Promotion of cell proliferation by HBXIP via upregulation of human telomerase reverse transcriptase in human mesenchymal stem cells1
Introduction
The hepatitis B virus X-interacting protein (HBXIP), originally cloned by Melegari et al from HepG2 cells[1], is specifically associated with the C-terminal of the X protein of the hepatitis B virus (HBX) and negatively regulates the activity of HBX. The hbxip gene encodes a 9.6 kDa protein with 91 amino acids[1]. As a constitutive expressive protein, the basic physiological function of HBXIP remains unclear. Previous studies have indicated that the overexpression of HBXIP suppresses hepatitis B virus replication in HepG2 cells in addition to suppressing the transactivation phenotype of HBX[1]. Furthermore, HBXIP forms complexes with survivin, an anti-apoptotic protein that is overexpressed in most human cancers, to selectively suppress apoptosis initiated via the mitochondria/cytochrome c pathway[2]. HBXIP also binds with hSuv3p, a human ATP-dependent RNA/DNA helicase, and the parts of HBXIP binding is just the mitochondrial import domain of hSuv3p[3]. HBXIP is also a regulator of centrosome duplication, required for bipolar spindle formation in HeLa human carcinoma cells and primary mouse embryonic fibroblast cells. Most cells deficient in HBXIP arrest in prometaphase with monopolar spindles, and the overexpression HBXIP causes tripolar or multipolar spindles due to excessive centrosome replication[4]. We previously found that HBXIP inhibited the apoptosis induced by HBX in H7402 cells[5], and promoted the cell proliferation both in cancer cells and normal cells by upregulating the expression of cyclin D1 and cyclin E and downregulating the expression of p21 and p27[6,7]. Our previous studies demonstrated that HBXIP promotes cell proliferation by regulating the transcriptional activity of NF-κB[8]. However, the mechanism of promoting cell proliferation meditated by HBXIP remains unclear. HBXIP can bridge HBX and survivin together to suppress the caspase activation in cancer cells. Because both HBX and survivin are able to upregulate the activity of human telomerase reverse transcriptase (hTERT)[9,10]. In the present study, we determine whether HBXIP promotes cell proliferation via regulating the activity of hTERT.
Telomeres are repetitive sequences at the ends of chromosomes that protect chromosomes from incomplete replication, nuclease degradation, and end-to-end fusion during replication[11]. Synthesis and maintenance of telomeres’ repeats are mediated by a specialized enzyme, known as telomerase[12]. Telomerase is a ribonucleic protein DNA polymerase that elongates the telomeres of chromosomes to compensate for losses that occur with each round of DNA replication. The human telomerase consists of 2 essential components: the human telomerase RNA component and the catalytic component, known as hTERT. Several other protein subunits associate with these core components to form the telomerase holoenzyme[13]. Among the multiple components of human telomerase, only the catalytic subunit hTERT seems to be the key component that determines telomerase activity. Although hTERT is expressed and telomerase is active during embryo development (telomerase positive), TERT is downregulated, and the expression of hTERT is repressed in mature somatic cells (telomerase negative). The other components of telomerase holoenzyme are usually expressed ubiquitously in both telomerase-positive and -negative cells[13,14]. Telomerase activity is a common feature of tumor tissue and cell lines, and telomerase activation has been suggested to be a late event in tumorigenesis[15]. The transcriptional activation of the hTERT gene is a critical rate-limiting step in hTERT function and telomerase activity. The hTERT gene has multiple sites for transcriptional regulation. Transcription factors, such as c-Myc, Sp1, NF-κB, and upstream stimulatory factors have been shown to upregulate hTERT transcription[16–18]. In addition, a previous report indicated that the overexpression of Bcl-2, which is involved in the inhibition of cellular apoptosis, also greatly increases telomerase activity[19].
