Modulation of inflammation-related genes of polysaccharides fractionated from mycelia of medicinal basidiomycete Antrodia camphorata1
Introduction
Antrodia camphorata is a medicinal mushroom in Taiwan well-known as a folk medicine for the treatment of intoxication caused by alcohol or drugs, and is also used in the treatment of diarrhea, abdominal pain, hypertension, skin itching, and liver cancer[1]. It specifically parasitizes on the inner cavity of the wood of the endemic species Cinnamomum kanehirai and grows extremely slowly in nature. Therefore, the submerged liquid culture using the parasitic hyphae of A camphorata for the production of mycelia has being become one of the most important methods. The biological functions of A camphorata have been studied in various bioassays. In the research of aqueous extracts from A camphorata mycelia, the suppression of oxidative hemolysis and lipid/protein peroxidation in erythrocytes has been demonstrated[2]. The inhibition ability of its fermented filtrate on H2O2-induced lipid peroxidation and its extracts on the CCl4-induced rat liver damage was also reported[3,4]. The extracts also showed a concentration-dependent inhibition of N-formyl-methionyl-leucyl-phenylalanine or phorbol 12-myristate 13-acetate-induced reactive oxygen species production in peripheral human neutrophils or mononuclear cells[5]. Moreover, the fermented culture broth of A camphorata has been found to have inhibitory capabilities on the production of lipopolysaccharide (LPS)-induced tumor necrosis factor (TNF)-α, interleukin (IL)-1β, inducible nitric oxide synthase (iNOS) and cyclooxygenase (COX)-2 expression[6]. Water extracts of submerged culture of A camphorata have being shown to have antitumor activity[7–10]. Polysaccharides extracted from fruiting bodies or cultured mycelia of A camphorata exhibit antihepatitis B virus activity[11]. As the polysaccharide components of mushroom species have been established as the most promising pharmacologically-active anti-tumor portion[12,13], the partially-purified polysaccharide component from A camphorata mycelia was also found to have antitumor effects on both the in vitro and in vivo model[14]. Fungous LPS from A camphorata was found to inhibit bacterial LPS-induced intercellular adhesion molecule-1 expression and the subsequent monocyte adhesion process in vascular endothelial cells[15]. However, there is no report about the anti-inflammatory activity of the A camphorata polysaccharide fraction.
Cells of the monocytic/macrophage lineage play an important role in response to inflammation and infection, as the main players performing innate immune response and contributors rendering adaptive immune response. Bacterial LPS, a component of the gram-negative bacteria cell wall, activates macrophages to secrete pro-inflammatory cyto-kines, such as IL-6, TNF-α, and secondary mediators such as leukotrienes, prostaglandins (PG) and nitric oxide (NO). The iNOS is responsible for high output formation of NO by macrophages against invading microorganisms[16] or tumor cells[17]. Although large production of NO by iNOS may promote host defensive potency, it also contributes to septic and hemorrhagic shock, rheumatoid arthritis and chronic infections[18]. The COX-2 is an essential enzyme in the production of inflammatory PG, and is inducible in activated macrophages, fibroblasts, and several other cell types. In vivo, the expression of COX-2 is observed in chronic inflammatory conditions such as arthritis[19] and human colon cancer tissue[20]. In vitro, COX-2 expression was induced in response to stimuli such as LPS and growth factors[21,22]. Therefore, the screening of chemopreventive products from natural resources with the functions focused on inhibiting NO and PGE2 production would be an effective and direct method.
The anti-inflammatory effects of the polysaccharide fractions from A camphorata have never been studied. The objective of this study was to investigate the effects of the polysaccharides from the sequential extractions of A camphorata mycelia on LPS-induced inflammation-related gene expression by using mouse macrophages.
Materials and methods
Materials LPS (Escherichia coli 055:B5) and anti-β-actin antibodies were purchased from Sigma-Aldrich (St Louis, MO, USA). SuperSignal West Pico Chemiluminescent Substrate was obtained from Pierce (Rockford, IL, USA). Antibodies against COX-2, Sp1 and TLR4 were purchased from Santa Cruz (Santa Cruz, CA, USA). The antibody against NF-κB p65 was from Abcam (Cambridge, UK). The antibody against iNOS/NOS II was from Upstate (Lake Placid, NY, USA). PRO-PREPTM Protein Extraction Solution was from iNtRON Biotechnology (Kyungki-Do, Korea). Bio-Rad protein assay was purchased from Bio-Rad (Hercules, CA, USA). TRIzol reagent and SuperScript II were from Invitrogen (Carlsbad, CA, USA).
Cell culture RAW 264.7 cells were purchased from Bioresource Collection and Research Center (Hsinchu, Taiwan) and cultured at 37 ºC in a 5% CO2 atmosphere in Dulbecco’s modified Eagle’s medium (DMEM; GIBCO, Carlsbad, CA, USA) supplemented with 10% fetal bovine serum FBS (Biowest, Rue de la Caille, Nuaille, France). The endotoxin concentration of FBS was below 0.15 ng/mL, which was certificated by Biowest.
