho-specific antibodies. Interestingly, honokiol only strongly blocked the phosphorylation of Akt and IκBα (at 15 and 30 min) without altering the total level of Akt, but weakly suppressed or not at all the phosphorylation of other proteins. This suggests that the Akt pathway could be regarded as a target event, although it enhanced LPS-induced Akt phosphorylation at 5 min, as shown in the cases of phosphatase inhibitors. Furthermore, since 40 μmol/L honokiol never or weakly blocked the phosphorylation of MAPK (ERK and p38; Figure 6A), it is thought that this compound may selectively modulate the PI3K/Akt pathway.
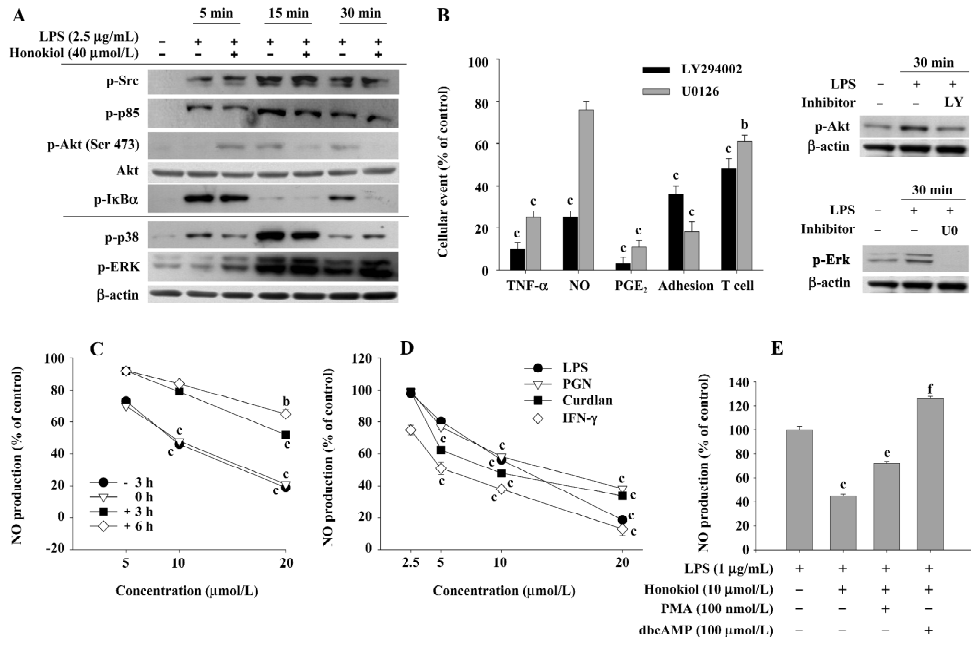
To confirm whether the Akt signal is critical in honokiol-mediated inhibitory effects, specific inhibitors (LY294002 and U0126) blocking the activation of Akt and ERK were employed to evaluate their potency in various inflammatory responses. As depicted in Figure 6B, the PI3K/Akt inhibitor LY294002 strongly suppressed the LPS-induced production of TNF-α, NO, PGE2, CD29-mediated cell–cell adhesion, and Con A-induced T cell proliferation, as shown in the case of honokiol, whereas U0126 only partially blocked NO production and T cell proliferation. Meanwhile, LY294002 and U0126 were shown to suppress the phosphorylation of Akt and ERK triggered by LPS at 30 min, as assessed by measuring the phosphorylation level, indicating that the inhibitors worked very well. To characterize the importance of the Akt pathway as an early signaling event, the time-dependent function of honokiol treatment and NO inhibitory effects under different Akt-inducing stimuli or Akt-regulating signals (such as PKC and PKA) were carefully evaluated. The inhibitory potency of honokiol was dramatically reduced when honokiol treatment was delayed after exposure to LPS (Figure 6C). Honokiol also strongly suppressed NO production induced by LPS, PGN (a ligand of TLR-2), curdlan (β-glucan), and IFN-γ, which are known to induce the phosphorylation of Akt (Figure 6D). Finally, the Akt inhibitory conditions achieved through upregulating PKC (by PMA) or PKA (by dbcAMP) interestingly abrogated honokiol inhibition obtained in the LPS exposure (Figure 6E). Therefore, our data suggest that the Akt pathway may be a critical target of honokiol in its anti-inflammatory actions.
