VGluT1- and GAD-immunoreactive terminals in synaptic contact with PAG-immunopositive neurons in principal sensory trigeminal nucleus of rat1
Introduction
The trigeminal sensory nuclear complex was originally divided into 4 nuclei: principalis (Vp), oralis (Vo), interpolaris (Vi), and caudalis (Vc) by Olszewski[1]. Vp comprises a ventral division and a dorsal division (Vpd), which are cyto-architectonically different and have been proposed to serve the different functions of relaying and processing of sensory signals from the head, such as tactility and trigeminal proprioception[2–4]. Such functions of the Vpd can be supported by the findings that this structure receives direct primary input from intraoral structures and possesses diverse afferent and efferent fiber connections with other brain areas[5–8].
It is well known that glutamate and gamma-aminobutyric acid (GABA) are important excitatory and inhibitory neutrotransmitters in the brain, respectively. Several lines of evidence suggest that these transmitters are involved in signal transmission or processing within the Vpd[9–11]. The Vpd contains a high density of different-sized, immunohisto-chemically heterogeneous neurons, many of which are likely to contain glutamate[12], and constitute most of the Vpd projection neurons[11]; therefore, these neurons play essential roles in relaying mechanical sensory signals from the head. In addition, the observation that myelinated trigeminal primary afferent terminals are immunoreactive for glutamate implies the glutamatergic nature of their associated input to the Vpd[9]. However, in the central nervous system (CNS), glutamate can also serve as the substrate for GABA synthesis, and phosphate-activated glutaminase (PAG), a marker for glutamatergic structures, was expressed only in the glutamatergic cell bodies and dendrites[13]. So because of the lack of suitable immunocytochemical markers for glutamatergic axons, an understanding of the sensory transmission through the glutamatergic primary afferents from the orofacial area has been hampered. Recently, 3 vesicular glutamate transporters, vesicular glutamate transporter 1 (VGluT1), VGluT2 and VGluT3, have been identified and shown to be present selectively in axons belonging to largely nonoverlapping populations of glutamatergic neurons throughout the CNS[14–16]. Previous immunocytochemical studies have provided evidence that VGluT1 is strongly expressed in the myelinated afferent fibers of the Vpd, whereas VGluT2 and VGluT3-IRs are expressed at very low levels[17,18]. On the other hand, many axon terminals in the Vpd were immunostained for GABA[9]. Based on the aforementioned studies, we infer that VGluT1-LI axon terminals, coming from trigeminal primary afferents, could activate Vpd glutamatergic projection neurons which may be modulated by GABA-LI axon terminals. Therefore, an attempt was made to examine whether or not the VGluT1-LI axon terminals of the Vpd come from the primary afferent fibers by performing a trigeminal rhizotomy in rats, and examining the relationship between the PAG-LI neurons and VGluT1/glutamate decarboxylase (GAD; a marker for GABAergic neurons)-LI axon terminals in the Vpd under the confocal laser-scanning microscope (CLSM) and electron microscope (EM).
Materials and methods
All procedures for the experiments were approved by the Animal Care and Use Committees at the Fourth Military Medical University, Xi’an, China. A total of 10 adult Sprague-Dawley male rats weighing 200–250 g were used. The rats were anesthetized with chloral hydrate (70 mg/100 g body weight) and perfused transcardially for light and electron microscopic experiments[19].
Triple-immunofluorescence histochemistry for VGluT1, GAD and PAG was performed. Briefly, the sections were incubated at room temperature sequentially with: (1) a mixture of 50 µg/mL mouse anti-PAG IgM[20] and 0.8 µg/mL guinea pig anti-VGluT1 IgG[21] or mouse anti-GAD IgG (1:500; Chemicon, Temecula, CA, USA) overnight; (2) biotinylated donkey anti-mouse IgM (1:100; Jackson ImmunoResearch, West Grove, PA, USA) for 4 h; and (3) a mixture of 10% (v/v) normal mouse serum, fluorescein isothiocyanate (FITC)-labeled avidin-D (1:1000; Vector Labs, Burlingame, CA, USA) and rhodamine-labeled goat anti-guinea pig IgG (1:100; Chemicon, USA) and Cy5-labeled donkey anti-mouse IgG (1:100; Chemicon, USA) for 3 h. The incubation medium in steps 1 and 2 was prepared by using 0.05 mol/L phosphate-buffered 0.9% saline (PBS) containing 0.5% (v/v) Triton X-100, 0.25% (w/v) λ-carrageenan, 0.05% (w/v) NaN3 and 5% (v/v) normal donkey serum. The incubation medium in step 3 was prepared by using 0.05 mol/L PBS containing 0.3% (v/v) Triton X-100. After the incubation, the sections were rinsed in 0.05 mol/L PBS, mounted onto gelatin-coated glass slides, and then observed under the CLSM (LSM 410; Zeiss, Oberkochen,Germany) by using a laser beam of 488 nm, 543 nm and 633 nm with appropriate emission filters for FITC (510–525 nm), rhodamine (590–610 nm) and Cy5 (670–810 nm). In the control experiments for the immunofluorescence histochemistry, one of the primary antibodies was omitted or replaced with normal IgG; no immunoreactivity for the omitted or replaced antibody was found.