Pretreatment of the mycelial powder Prior to the extraction of the polysaccharides, a supercritical fluid carbon dioxide (SC-CO2, 99.5% in purity) extraction was performed to remove some inherently existing oil soluble substances in order to minimize the interference to the polysaccharides, according to a previous report[23]. Briefly, 100 g of the lyophilized mycelial powder was weighed and added with 5% n-hexane to serve as a modifier. The extraction was carried out at 60 °C and 5000 psi in a supercritical gluid extraction apparatus (ISCO SFXTM 2-10, Isco, Lincoln, NE, USA) attached with a modified extraction vessel. In the beginning, a dynamic continuous extraction was adopted at a flow rate of 1 mL SC-CO2/min for 1 h, and was then followed by a static extraction for an additional l h. The oil soluble extracts were collected in 95% ethanol. The residue (ACR1) remaining in the extraction vessel was used in the subsequent experi-mentations.
Preparation of AC-1 and AC-2 The method of Ker et al[23] was followed for the preparation of the polysaccharide fraction AC-1 and AC-2. For the AC-1 fraction, ACR1 (100 g) was extracted with a reflux 3 times with 2000 mL double distilled water (DDW) at 90 °C and was constantly stirred at 400 r/min for 2 h. The extracts were filtered with aspiration after cooling; the residue (ACR2) was kept for further experimenta-tion. One mol/L HCl was added to the filtrate to adjust the pH to 4.0, and then a 2 fold volume of ethanol (95%) was added to precipitate the water-soluble polysaccharides, which were collected and further purified in 400 mL of hot water (100 °C). Finally, the water-soluble polysaccharides were precipitated with the addition of a 3 fold volume of ethanol (95%), and then collected and lyophilized (AC-1). For the AC-2 fraction, the residue ACR2 was added with 1000 mL of 2% NaOH and extracted 3 times, and constantly stirred at 400 r/min and refluxed. The extracts were filtered with aspiration after cooling; the residue was stored for the other experimentation. The filtrate was collected and adjusted to pH 4.0 with 12 mol/L H2SO4, and left to stand overnight. The sediment was collected, dialyzed and lyophilized to recover the isoelectric precipitate (AC-2). The yields of the polysaccharide of A camphorata were 2.92% (w/w) in the AC-1 fraction, and 10.38% (w/w) in the AC-2 fraction. The average molecular masses of AC-1 and AC-2 were 508 kDa and 394 kDa by gel permeation chromatography analysis.
Cell viability assay RAW 264.7 cells were plated in a 6 well plate at 2.8×106 cells per well and allowed to adhere to the plate overnight; then the culture medium were refreshed by new medium containing AC-1 or AC-2. After the introduction of AC-1 or AC-2 for 1 h, the cells were stimulated with LPS (500 μg/L). The cell numbers were counted after 18 h of exposure to LPS. Cell viability was examined using trypan blue exclusion and counted using hemocytometer and phase contrast microscopy.
Nitrite determination RAW 264.7 cells were cultured in a 24 well plate at a density of 5×105, 1 d before LPS treatment. The cells were treated with AC-1 or AC-2 1 h before the introduction of 500 μg/L LPS. After LPS treatment for 18 h, the extracellular medium containing nitrite ion (NO2–) was used as an indication of NO production, and the amount of NO2– in the culture medium was determined according to the colorimetric method by using Griess reagent. The isolated supernatants were incubated with an equal volume of Griess reagent and incubated at room temperature for 10 min. Absorbance at 540 nm was then read and compared with known standard solutions of NaNO2.
Protein extraction RAW 264.7 cells were precultured in a 3.5 cm dish for cell lysate extraction 1 d before LPS treatment. The cells were treated with each polysaccharide 1 h prior to the introduction of 500 μg/L LPS. The total cell lysates were prepared by lysing the cells in buffer containing PRO-PREPTM Protein Extraction Solution containing 10 mmol/L NaF and 1 mmol/L orthovanadate at 4 ºC for 15 min and centrifuged at 7500×g at 4 ºC for 30 s. The supernatants containing the protein extracts were stored at -80 ºC for stabilization.
Nuclear extract preparation The cells from the 10 cm dishes were washed twice with phosphate-buffered saline PBS and scraped in 1 mL of PBS. The cells were collected by centrifuging at 7500×g for 30 s, resuspended in 0.4 mL of buffer A [10 mmol/L N-(2-hydroxyethyl)-piperazine-N’-2-ethanesulfonic acid HEPES, pH 7.9, 10 mmol/L KCl, 1.5 mmol/L MgCl2, and 0.5 mmol/L EDTA] at 4 ºC for 10 min. Then nuclei were pelleted by centrifugation at 7500×g for 30 s. The pellets were resuspended in 0.1 mL of buffer C [20 mmol/L HEPES, pH 7.9, 420 mmol/L NaCl, 1.5 mmol/L MgCl2, 0.2 mmol/L EDTA, and 25% glycerol (v/v)] at 4 ºC for 20 min. The suspension was centrifuged by centrifugation at 7500×g for 2 min. The supernatants were collected and stored at -80 ºC until use. Both buffers A and C contained the following protease inhibitors: 0.5 mmol/L phenylmethylsulfonyl, 1 mmol/L orthovanadate, 2 μg/mL pepstatin A, and 2 μg/mL leupeptin.
Western blotting Protein concentration was determined by using the Bio-Rad protein assay reagent. The extracted protein (30 μg) was separated in 8% SDS-PAGE and transferred to a polyvinylidene fluoride PVDF membrane. After blotting, the membrane was incubated with specific primary antibodies overnight at 4 ºC, then further incubated for 1 h with a horseradish peroxidase HRP-conjugated secondary antibody and eventually incubated with SuperSignal West Pico Chemiluminescent Substrate for 2 min. The bounded antibodies were detected by Kodak Digital ScienceTM (Image Station 4000 MM, New Haven, CT, USA).