Discussion
In this study, we explored the anti-inflammatory effect of honokiol (Figure 1) and its regulating mechanism using various immune cells and their inflammatory responses under non-cytotoxic conditions (Figure 2). Thus, honokiol (and magnolol) suppressed LPS-mediated cellular responses (Figure 3), such as the production of TNF-α, PGE2, and NO, and upregulated CD80; honokiol (and magnolol) also suppressed CD29-mediated cell–cell and cell-fibronectin adhesions (Figure 4) and mitogen-mediated lymphocyte proliferation (Figure 5) in a dose-dependent manner. The production of inflammatory mediators seems to be due to the suppression of transcriptional activation, since it blocked mRNA levels of the pro-inflammatory proteins (Figure 3E). In agreement with previous papers[29,30], honokiol blocked the production of NO and TNF-α and the proliferation of lymphocytes and CTLL-2 cells more significantly than magnolol (Figures 3, 5). In particular, the inhibitory potency (IC50 values=3–15 µmol/L) of honokiol on NO, PGE2, and TNF-α production is considerable when compared with other natural products or clinically-available drugs. The activities are similar with other sesquiterpene lactone compounds, such as cynaropicrin[21], or higher than those of previously isolated natural compounds, such as ginsenosides, flavonoids and coumarins, and pentoxifylline and prednisolone with IC50 values of 75–200 µmol/L[31–33]. Furthermore, honokiol was previously found to inhibit other inflammatory gene products, such as IL-8, intercellular adhesion molecule (ICAM)-1, and matrix metalloproteinase-9 induced by LPS, TNF-α, and Propionibacterium acnes[16,34]. It has been also reported that honokiol inhibited PMA- or formyl peptide (fMLP)-induced reactive oxygen species production by neutrophils[35]. Therefore, our data suggest that honokiol may be considered a promising drug with potent anti-inflammatory actions in addition to its strong anticancer, anti-angiogenesis, and antineurodegeneration effects.
Post-treatment with honokiol 3 and 6 h after LPS markedly reduced its inhibitory activity (Figure 6C), suggesting that the pharmacological target of honokiol may be activated early. Several research groups have reported that honokiol and magnolol strongly inhibited NF-κB translocation, a critical step found in early inflammatory events and tumor angiogenesis[36–38]. Using an immunoblotting analysis of the phosphorylation level of IκBα, we also learned that the phosphorylation of IκBα at 30 min was diminished by honokiol treatment. Indeed, the Fong group mechanistically proved current results through demonstrating that magnolol and honokiol strongly inhibited IKK activation induced by TNF-α in U937 cells via directly suppressing IKK activity[16,17], although the signal transducer and activator of transcription protein 3[39] and the c-Jun N-terminal kinase were also regarded as pharmacological targets[40]. It was however notable that the LPS induction of the phosphorylation of Akt, an upstream kinase of IKK, was strongly suppressed by honokiol exposure (Figure 6A), suggesting that the real target of honokiol may be not IKK only, but its upstream kinase. Several lines of evidence seem to support this possibility including: (i) honokiol has been reported to block the TNF-α-induced phosphorylation of Akt[41]; (ii) an inhibitor of PI3K/Akt, LY294002, but not U0126, an ERK inhibitor, as well as p38 MAPK inhibitor SB203580 (data not shown), displayed a similar inhibitory spectrum (Figure 6B); (iii) honokiol suppressed all NO production conditions induced by other Akt-activating inducers, such as IFN-γ, PGN, and curdlan (Figure 6D); and (iv) honokiol inhibition was abolished by PKC (by PMA) or PKA (by dbcAMP) treatment (Figure 6E), similar to Akt inhibition[42,43], although we cannot exclude Akt-independent upregulation manners.
The PI3K/Akt pathway is known to play critical roles in various cellular processes, such as apoptosis, inflammatory responses, and tumor angiogenesis[36–38]. Due to its multipotential roles in various disease onsets, it is regarded as a therapeutic target protein in developing anticancer and anti-inflammatory drugs[44]. The activation of the PI3K/Akt pathway requires a series of upstream signaling cascades composed of non-receptor-type protein tyrosine kinase, such as Src, serine/threonine kinases, such as 3'-phosphoinositide-dependent protein kinase-1 (PDK1) and S473 kinase, and p85[45,46]. Thus, the Src-induced phosphorylation of p85 allows the activation of p110, a catalytic subunit of PI3K, which participates in producing phosphatidylinositol-3,4,5-triphosphate (PIP3)[47]. Then, through binding to PIP3, PDK1 is consequently able to phosphorylate threonine-308/serine-473 of Akt[47]. Whether honokiol is able to directly diminish the kinase activity of Akt is not clear yet. However, no inhibition of Src and p85 phosphorylation (Figure 6A) seems to suggest that the target of honokiol may be p110 with kinase activity, PDK1, or even Akt itself. Since exploring the direct target of honokiol will greatly improve our understanding of its pharmacological actions, the exact molecular studies on this point will be carefully carried out in the next experiments using direct kinase assays.