Mesenchymal stem cells (MSC) are a cell type with differentiation capacity along mesodermal lineages, and fortuitously, are easily isolated and propagated in vitro. MSC are non-hematopoietic stromal cells that are capable of differentiating into––and contribute to the regeneration of––mesenchymal tissues. MSC appear to constitute a heterogeneous population of undifferentiated and committed lineage-primed cells, capable of homing upon engraftment to a number of growth microenvironments, extensive proliferation, producing large numbers of differentiated progeny, and functional tissue repair after injury. MSC are identified by the expression of many molecules, including CD105 (SH2) and CD73 (SH3/4), and are negative for the hematopoietic markers CD34, CD45, and CD14. In addition, MSC are extensively distributed throughout tissues, and bone marrow MSC provide the stromal component of the niche of hematopoietic stem cells. Because they present several features setting them apart from other stem cells, MSC may constitute another paradigm for stem cell systems, where self-renewal and hierarchy are no longer essential, but where plasticity is the major characteristic. The properties of MSC make these cells potentially ideal candidates for tissue engineering[20] and antitumor therapy[21].
In the present study, we first investigated the effect of HBXIP on hTERT. Our data showed that HBXIP was able to regulate hTERT at 3 levels, including promoting the transcriptional activity of hTERT, telomerase activity, and the expression of hTERT in BMMS-03 cells, suggesting that HBXIP promotes the cell proliferation via the upregulation of hTERT.
Materials and methods
Cell culture BMMS-03 human MSC (hMSC) with CD105+, CD106+, and CD34–[22,23], derived from the bone marrow of a 4-month-old elicited fetus, were used in this study. All sampling methods and protocols used in the study conformed to the relevant ethical guidelines, and informed consent for tissue collection was obtained from patient before elicitation. BMMS-03 cells were maintained with DMEM medium, supplemented with inactivated 10% fetal calf serum, 100 U/mL penicillin, and 100 µg/mL streptomycin in a humidified atmosphere of 37 ºC with 5% CO2.
Construction of HBXIP gene in vectors The construction of pcDNA3-hbxip has been described[5]. In brief, total RNA was extracted from H7402 cells using Trizol (Invitrogen, Carlsbad, CA, USA) reagent following the manufacturer’s recommendations. Prior to the first cDNA strand synthesis, total RNA was digested with RNase-free DNase I (TaKaRa, Tokyo, Japan) at 37 ºC for 20 min and inactivated at 60 ºC for 10 min. The first cDNA strand was synthesized in a 20 µL reaction system with M-MLV reverse transcriptase (TaKaRa, Japan). In total, 1 µL cDNA template was used in a 50 µL reaction volume with rTag DNA polymerase (TaKaRa, Japan) and the oligonucleotide primers were as follows: 5'-GACGAATTCATGGAGGCGACCTTGGAGCA-3'(forward) and 5'-GATCTCGAGTCAAGAGGCCATTTTGTGCA-3´ (reverse). The resultant cDNA fragments were inserted into a pcDNA3 vector (termed pcDNA3-hbxip).
Construction of HBXIP-specific siRNA in the plasmid vector According to the report[1], we cloned small hairpin RNA targeting HBXIP mRNA containing the sense target sequence (5'-GCAGCTAAGCTAACCTCTG-3') followed by a 9 nucleotide hairpin loop complementary target sequence in a pSilencer 3.0-H1 plasmid (Ambion, Austin, USA) under the control of the H1 promoter. The resulting construct pSilencer–hbxip was used to transfect mammalian cells. After PCR amplication and enzyme digestion, identification of the RNA interference (RNAi) fragment of HBXIP inserted in the vector was performed by sequencing.
Assay of hTERT promoter activity The pBT-SE plasmid containing the luciferase reporter of the hTERT promoter was kindly provided by Prof Li-xin WEI, (Second Military Medical University, Shanghai, China). A 1.7 kb DNA fragment encompassing the hTERT gene promoter was elongated to the pGL3–Basic vector (Promega, Madison, WI, USA), allowing transcription of the firefly luciferase gene under the control of this fragment. For the luciferase assay, the cells were seeded onto 6-well tissue culture plates, cultured overnight, and transiently cotransfected with plasmids, such as 0.5 μg pBT–SE hTERT promoter reporter; 0.5 µg pRL-TK (Promega, USA), containing the Renilla reniformis luciferase gene as the inner control; 1 or 1.5 µg pcDNA3–hbxip, using Lipofectamine 2000 (Invitrogen, USA); and 2 µg pcDNA3 and 2 µg pSilencer-hbxip were used as the control cotransfected with pBT-SE and pRL-TK, respectively. The level of firefly luciferase activity was normalized to that of Renilla reniformis luciferase activity for each transfection. The cells were lysed after 40 h transfection, and luciferase activity was determined using the dual luciferase reporter assay system (Promega, USA), following the manufacturer’s specifications. Luciferase and renilla luciferase luminescence were measured by using a luminometer. All of the data shown in this study were obtained from at least 3 independent experiments.