RT-PCR RAW 264.7 cells were cultured in a 3.5 cm dish the day before LPS treatment. The cells were treated with each inhibitor 1 h prior to the introduction of 500 μg/L LPS. The RNA was extracted with TRIzol reagent and detected by RT-PCR technique. RT was performed on 2 μg of total RNA by random primers (9 mers) and SuperScript II, then 1/20 volume of reaction mixture was pooled, followed by PCR with mouse COX-2 specific primers (5'-CAGCAAATCCTTGCTGTTCC-3' and 5'-TGGGCAAAGAATGCAAACATC-3'), mouse iNOS specific primers (5'-GTCAACTGCAAGA-GAACGGAGAAC-3' and 5'-GAGCTCCTCCAGAGGGTAGGCT-3'), mouse IL-6 specific primers (5'-AGTAAGTTCCT-CTCTGCAAGAGACT-3' and 5'-CACTAGGTTTGCCGAGTAGATCTC-3'), mouse IL-10 specific primers (5'-CGTCGGATCCGCCATGCCTGGCTCACCACTGCT-3' and 5'-CGTCTCTAGATTAGCTTTTCATTTTGATCA-3'), or β-actin specific primers (5'-CCTAAGGCCAACCGTGAAAA-3' and 5'-TCTTCATGGTGCTAGGAGCCA-3'). Cycle numbers of PCR were 25 cycles for each primer. The RT-PCR products were separated on 1% agarose gel and analyzed.
Protein chip assay RayBio Mouse Cytokine Antibody Array I was purchased from RayBiotech (Norcross, GA, USA). It was employed to assay cell culture conditioned medium and used according to the manufacturer’s instruc-tions. The confluent cells were replaced from medium containing 10% FBS to serum-free medium; in the meantime, AC preparations were added into the cells. LPS was added 1 h after AC treatment. The conditioned medium was collected after LPS treatment for 9 h. Twenty-two different cytokines were evaluated: granulocyte-colony stimulating factor (GCSF), granulocyte-macrophage colony stimulating factor (GM-CSF), IL-2, IL-3, IL-4, IL-5, IL-6, IL-9, IL-10, IL-12p40/p70, IL-12p70, IL-13, IL-17, interferon-γ, monocyte chemoat-tractant protein-1 (MCP-1), monocyte chemoattractant protein-5 (MCP-5), regulated upon activation, normal T-cell expressed, and presumably secreted (RANTES), stem cell factor(SCF), sTNFR1, TNF-α, thrombopoietin, and vascular endothelial growth factor. The bounded cytokines were detected by biotin-labeled antibodies and horseradish peroxidase-labeled streptavidin, and were detected by SuperSignal West Pico Chemiluminescent Substrate for 2 min. The bounded antibodies were analyzed by Kodak Digital ScienceTM (Image Station 4000MM).
Statistical analysis Differences among the data of the LPS-treated control and further treatments with each polysaccharide fractions were analyzed by Student’s t-test. Statistical probability was expressed as P<0.05.
Results
Effects of AC-1 and AC-2 on the viability of RAW 264.7 cells Before testing the effects of AC-1 and AC-2 on LPS-induced nitrite and COX-2 expression, we tested the effects of AC-1 and AC-2 on cell viability. After 18 h treatment of AC-1 or AC-2 and LPS in mouse macrophage RAW 264.7 cells, cell numbers were counted individually. The results presented in Figure 1 demonstrate that cell numbers were not affected by AC-1 at 100 mg/L or AC-2 at 50 mg/L. A minute decrease was found at 200 mg/L of AC-1 mg/L or 100 mg/L, and 200 mg/L of AC-2 (Figure 1). After statistical analysis, we suggested that there was no significant cytotoxicity under 200 mg/L of AC-1 or AC-2. Further study on the inflammation-related gene expression affected by AC-1 and AC-2 under the concentration of 200 mg/L was conducted.
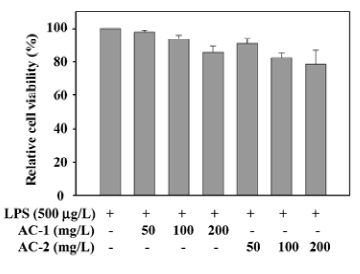
Effects of AC-1 and AC-2 on LPS-induced NO production NO synthesized by iNOS has been implicated as a mediator of inflammation. The inhibitory effects of AC-1 and AC-2 on LPS-induced NO production were investigated. As shown in Figure 2, NO production was greatly increased after LPS treatment for 18 h. Only AC-2, but not AC-1, showed a dose-dependent reduction of NO production between 50 and 200 mg/L. This suggests that the AC-2 fraction has the ability to inhibit LPS-induced NO production.