Pharmacokinetic studies with honokiol revealed that this compound is effective in vivo. Thus, single treatment with a 250 mg/kg injection (ip) exhibited a maximum plasma concentration of more than 3.5 mmol/L with an elimination half-life of 5.2 h[48]. Multiple intraperitoneal treatments with 3 mg/mouse at 1–2 d intervals was promisingly curative of tumor generation and growth in nude mice[49]. These results indicate that various in vitro effects on inflammation, apoptosis, and neuroprotection can be achievable under in vivo conditions, and therefore honokiol seems to be applicable as an anti-inflammatory and anticancer drug.
In conclusion, honokiol strongly inhibited various inflammatory responses, such as the LPS-induced upregulation of inflammatory mediators, inflammatory genes, and CD80; the functional activation of β1-integrin (CD29); and the mitogenic proliferation of lymphocytes and CD8+CTLL-2 cells. According to pharmacological, biochemical, and functional analyses, honokiol was found to interrupt the early activated intracellular signaling molecules PI3K/Akt, but not Src, ERK, and p38. Therefore, our data suggest that honokiol may act as a potent anti-inflammatory agent to be developed with PI3K/Akt inhibitory activity.
Acknowledgement
We acknowledge The Central Laboratory of Kangwon National University for the flow cytometric analysis.
References
- Stafford JL, Neumann NF, Belosevic M. Macrophage-mediated innate host defense against protozoan parasites. Crit Rev Microbiol 2002;28:187-248.
- Newman SL. Macrophages in host defense against Histoplasma capsulatum. Trends Microbiol 1999;7:67-71.
- Shiloh MU, Nathan CF. Reactive nitrogen intermediates and the pathogenesis of Salmonella and mycobacteria. Curr Opin Microbiol 2000;3:35-42.
- Cherayil BJ, Antos D. Inducible nitric oxide synthase and salmonella infection. Microbes Infect 2001;3:771-6.
- Fujiwara N, Kobayashi K. Macrophages in inflammation. Curr Drug Targets Inflamm Allergy 2005;4:281-6.
- Appel S, Mirakaj V, Bringmann A, Weck MM, Grunebach F, Brossart P. PPAR-gamma agonists inhibit toll-like receptor-mediated activation of dendritic cells via the MAP kinase and NF-kappaB pathways. Blood 2005;106:3888-94.
- Ojaniemi M, Glumoff V, Harju K, Liljeroos M, Vuori K, Hallman M. Phosphatidylinositol 3-kinase is involved in Toll-like receptor 4-mediated cytokine expression in mouse macrophages. Eur J Immunol 2003;33:597-605.
- Wang X, Wang Y, Geng Y, Li F, Zheng C. Isolation and purification of honokiol and magnolol from cortex Magnoliae officinalis by high-speed counter-current chromatography. J Chromatogr A 2004;1036:171-5.
- Ou HC, Chou FP, Sheu WH, Hsu SL, Lee WJ. Protective effects of magnolol against oxidized LDL-induced apoptosis in endothelial cells. Arch Toxicol 2007;81:421-32.
- Liou KT, Shen YC, Chen CF, Tsao CM, Tsai SK. Honokiol protects rat brain from focal cerebral ischemia-reperfusion injury by inhibiting neutrophil infiltration and reactive oxygen species production. Brain Res 2003;992:159-66.
- Bang KH, Kim YK, Min BS, Na MK, Rhee YH, Lee JP, et al. Antifungal activity of magnolol and honokiol. Arch Pharm Res 2000;23:46-9.
- Kong ZL, Tzeng SC, Liu YC. Cytotoxic neolignans: an SAR study. Bioorg Med Chem Lett 2005;15:163-6.
- Lin YR, Chen HH, Ko CH, Chan MH. Neuroprotective activity of honokiol and magnolol in cerebellar granule cell damage. Eur J Pharmacol 2006;537:64-9.