Transfection One day before transfection, the BMMS-03 cells were collected and seeded into 6-well plates at 1×105 cells per well (n=3, each group). The cells were transfected with plasmids, such as 2 µg pcDNA3 empty vector, 1 µg or 1.5µg pcDNA3-hbxip, and 2µg pSilencer–hbxip by using Lipofectamine 2000, respectively, according to the manufacturer’s instruction. The transfection mixture was removed after 6 h transfection and completed medium with 10% fetal calf serum was added. The transfection efficiency in the cells was monitored by the cotransfection of 0.1 µg pEGFP-C2 plasmid, which expresses the green fluorescence protein.
Telomeric repeat amplification protocol assay The telomeric repeat amplification protocol (TRAP) assay was performed by using the TRAPeze telomerase detection kit according to manufacturer’s instructions (InterGen, Burlington, USA). The cells transiently transfected or the untransfected controls were pelleted, washed twice with ice-cold phosphate buffered saline (PBS), and then repelleted. Each cell pellet was then resuspended in the 3-cholamidopropyl dimethylammonio-1-propanesulfonate lysis buffer supplied in the kit. Extension was performed for 30 min at 30 °C, and the Taq polymerase was then added to each sample and PCR amplification was performed as follows: 33 cycles at 94 °C for 30 s; 59 °C for 30 s, and 72 °C for 1 min. Products were separated on 12% SDS-PAGE and visualized by means of sliver staining. Each sample was assayed for telomerase activity in duplicate, starting from the protein extracts of the cell line. Telomerase activity was calculated from the ratio of the intensity of the telomeric repeat ladder by applying Glyco Band Scan software (PROZYME, San Leandro, CA, USA), starting at 50 bp, to that of the 36 bp inner reference.
Western blot analysis After transfection, the cells were washed twice in cold PBS (pH 7.4). Total protein extracts were prepared by lysis buffer, including 50 mmol/L Tris-HCl (pH 7.5), 150 mmol/L NaCl, 10 mmol/L EDTA 1% Nonidet P 40, 0.1% SDS, 1 mmol/L phenylmethylsulfonyl fluoride and 1 µg/mL each leupeptin, aprotinin, and pepstatin. Insoluble proteins were discarded by high-speed centrifugation at 4 °C. The protein concentration in the supernatant was determined by using a commercially available assay (Bio-Rad, Hercules, USA). Equal amounts of lysates were separated with the use of SDS-PAGE and electrophoretically transferred onto a polyvinylidene difluoride membrane (Millipore, Bedford, MA, USA). The membrane was blocked with 5% non-fat dry milk in PBS containing 0.1% Tween-20 (PBST) and incubated overnight at 4 °C with the following specific primary antibodies: mouse anti-hTERT (Santa Cruz Biotechnology, Santa Cruz, CA, USA; 1: 800 dilution), mouse anti-c-Myc (Santa Cruz Biotechnology; 1:500 dilution), mouse anti-Bcl-2 (NeoMarkers, Fremont, CA, USA; 1:500 dilution), rabbit anti-HBXIP[6] (1:1000 dilution), and β-actin (Sigma, St Louis, MO, USA; 1:20000 dilution). Subsequently, the membrane was washed with PBST buffer and incubated with the appropriate secondary antibody and developed with the use of an enhanced chemiluminescence system (Amersham Pharmacia Biotech, Buckinghamshire, UK). All experiments were repeated 3 times.
Statistical analysis Comparisons between means was tested by Student’s t-test and P-values less than 0.05 were considered statistically significant. The data are expressed as mean±SEM.