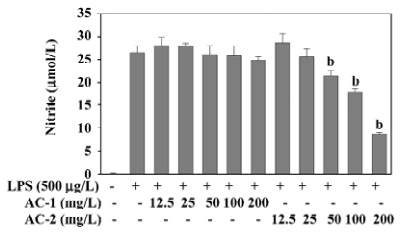
Effects of AC-1 and AC-2 on LPS-induced expression of the iNOS protein and mRNA In general, iNOS is not present in the resting cells, but is induced by various stimuli. Increased expression of iNOS has been associated with inflammatory disorders. Because LPS-induced NO production could be reduced by AC-2, we further tested the effects of AC-1 and AC-2 on the LPS-induced iNOS protein and mRNA expression. As shown in Figure 3, AC-2, but not AC-1, dose-dependently inhibited the LPS-induced iNOS protein (Figure 3A) and mRNA (Figure 3B) expression in RAW 264.7 cells. This suggests that the inhibitory effects of AC-2 on LPS-induced NO production is due to the suppression of LPS-induced iNOS gene expression.
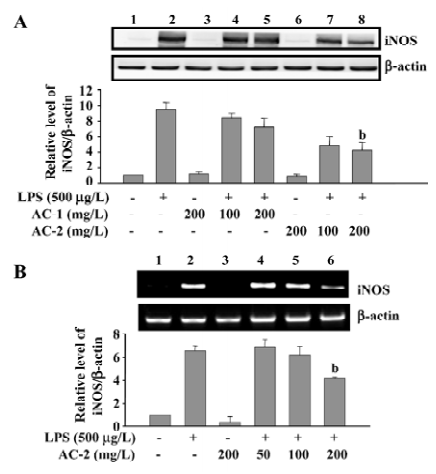
Effects of AC-1 and AC-2 on LPS-induced expression of COX-2 protein and mRNA Arachidonic acid is released from the cell membrane catalyzed by phospholipase A2, converted into PGH2 by cyclooxygenase, and further metabolized to PGE2. The effects of AC-1 and AC-2 on the expression of the COX-2 protein and mRNA were investigated. As shown in Figure 4, 200 mg/L AC-1 or 200 mg/L AC-2 did not suppress either the LPS-induced COX-2 protein (Figure 4A) or mRNA (Figure 4B) expression. Furthermore, we detected the PGE2 concentration in the culture medium. We also found that that 200 mg/L AC-1 or 200 mg/L AC-2 did not inhibit LPS-induced PEG2 production (data not shown). These data suggest that AC-1 and AC-2 had no inhibitory effect on LPS-induced COX-2 gene expression and PGE2 production.
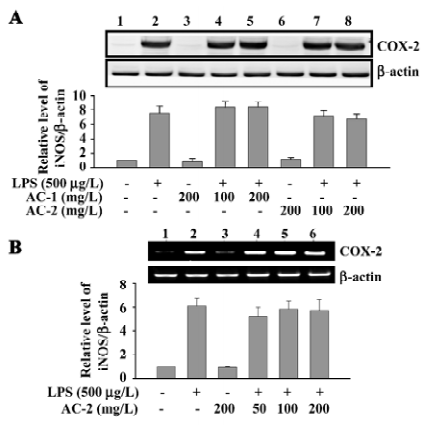
Effects of AC-1 and AC-2 on LPS-induced cytokine expression In order to widely observe the effects of AC-1 and AC-2 on LPS-induced cytokine expression, the mouse cytokine antibody array (Figure 5A) was applied. When the cells were incubated with AC-1 or AC-2 for 10 h, neither AC-1 nor AC-2 changed the cytokine expression pattern, compared to the control cells (Figure 5Bi, iii, v). After LPS treatment for 9 h, the protein expression of GCSF, GM-CSF, IL-6, IL-10, MCP-1, MCP-5, RANTES, sTNFRI, and TNF-α all increased (Figure 5Bi, ii). Each LPS-increased dot was quantified and the result is shown in Figure 5C. It indicated that AC-2, but not AC-1, inhibited LPS-induced protein expression of IL-6, IL-10, MCP-5 and RANTES, and neither AC-1 nor AC-2 inhibited LPS-induced GCSF, GM-CSF, MCP-1, sTNFRI, and TNF-α protein secretion in mouse macrophages.