- Lee B, Kim CH, Moon SK. Honokiol causes the p21WAF1-mediated G(1)-phase arrest of the cell cycle through inducing p38 mitogen activated protein kinase in vascular smooth muscle cells. FEBS Lett 2006;580:5177-84.
- Ishitsuka K, Hideshima T, Hamasaki M, Raje N, Kumar S, Hideshima H, et al. Honokiol overcomes conventional drug resistance in human multiple myeloma by induction of caspase-dependent and -independent apoptosis. Blood 2005;106:1794-800.
- Tse AK, Wan CK, Zhu GY, Shen XL, Cheung HY, Yang M, et al. Magnolol suppresses NF-kappaB activation and NF-kappaB regulated gene expression through inhibition of IkappaB kinase activation. Mol Immunol 2007;44:2647-58.
- Tse AK, Wan CK, Shen XL, Yang M, Fong WF. Honokiol inhibits TNF-alpha-stimulated NF-kappaB activation and NF-kappaB-regulated gene expression through suppression of IKK activation. Biochem Pharmacol 2005;70:1443-57.
- Lee J, Jung E, Park J, Jung K, Lee S, Hong S, et al. Anti-inflammatory effects of magnolol and honokiol are mediated through inhibition of the downstream pathway of MEKK-1 in NF-kappaB activation signaling. Planta Med 2005;71:338-43.
- Munroe ME, Arbiser JL, Bishop GA. Honokiol, a natural plant product, inhibits inflammatory signals and alleviates inflammatory arthritis. J Immunol 2007;179:753-63.
- Cho JY, Chain BM, Vives J, Horejsi V, Katz DR. Regulation of CD43-induced U937 homotypic aggregation. Exp Cell Res 2003;290:155-67.
- Cho JY, Baik KU, Jung JH, Park MH. In vitro anti-inflammatory effects of cynaropicrin, a sesquiterpene lactone, from Saussurea lappa. Eur J Pharmacol 2000;398:399-407.
- Cho JY, Fox DA, Horejsi V, Sagawa K, Skubitz KM, Katz DR, et al. The functional interactions between CD98, beta1-integrins, and CD147 in the induction of U937 homotypic aggregation. Blood 2001;98:374-82.
- Cho JY, Skubitz KM, Katz DR, Chain BM. CD98-dependent homotypic aggregation is associated with translocation of protein kinase Cdelta and activation of mitogen-activated protein kinases. Exp Cell Res 2003;286:1-11.
- Larrucea S, Gonzalez-Rubio C, Cambronero R, Ballou B, Bonay P, Lopez-Granados E, et al. Cellular adhesion mediated by factor J, a complement inhibitor. Evidence for nucleolin involvement. J Biol Chem 1998;273:31718-25.
- Bradford MM. A rapid and sensitive method for the quantitation of microgram quantities of protein utilizing the principle of protein-dye binding. Anal Biochem 1976;72:248-54.
- Raso GM, Pacilio M, Di Carlo G, Esposito E, Pinto L, Meli R. In-vivo and in-vitro anti-inflammatory effect of Echinacea purpurea and Hypericum perforatum. J Pharm Pharmacol 2002;54:1379-83.
- Cieslik K, Zhu Y, Wu KK. Salicylate suppresses macrophage nitric-oxide synthase-2 and cyclo-oxygenase-2 expression by inhibiting CCAAT/enhancer-binding protein-beta binding via a common signaling pathway. J Biol Chem 2002;277:49304-10.
- Berg J, Fellier H, Christoph T, Grarup J, Stimmeder D. The analgesic NSAID lornoxicam inhibits cyclooxygenase (COX)-1/-2, inducible nitric oxide synthase (iNOS), and the formation of interleukin (IL)-6 in vitro. Inflamm Res 1999;48:369-79.
- Son HJ, Lee HJ, Yun-Choi HS, Ryu JH. Inhibitors of nitric oxide synthesis and TNF-alpha expression from Magnolia obovata in activated macrophages. Planta Med 2000;66:469-71.
- Matsuda H, Kageura T, Oda M, Morikawa T, Sakamoto Y, Yoshikawa M. Effects of constituents from the bark of Magnolia obovata on nitric oxide production in lipopolysaccharide-activated macrophages. Chem Pharm Bull (Tokyo) 2001;49:716-20.