Results
Promotion of hTERT promoter by HBXIP Because the catalytic subunit of telomerase is a primary target for activity and its gene expression is mainly regulated at the transcriptional level, we measured the hTERT promoter activities in BMMS-03 cells using the pBT–SE plasmid by dual luciferase reporter assay. The HBXIP expression vector pcDNA3–hbxip or the HBXIP siRNA vector pSilencer–hbxip was cotransfected along with the pBT–SE plasmid into BMMS-03 cells. The results showed that the activity of luciferase was significantly increased in a dose-dependent manner compared to the control when HBXIP was overexpressed in the BMMS-03 cells, whereas RNAi targeting HBXIP mRNA led to a decrease in the activity of the hTERT promoter (Figure 1).
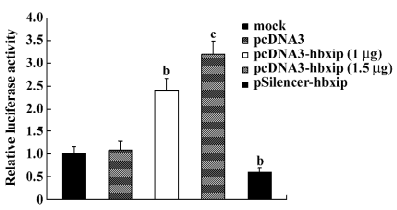
Enhancement of telomerase activity by HBXIP In order to determine if HBXIP could mediate the endogenous telomerase enzymatic activity, the TRAP assay was used to measure the telomerase activity in BMMS-03 cells. The results showed that the overexpression of HBXIP was able to increase the telomerase activity by TRAP assay (Figure 2), whereas, RNAi targeting HBXIP mRNA resulted in the decrease of the hTERT activity.
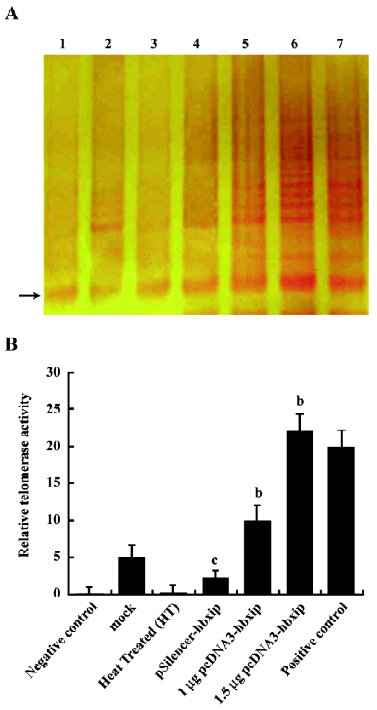
Upregulation of the expression of hTERT, c-Myc, and Bcl-2 by HBXIP Transfection efficiency revealed that approximately 70%–80% of cells showed green fluorescence (Figure 3). The above data showed that HBXIP was able to upregulate the transcription and activity of hTERT. Next, we investigated the effect of HBXIP on the expression of the hTERT protein in BMMS-03 cells. After transfection, we found that the overexpression of HBXIP upregulated the expression of the hTERT protein by Western blot analysis; however, the hTERT protein was undetectable in BMMS-03 cells in the control and treatment with RNAi targeting HBXIP mRNA (Figure 4). In addition, the expression of c-Myc, a transcription factor involved in the control of the hTERT promoter, was examined by Western blot analysis in the BMMS-03 cells. Bcl-2, an anti-apoptosis protein, was also examined. RNAi targeting HBXIP mRNA had opposite results (Figure 4), which is consistent with our previous results by using MCF-7 or H7402 cells[6]. It was confirmed that HBXIP was overexpressed by transfection with the pcDNA3-hbxip plasmid, and HBXIP was downregulated by transfection with the pSilencer-hbxip plasmid (Figure 4). We further confirmed this finding by applying Glyco Band-Scan software (Figure 4).