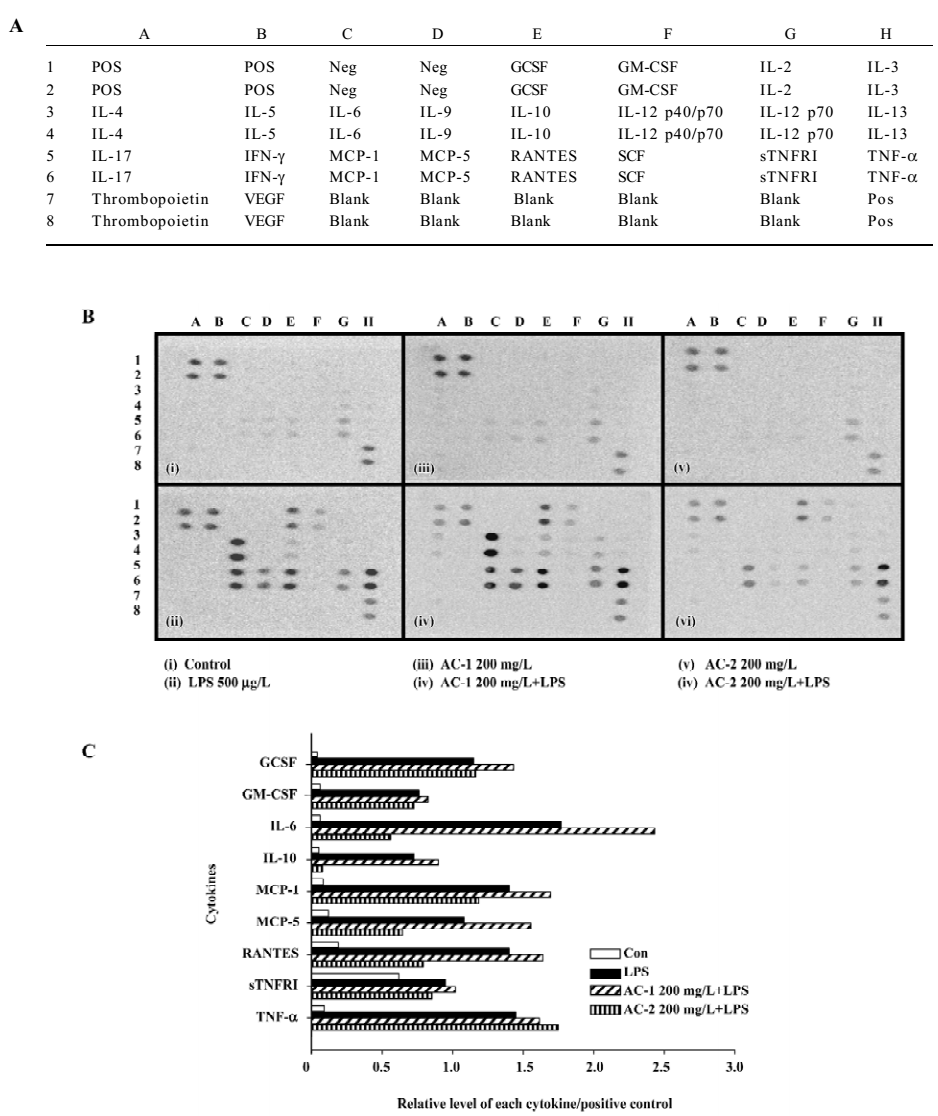
Effects of AC-2 on LPS-induced IL-6 and IL-10 mRNA expression We further identified whether AC-2 inhibited LPS-induced mRNA expression of IL-6 and IL-10. The mRNA expression of IL-6 and IL-10 were monitored by RT-PCR. AC-2 dose-dependently inhibited LPS-induced IL-6 mRNA expression (Figure 6A); it also inhibited IL-10 mRNA expression (Figure 6B). The inhibitory effect on LPS-induced IL-6 and IL-10 gene expression by AC-2 is important in the regulation of IL-6 and IL-10 genes in mouse macrophages.
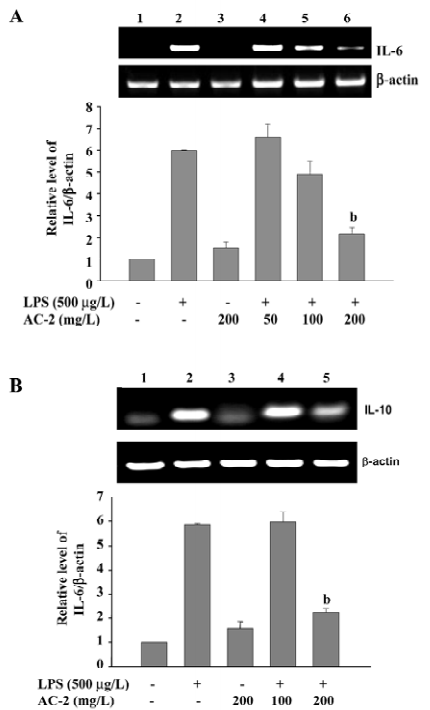
Effects of AC-2 on TLR4 and LPS-induced NF-κB translocation Because AC-2 has the ability to repress some specific gene activation by LPS, we further identified the effects of AC-2 on the upstream linkage of LPS signaling. TLR4 is a membrane receptor for the recognition of LPS. After binding to LPS, it will activate the NF-κB pathway to induce the expression of many inflammatory genes[24]. Next, we analyzed whether TLR4 and NF-κB were affected by LPS and AC-2. As shown in Figure 7A, the protein expression of the TLR4 receptor was unchanged after LPS and AC-2 treatment. NF-κB p65 translocation is one of the important signal pathways activated by LPS; therefore, the effect of AC-2 on LPS-induced NF-κB p65 translocation was monitored. It showed that LPS increased the nuclear translocation of p65, and AC-2 inhibited LPS-induced p65 translocation (Figure 7B).
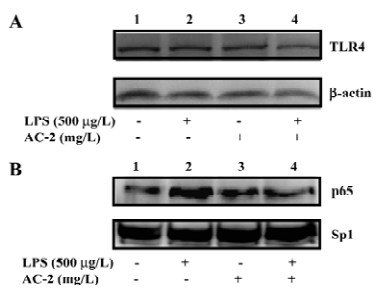
Discussion
A camphorata (Polyporaceae, Aphyllophorales), the cause of brown heart rot of Cinnamomum kanahirai Hay in Taiwan, is a new basidiomycete and a scarce traditional medicine that has attracted great attention due to its antioxidant and antitumor effects in vivo and in vitro. It was identified in 1990 as a traditional Chinese medicine in Taiwan. The present study was undertaken to elucidate the pharmacological and molecular effects of the partially purified polysaccharide fraction from A camphorate on LPS-induced inflammatory mediators in macrophages. The results indicate that AC-2 is an effective inhibitor of LPS-induced inflammatory mediators such as IL-6, IL-10, MCP-5, RANTES, and NO. This indicates that the polysaccharide fraction of A camphorate appears to have some potential bioactive compounds in inhibiting LPS-induced inflammatory gene expression, including IL-6, IL-10, iNOS, MPC-5, and RANTES.