- Cho JY, Park J, Kim PS, Yoo ES, Baik KU, Park MH. Savinin, a lignan from Pterocarpus santalinus inhibits tumor necrosis factor-alpha production and T cell proliferation. Biol Pharm Bull 2001;24:167-71.
- Cho JY, Baik KU, Yoo ES, Yoshikawa K, Park MH. In vitro antiinflammatory effects of neolignan woorenosides from the rhizomes of Coptis japonica. J Nat Prod 2000;63:1205-9.
- Cho JY, Yoo ES, Baik KU, Park MH, Han BH. In vitro inhibitory effect of protopanaxadiol ginsenosides on tumor necrosis factor (TNF)-alpha production and its modulation by known TNF-alpha antagonists. Planta Med 2001;67:213-8.
- Park J, Lee J, Jung E, Park Y, Kim K, Park B, et al. In vitro antibacterial and anti-inflammatory effects of honokiol and magnolol against Propionibacterium sp. Eur J Pharmacol 2004;496:189-95.
- Liou KT, Shen YC, Chen CF, Tsao CM, Tsai SK. The anti-inflammatory effect of honokiol on neutrophils: mechanisms in the inhibition of reactive oxygen species production. Eur J Pharmacol 2003;475:19-27.
- Maulik N, Das DK. Redox signaling in vascular angiogenesis. Free Radic Biol Med 2002;33:1047-60.
- Bharti AC, Aggarwal BB. Nuclear factor-kappa B and cancer: its role in prevention and therapy. Biochem Pharmacol 2002;64:883-8.
- Bochkov VN, Leitinger N. Anti-inflammatory properties of lipid oxidation products. J Mol Med 2003;81:613-26.
- Aggarwal BB, Sethi G, Ahn KS, Sandur SK, Pandey MK, Kunnumakkara AB, et al. Targeting signal-transducer-and-activator-of-transcription-3 for prevention and therapy of cancer: modern target but ancient solution. Ann N Y Acad Sci 2006;1091:151-69.
- Park EJ, Kim SY, Zhao YZ, Sohn DH. Honokiol reduces oxidative stress, c-jun-NH2-terminal kinase phosphorylation and protects against glycochenodeoxycholic acid-induced apoptosis in primary cultured rat hepatocytes. Planta Med 2006;72:661-4.
- Ahn KS, Sethi G, Shishodia S, Sung B, Arbiser JL, Aggarwal BB. Honokiol potentiates apoptosis, suppresses osteoclastogenesis, and inhibits invasion through modulation of nuclear factor-kappaB activation pathway. Mol Cancer Res 2006;4:621-33.
- Li L, Sampat K, Hu N, Zakari J, Yuspa SH. Protein kinase C negatively regulates Akt activity and modifies UVC-induced apoptosis in mouse keratinocytes. J Biol Chem 2006;281:3237-43.
- Lou L, Urbani J, Ribeiro-Neto F, Altschuler DL. cAMP inhibition of Akt is mediated by activated and phosphorylated Rap1b. J Biol Chem 2002;277:32799-806.
- Yamamoto Y, Gaynor RB. Role of the NF-kappaB pathway in the pathogenesis of human disease states. Curr Mol Med 2001;1:287-96.
- Yamamoto Y, Gaynor RB. IkappaB kinases: key regulators of the NF-kappaB pathway. Trends Biochem Sci 2004;29:72-9.
- Moissoglu K, Sachdev S, Gelman IH. Enhanced v-Src-induced oncogenic transformation in the absence of focal adhesion kinase is mediated by phosphatidylinositol 3-kinase. Biochem Biophys Res Commun 2005;330:673-84.
- Caron RW, Yacoub A, Li M, Zhu X, Mitchell C, Hong Y, et al. Activated forms of H-RAS and K-RAS differentially regulate membrane association of PI3K, PDK-1, and AKT and the effect of therapeutic kinase inhibitors on cell survival. Mol Cancer Ther 2005;4:257-70.
- Chen SZ, Jia H, Wu YH, Wang H. Pharmacokinetics of honokiol in rats. Beijing Da Xue Xue Bao 2004;36:41-4. Chinese..
- Bai X, Cerimele F, Ushio-Fukai M, Waqas M, Campbell PM, Govindarajan B, et al. Honokiol, a small molecular weight natural product, inhibits angiogenesis in vitro and tumor growth in vivo. J Biol Chem 2003;278:35501-7.