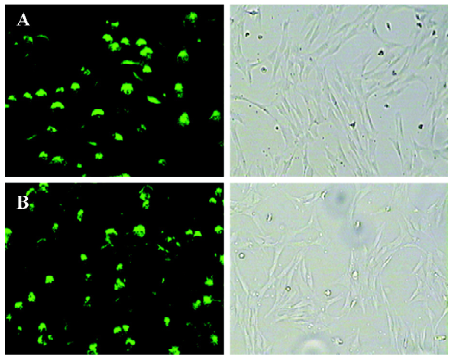
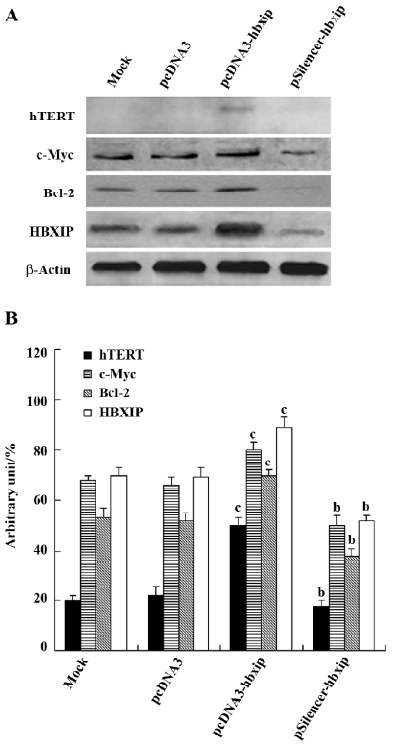
Discussion
HBXIP, originally cloned from HepG2 cells, specifically interacts with the C-terminal of HBX so that it negatively regulates the activity of HBX. Although some binding proteins of HBXIP have been identified in the last few years, as a protein of constitutive expression, the basic physiological functions of HBXIP are still unclear. Because NF-κB is an upregulator of the hTERT promoter[14], we previously found that HBXIP promotes cell proliferation via regulating the transcriptional activity of NF-κB[8], so we suppose that hTERT involves the promotion of cell proliferation by HBXIP. Therefore, in this study, we investigated the effect of HBXIP on telomerase activity in hMSC.
Uncontrolled proliferation is a hallmark of cancer cells. The maintenance of normal cell function and tissue homeostasis is dependent on the precise regulation of multiple signaling pathways that control cellular decisions to either proliferate, differentiate, arrest cell growth, or apoptosis. Studies of both oncogenic processes as well as normal cell growth control have revealed regulating the cell cycle to control the cell proliferation. A number of other cell regulatory activities, including NF-κB and hTERT, have also been shown to control the cell proliferation[24,25].
Telomerase is a key enzyme that catalyzes the synthesis of telomere DNA participating in cell immortalization through the stabilization of the chromosomal structure. It is mostly expressed in human tumors and is low and difficult to detect in somatic cells generally[26,27], so it may be a useful molecular marker for cancer diagnosis and therapeutic strategies. The hTERT mRNA expression temporally parallels changes in telomerase activity during cellular differentiation and neoplastic transformation. The overexpression of hTERT in cancer cells is thought to contribute to tumor development and angiogenesis. Telomere shortening is a well-known mechanism for cell growth control. In order to continue indefinite divisions of all immortal cells, single cell eukaryotes, for instance L-O2, must maintain their telomeres through telomerase expression. In this experiment, we first examined the effect of HBXIP on telomerase activity in hepatoma H7402 cells and normal liver L-O2 cells, but no significant changes was observed between HBXIP-transfected cells and the controls, which may due to the higher background of telomerase activity in tumor cells and normal liver L-O2 cells transfected with the hTERT gene. Although not immortal, MSC have the ability to expand many-fold in culture, while retaining their growth and multilineage potential. Then we tried to test the effect of HBXIP on human bone marrow MSC, BMMS-03, because MSC have higher telomerase activity than normal cells and lower telomerase activity than tumor cells. Our results showed that the overexpression of HBXIP could upregulate telomerase at 3 levels, including transcription (Figure 1), activity (Figure 2), and the expression of hTERT (Figure 4), whereas RNAi targeting HBXIP mRNA resulted in the opposite effect. Although various steps at the transcriptional and post-translational levels have been found to modulate telomerase activity, the transcriptional control of the hTERT gene is the major contributor to the regulation of telomerase activity in most cell types.