IL-6 was originally identified as a B-cell differentiation factor, but it is now known to play a central role in host defense because it is released in response to infection, burns, trauma and tumor, and its functions range from key roles in acute phase protein induction to B- and T-cell growth and differentiation[25]. IL-6 can induce a variety of acute-phase proteins, such as fibrinogen, serum amyloid A, and the C-reactive protein in human hepatocytes[26]. IL-6-deficient mice also show a severely defective inflammatory acute-phase response after tissue damage or infection[27]. An unregulated, high-level production of IL-6 could generate an undesired inflammatory state, a circumstance that can cause various diseases. Several reports indicate that IL-6 is implicated in the pathogenesis of a number of human disorders, including rheumatoid arthritis and inflammatory bowel disease[28,29]. AC-2, a partially purified polysaccharide fraction from A camphorate could inhibit LPS-induced IL-6 gene expression, which could provide a therapeutic clue in IL-6-related diseases.
IL-10 was discovered as a cytokine synthesis inhibiting factor, and its principal function is the activity inhibition of Th1 cells[30]. IL-10 has multiple biological functions on different cell types. In macrophages, IL-10 inhibits ligand-induced activation and the production of pro-inflammatory cytokines from macrophages[31,32]. IL-10 inhibits the proliferation as well as cytokine synthesis of CD4+ T-cells[33]. Other immunosuppressive effects have also been reported on eosinophils[34], neutrophils[35], and dendritic cells[36]. In contrast to these immunosuppressive effects, IL-10 has been shown to have the abilities of immunostimulation on cytotoxic T-cells[37], and is a growth costimulator for thymocytes and mast cells[38]. In this study, AC-2 also inhibited LPS-induced IL-10 gene expression.
Besides IL-6 and IL-10, AC-2 also reduced LPS-induced iNOS gene expression and NO release in RAW 264.7 cells. The expression inhibition of the iNOS protein responsible for NO inhibition by A camphorata had been reported[6]. Here we suggested for the first time that A camphorata reduced LPS-induced NO production by inhibiting iNOS mRNA expression. Although nanomolar concentrations of NO play an important physiological role as a defense molecule in the immune system[39], overproduction of NO, predominantly via the upregulation of iNOS in macrophages, contributes to numerous pathological processes, including inflammation[40] and atherosclerosis[41]. The mechanism responsible for NO inhibition of several plant extracts had been reported, including direct scavenging of NO[42], suppression of iNOS activity[43], or the reduction on iNOS gene expression[44]. In the present data, the AC-2 fraction of A camphorata expressed anti-inflammatory effects via iNOS gene inhibition. This inhibition may in part be through inhibiting LPS-activated NF-κB translocation (Figure 7B). This data suggests that the AC-2 polysaccharide fraction of A camphorate could be an attractive candidate for adjunctive therapy in gram-negative bacterial infections.
AC-2 also has the ability to repress the secretion of MCP-5 and RANTES. MCP-5 is a potent monocyte active chemokine that is involved in allergic inflammation[45]; RANTES is also an important chemoattractant for many immune cells[46]. Some molecules inhibit LPS-activated macrophages by interfering LPS binding to cell surface[47,48]. In this study, it is not likely that AC-2-inhibited cytokine expression is due to interferring LPS binding to its receptor TLR4 because the protein expression of TLR4 does not change after AC-2 treatment (Figure 7A). LPS-induced COX-2, GCSF, GM-CSF, MCP-1, sTNFRI, and TNF-α were not reduced simultaneously. Although the inhibition of COX-2 protein expression by A camphorata had been reported[6], there was no such bioactive constituent in this polysaccharide fraction of A camphorata. It also suggests that the bioactive compounds for inhibiting COX-2 and iNOS existed in different components of A camphorata. However, the characterization of candidate compounds that mediate the inhibition of LPS-induced NO, IL-6, IL-10, MCP-5, and RANTES secretion requires further study.
In conclusion, the present study shows that the fractionated polysaccharides AC-2 of A camphorata inhibits the production of IL-6, IL-10, MPC-5, RANTES, and NO in LPS-stimulated mouse macrophages. This inhibition of IL-6, IL-10, and iNOS was mediated by transcriptional downregula-tion of IL-6, IL-10, and iNOS genes. Because IL-6, IL-10, MCP-5, RANTES, and NO are thought to be associated with acute and chronic inflammation diseases, the inhibitory effects of A camphorata on LPS-induced IL-6, IL-10 MCP-5, RANTES, and NO might provide a partial anti-inflammation function in LPS-induced inflammatory conditions.
References
- Tsai Z, Liaw S. The use and the effect of . Taichung, Taiwan: Sheng-Tun Publishers; 1982.
- Hseu YC, Chang WC, Hseu YT, Lee CY, Yech YJ, Chen PC, et al. Protection of oxidative damage by aqueous extract from Antrodia camphorata mycelia in normal human erythrocytes. Life Sci 2002;71:469-82.
- Song TY, Yen GC. Protective effects of fermented filtrate from Antrodia camphorata in submerged culture against CCl4-induced hepatic toxicity in rats. J Agric Food Chem 2003;51:1571-7.
- Hsiao G, Shen MY, Lin KH, Lan MH, Wu LY, Chou DS, et al. Antioxidative and hepatoprotective effects of Antrodia campho-rata extract. J Agric Food Chem 2003;51:3302-8.
- Shen YC, Chou CJ, Wang YH, Chen CF, Chou YC, Lu MK. Anti-inflammatory activity of the extracts from mycelia of Antrodia camphorata cultured with water-soluble fractions from five different Cinnamomum species. FEMS Microbiol Lett 2004;231:137-43.
- Hseu YC, Wu FY, Wu JJ, Chen JY, Chang WH, Lu FJ, et al. Anti-inflammatory potential of Antrodia Camphorata through inhibition of iNOS, COX-2 and cytokines via the NF-kappaB pathway. Int Immunopharmacol 2005;5:1914-25.
- Hseu YC, Yang HL, Lai YC, Lin JG, Chen GW, Chang YH. Induction of apoptosis by Antrodia camphorata in human premyelo-cytic leukemia HL-60 cells. Nutr Cancer 2004;48:189-97.
- Hsu YL, Kuo YC, Kuo PL, Ng LT, Kuo YH, Lin CC. Apoptotic effects of extract from Antrodia camphorata fruiting bodies in human hepatocellular carcinoma cell lines. Cancer Lett 2005;221:77-89.
- Song TY, Hsu SL, Yen GC. Induction of apoptosis in human hepatoma cells by mycelia of Antrodia camphorata in submerged culture. J Ethnopharmacol 2005;100:158-67.
- Song TY, Hsu SL, Yeh CT, Yen GC. Mycelia from Antrodia camphorata in submerged culture induce apoptosis of human hepatoma HepG2 cells possibly through regulation of Fas pathway. J Agric Food Chem 2005;53:5559-64.
- Lee IH, Huang RL, Chen CT, Chen HC, Hsu WC, Lu MK. Antrodia camphorata polysaccharides exhibit anti-hepatitis B virus effects. FEMS Microbiol Lett 2002;209:63-7.
- Jong SC, Birmingham JM. Medicinal and therapeutic value of the shiitake mushroom. Adv Appl Microbiol 1993;39:153-84.
- Mizuno T, Wasa T, Ito H, Suzuki C, Ukai N. Antitumor-active polysaccharides isolated from the fruiting body of Hericium erinaceum, an edible and medicinal mushroom called yamabu-shitake or houtou. Biosci Biotechnol Biochem 1992;56:347-8.
- Liu JJ, Huang TS, Hsu ML, Chen CC, Lin WS, Lu FJ, et al. Antitumor effects of the partially purified polysaccharides from Antrodia camphorata and the mechanism of its action. Toxicol Appl Pharmacol 2004;201:186-93.
- Cheng JJ, Yang CJ, Cheng CH, Wang YT, Huang NK, Lu MK. Characterization and functional study of Antrodia camphorata lipopolysaccharide. J Agric Food Chem 2005;53:469-74.
- Green SJ, Nacy CA, Meltzer MS. Cytokine-induced synthesis of nitrogen oxides in macrophages: a protective host response to Leishmania and other intracellular pathogens. J Leukoc Biol 1991;50:93-103.
- Lorsbach RB, Murphy WJ, Lowenstein CJ, Snyder SH, Russell SW. Expression of the nitric oxide synthase gene in mouse macrophages activated for tumor cell killing. Molecular basis for the synergy between interferon-gamma and lipopolysaccharide. J Biol Chem 1993;268:1908-13.
- Nussler AK, Billiar TR. Inflammation, immunoregulation, and inducible nitric oxide synthase. J Leukoc Biol 1993;54:171-8.
- Sano H, Hla T, Maier JA, Crofford LJ, Case JP, Maciag T, et al. In vivo cyclooxygenase expression in synovial tissues of patients with rheumatoid arthritis and osteoarthritis and rats with adjuvant and streptococcal cell wall arthritis. J Clin Invest 1992;89:97-108.
- Kargman SL, O’Neill GP, Vickers PJ, Evans JF, Mancini JA, Jothy S. Expression of prostaglandin G/H synthase-1 and -2 protein in human colon cancer. Cancer Res 1995;55:2556-9.
- O’Sullivan MG, Chilton FH, Huggins EM Jr, McCall CE. Lipopolysaccharide priming of alveolar macrophages for enhanced synthesis of prostanoids involves induction of a novel prostaglandin H synthase. J Biol Chem 1992; 267: 14 547–50.
- Chen LC, Chen BK, Chang JM, Chang WC. Essential role of c-Jun induction and coactivator p300 in epidermal growth factor-induced gene expression of cyclooxygenase-2 in human epidermoid carcinoma A431 cells. Biochim Biophys Acta 2004; 1683: 38–48.
- Ker YB, Chen KC, Chyau CC, Chen CC, Guo JH, Hsieh CL, et al. Antioxidant capability of polysaccharides fractionated from submerge-cultured Agaricus blazei mycelia. J Agric Food Chem 2005;53:7052-8.
- Akira S, Takeda K. Toll-like receptor signalling. Nat Rev Immunol 2004;4:499-511.
- Kamimura D, Ishihara K, Hirano T. IL-6 signal transduction and its physiological roles: the signal orchestration model. Rev Physiol Biochem Pharmacol 2003;149:1-38.