Many studies have indicated that telomerase activity in telomerase-competent cells is linked to cellular proliferation[25,28–30]. In contrast to the telomerase-competent cells described, telomerase incompetent cells are always telomerase negative. For example, rapidly growing primary human fibroblasts are telomerase negative, and continuous telomere erosion limits the number of population doublings. This limited proliferation capacity could be overcome by enforced expression of the catalytic component hTERT in transformed fibroblasts[31]. RNAi targeting hTERT mRNA could inhibit the proliferation of hepatocarcinoma cells specifically via the suppression of telomerase activity, hTERT, and c-Myc expression[32]. hTERT gene expression is the first step in telomerase activation for continuous stem cell renewal and proliferation. hTERT expression is often found to be upregulated when cells are proliferating and downregulated during cell differentiation. Many inducible transcription factors have been identified binding the hTERT promoters, such as c-Myc and NF-κB, to regulate the hTERT promoter[14]. Regulation by these transcription factors suggests that the induction of hTERT expression plays an important physiological role. In our data, the Western blot assay indicated that the overexpression of HBXIP was able to upregulate the expression of c-Myc and Bcl-2 in the BMMS-03 cells, which may contribute to the upregulation of hTERT activity[16,19]. c-Myc forms dimers with Max that bind to the 5'-CACGTG-3' sequence (E-box) in promoters and transactivate target genes. The Bcl-2 oncoprotein, as an inhibitor of cellular apoptosis, has also been reported to modulate telomerase[19,32]. The mechanisms involved in the regulation of telomerase activity are of special interest since the modulation of telomerase activity can be used to alter cellular life span and thus can be exploited in anticancer and anti-ageing therapies.
In conclusion, our findings demonstrate that HBXIP stimulates the transcription of hTERT and telomerase activity, as well as upregulates the expression of hTERT, c-Myc, and Bcl-2 in BMMS-03 cells. Therefore, HBXIP promotes cell proliferation via the upregulation of NF-κB[20] and hTERT.
Acknowledgements
We thank Prof Li-xin WEI of Second Military Medical University, Shanghai, China, for providing the plasmid of pBT-SE.
References
- Melegari M, Scaglioni PP, Wands JR. Cloning and characterization of a novel hepatitis B virus x binding protein that inhibits viral replication. J Virol 1998;72:1737-43.
- Marusawa H, Matsuzawa S, Welsh K, Zou H, Armstrong R, Tamm I, et al. HBXIP functions as a cofactor of survivin in apoptosis suppression. EMBO J 2003;22:2729-40.
- Minczuk M, Mroczek S, Pawlak SD, Stepien PP. Human ATP-dependent RNA/DNA helicase hSuv3p interacts with the cofactor of survivin HBXIP. FEBS J 2005;272:5008-19.
- Fujii R, Zhu C, Wen Y, Marusawa H, Bailly-Maitre B, Matsuzawa S, et al. HBXIP, cellular target of hepatitis B virus oncoprotein, is a regulator of centrosome dynamics and cytokinesis. Cancer Res 2006;66:9099-107.
- Zhang X, Ma H, Ye L, Dong N, Shi Z, Sha L, et al. Effect of hepatitis B X interacting protein on apoptosis induced by hepatitis B virus X protein. Chin J Biochem Mol Biol 2005;21:403-7.
- Wang F, Sha L, Zhang W, Wu L, Qiao L, Li N, et al. Involvement of hepatitis B X-interacting protein (HBXIP) in proliferation regulation of cells. Acta Pharmacol Sin 2007;28:431-8.
- Wang F, Wu L, Qiao L, Ye L, Zhang X. Promotion of cell proliferation via regulating cell cycle induced by HBXIP. Chin J Biochem Mol Biol 2007;23:487-91. Chinese..
- Wang F, Sha L, Qiao L, Wu L, Zhang X, Ye L. Promotion of transcriptional activity of NF-κB mediated by HBXIP in hepatoma cells. Prog Biochem Biophys 2007;34:1197-201. Chinese..
- Zhang X, Dong N, Zhang H, You J, Wang H, Ye L. Effects of hepatitis B virus X protein on human telomerase reverse transcriptase expression and activity in hepatoma cells. J Lab Clin Med 2005;145:98-104.
- Endoh T, Tsuji N, Asanuma K, Yagihashi A, Watanabe N. Survivin enhances telomerase activity via up-regulation of specificity protein 1- and c-Myc-mediated human telomerase reverse transcriptase gene transcription. Exp Cell Res 2005;305:300-11.