- Castell JV, Gomez-Lechon MJ, David M, Hirano T, Kishimoto T, Heinrich PC. Recombinant human interleukin-6 (IL-6/BSF-2/HSF) regulates the synthesis of acute phase proteins in human hepatocytes. FEBS Lett 1988;232:347-50.
- Kopf M, Baumann H, Freer G, Freudenberg M, Lamers M, Kishimoto T, et al. Impaired immune and acute-phase responses in interleukin-6-deficient mice. Nature 1994;368:339-42.
- Koss K, Satsangi J, Welsh KI, Jewell DP. Is interleukin-6 important in inflammatory bowel disease? Genes Immun 2000;1:207-12.
- Wong PK, Campbell IK, Egan PJ, Ernst M, Wicks IP. The role of the interleukin-6 family of cytokines in inflammatory arthritis and bone turnover. Arthritis Rheum 2003;48:1177-89.
- Fiorentino DF, Bond MW, Mosmann TR. Two types of mouse T helper cell. IV. Th2 clones secrete a factor that inhibits cytokine production by Th1 clones. J Exp Med 1989;170:2081-95.
- Fiorentino DF, Zlotnik A, Mosmann TR, Howard M, O’Garra A. IL-10 inhibits cytokine production by activated macrophages. J Immunol 1991;147:3815-22.
- de Waal Malefyt R, Abrams J, Bennett B, Figdor CG, de Vries JE. Interleukin 10 (IL-10) inhibits cytokine synthesis by human monocytes: an autoregulatory role of IL-10 produced by mono-cytes. J Exp Med 1991;174:1209-20.
- Del Prete G, De Carli M, Almerigogna F, Giudizi MG, Biagiotti R, Romagnani S. Human IL-10 is produced by both type 1 helper (Th1) and type 2 helper (Th2) T cell clones and inhibits their antigen-specific proliferation and cytokine production. J Immunol 1993;150:353-60.
- Takanaski S, Nonaka R, Xing Z, O’Byrne P, Dolovich J, Jordana M. Interleukin 10 inhibits lipopolysaccharide-induced survival and cytokine production by human peripheral blood eosinophils. J Exp Med 1994;180:711-5.
- Jenkins JK, Malyak M, Arend WP. The effects of interleukin-10 on interleukin-1 receptor antagonist and interleukin-1 beta production in human monocytes and neutrophils. Lymphokine Cytokine Res 1994;13:47-54.
- Beinhauer BG, McBride JM, Graf P, Pursch E, Bongers M, Rogy M, et al. Interleukin 10 regulates cell surface and soluble LIR-2 (CD85d) expression on dendritic cells resulting in T cell hypores-ponsiveness in vitro. Eur J Immunol 2004;34:74-80.
- Cohen SB, Crawley JB, Kahan MC, Feldmann M, Foxwell BM. Interleukin-10 rescues T cells from apoptotic cell death: association with an upregulation of Bcl-2. Immunology 1997;92:1-5.
- Conti P, Kempuraj D, Kandere K, Di Gioacchino M, Barbacane RC, Castellani ML, et al. IL-10, an inflammatory/inhibitory cytokine, but not always. Immunol Lett 2003;86:123-9.
- Hobbs AJ, Higgs A, Moncada S. Inhibition of nitric oxide synthase as a potential therapeutic target. Annu Rev Pharmacol Toxicol 1999;39:191-220.
- Bogdan C. Nitric oxide and the immune response. Nat Immunol 2001;2:907-16.
- Dusting GJ. Nitric oxide in coronary artery disease: roles in atherosclerosis, myocardial reperfusion and heart failure. Exs 1996;76:33-55.
- Bor JY, Chen HY, Yen GC. Evaluation of antioxidant activity and inhibitory effect on nitric oxide production of some common vegetables. J Agric Food Chem 2006;54:1680-6.
- Aktan F, Henness S, Roufogalis BD, Ammit AJ. Gypenosides derived from Gynostemma pentaphyllum suppress NO synthesis in murine macrophages by inhibiting iNOS enzymatic activity and attenuating NF-kappaB-mediated iNOS protein expression. Nitric Oxide 2003;8:235-42.
- Wadsworth TL, Koop DR. Effects of Ginkgo biloba extract (EGb 761) and quercetin on lipopolysaccharide-induced release of nitric oxide. Chem Biol Interact 2001;137:43-58.
- Sarafi MN, Garcia-Zepeda EA, MacLean JA, Charo IF, Luster AD. Murine monocyte chemoattractant protein (MCP)-5: a novel CC chemokine that is a structural and functional homologue of human MCP-1. J Exp Med 1997;185:99-109.
- Appay V, Rowland-Jones SL. RANTES: a versatile and controversial chemokine. Trends Immunol 2001;22:83-7.
- An SJ, Pae HO, Oh GS, Choi BM, Jeong S, Jang SI, et al. Inhibition of TNF-alpha, IL-1beta, and IL-6 productions and NF-kappa B activation in lipopolysaccharide-activated RAW 264.7 macrophages by catalposide, an iridoid glycoside isolated from Catalpa ovata G Don (Bignoniaceae). Int Immunopharmacol 2002;2:1173-81.
- Yan YJ, Li Y, Lou B, Wu MP. Beneficial effects of ApoA-I on LPS-induced acute lung injury and endotoxemia in mice. Life Sci 2006;79:210-5.