- Cassandra DB, Thomas RY, Patricia ML, Catherine AR. Telomerase activity: a biomarker of cell proliferation, not malignant transformation. Proc Natl Acad Sci USA 1997;94:13677-82.
- Greider CW. Telomere length regulation. Annu Rev Biochem 1996;65:337-65.
- Cong Y, Wright WE, Shay JW. Human telomerase and its regulation. Microbiol Mol Biol Rev 2002;66:407-25.
- Sikand K, Kaul D, Vaima N. Receptor Ck-dependent signaling regulates hTERT gene transcription. BMC Cell Biol 2006;7:2.
- Blasco MA, Rizen M, Greider CW, Hanahan D. Differential regulation of telomerase activity and telomerase RNA during multi-stage tumorigenesis. NatGenet 1996;12:200-4.
- Janknecht R. On the road to immortality: hTERT upregulation in cancer cells. FEBS Lett 2004;564:9-13.
- Yin L, Hubbard AK, Giardina C. NF-κB regulates transcription of the mouse telomerase catalytic subunit. J Biol Chem 2000; 275: 36 671–5.
- Sinha-Datta U, Horikawa I, Michishita E, Datta A, Sigler-Nicot JC, Brown M, et al. Transcriptional activation of hTERT through the NF-κB pathway in HTLV-I-transformed cells. Blood 2004;104:2523-31.
- Mandal M, Kumar R. Bcl-2 modulates telomerase activity. J Biol Chem 1997; 272: 14 183–7.
- Delorme B, Chateauvieux S, Charbord P. The concept of mesenchymal stem cells. Regen Med 2006;1:497-509.
- Tutter AV, Baltus GA, Kadam S. Embryonic stem cells: a great hope for a new era of medicine. Curr Opin Drug Discov Devel 2006;9:169-75.
- Zhang X, Xu Z, Ye L, Dong N, Zhao C, Cai B, et al. Isolation and identification of mesenchymal stem cells derived from human fetal bone marrow. Acta Scientiarum Naturalium Universitatis Nankaiensis 2006;39:107-10. Chinese..
- Autieri MV, Yue TL, Ferstein GZ, Ohlstein E. Antisense oligonucleotides to the p65 subunit of NF-κB inhibit human vascular smooth muscle cell adherence and proliferation and prevent neointima formation in rat carotid arteries. Biochem Biophys Res Commun 1995;213:827-36.
- Klapper W, Singh KK, Heidorn K, Parwaresch R, Krupp G. Regulation of telomerase activity in quiescent immortalized human cells. Biochim Biophys Acta 1998;1442:120-6.
- Broccoli D, Young JW, de Lange T. Telomerase activity in normal and malignant hematopoietic cells. Proc Natl Acad Sci USA 1995;92:9082-86.
- Hiyama E, Hiyama K, Yokoyama T, Shay JW. Immunohistochemical detection of telomerase (hTERT) protein in human cancer tissues and a subset of cells in normal tissues. Neoplasia 2001;3:17-26.
- Zou L, Zhang P, Luo C, Tu Z. Mad1 suppresses bladder cancer cell proliferation by inhibiting human telomerase reverse transcriptase transcription and telomerase activity. Urology 2006;67:1335-40.
- Weng NP, Levine BL, June CH, Hodes RJ. Regulated expression of telomerase activity in human T lymphocyte development and activation. J Exp Med 1996;183:2471-9.
- Ramirez RD, Wright WE, Shay JW, Taylor RS. Telomerase activity concentrates in the mitotically active segments of human hair follicles. J Invest Dermatol 1997;108:113-7.
- Bodnar AG, Ouellette M, Frolkis M, Holt SE, Chiu CP, Morin GB, et al. Extension of life-span by introduction of telomerase into normal human cells. Science 1998;279:349-52.
- Zhang PH, Zou L, Tu ZG. RNAi–hTERT inhibition hepatocellular carcinoma cell proliferation via decreasing telomerase activity. J Surg Res 2006;131:143-9.
- Massard C, Zermati Y, Pauleau AL, Larochette N, Metivier D, Sabatier L, et al. hTERT: a novel endogenous inhibitor of the mitochondrial cell death pathway. Oncogene 2006;25:4505-14.