Electron microscopically, the immunogold-silver method for VGluT1 or GAD, combined with the immunoperoxidase method for PAG, was performed. Briefly, the sections were incubated for 24 h at room temperature with a mixture of 0.8 µg/mL guinea pig anti-VGluT1 IgG or 1/500-diluted mouse anti-GAD IgG (Chemicon, USA) and 75 µg/mL mouse anti-PAG IgM, each diluted in 50 mmol/L Tris-buffered saline (TBS; pH 7.4) containing 2% (v/v) normal goat serum (TBS–NGS). Then the sections were washed in TBS and further incubated overnight at room temperature with a mixture of 1/100-diluted biotinylated donkey anti-mouse IgM antibody (Jackson ImmunoResearch, USA) and 1/100-diluted anti-guinea pig IgG or anti-mouse IgG antibody conjugated to 1.4 nm gold particles (Nanoprobes; Stony Brook, NY, USA), each diluted in TBS-NGS. Subsequently the sections were processed for: (1) 1% postfixation with glutaraldehyde in 0.1 mol/L phosphate buffer PB for 10 min; (2) silver enhancement with an HQ Silver Kit (Nanoprobes, Stony Brook, NY, USA); (3) incubation with an ABC reagent (Vector Labs, Burlingame, CA, USA) diluted at 1:50 in 50 mmol/L TBS for 3 h at room temperature; (4) visualization of PAG-immunoreactivity by incubation with diaminobenzidine tetrahydro-chloride and H2O2; (5) osmification with 1% OsO4 in 0.1 mol/L PB for 1 h; (6) counterstaining with uranyl acetate; and (7) flat-embedding in Durcupan (Fluka, Buchs, Switzerland) after dehydration and mounting on silicon-coated glass slides. Ultrathin sections were prepared and examined as described[19].
Trigeminal rhizotomy was prepared as described[22]. Briefly, after anesthesia, unilateral trigeminal rhizotomy was performed on 3 rats. A 10-day postoperative period was allowed for degeneration, and then the rats were fixed and tissue was prepared for VGluT1 immunohistochemistry as mentioned earlier. The sections containing the Vpd were incubated sequentially at room temperature with: (1) 0.5 µg/mL guinea pig anti-VGluT1 IgG overnight; (2) 1/100-diluted biotinylated anti-guinea pig IgG donkey antibody (Jackson ImmunoResearch, USA) for 4 h; and (3) 1/50-diluted avidin-biotinylated peroxidase complex (Vector Labs, USA) for 2 h. The incubation medium was the same as those used in the immunofluorescence histochemistry. Subsequently the sections were reacted with 0.02% (w/v) 3,3'-diaminobenzidine tetrahydrochloride and 0.003% H2O2 (v/v) for 10–30 min in 0.05 mol/L Tris-HCl buffer (pH 7.6).
Results
Under CLSM, the bodies of PAG-LI neurons were easily identified with FITC fluorescence (green channel); VGluT1-LI axon terminals with rhodamine fluorescence (red channel) and GAD-LI axon terminals with Cy5 fluorescence (blue channel). The PAG-IR was seen in small or medium-sized cell bodies of the Vpd neurons. On the other hand, VGluT1-IR was found mostly in the varicosities’ characteristic of axon terminals. We also found many GAD-LI axon varicosities distributed in the Vpd. Both the VGluT1- and GAD-LI axon terminals were frequently seen in close apposition to PAG-LI cell bodies, and there were some VGluT1- and GAD-LI axonal terminals which formed close appositions on the same PAG-LI cell body (Figure 1).
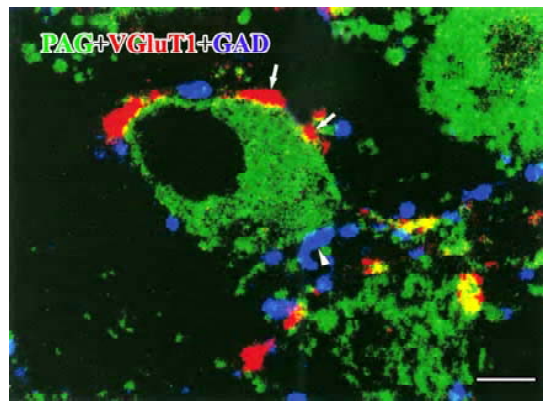
Under the EM, some terminals were labeled with immunogold-silver grains indicating VGluT1-LI axon terminals. Most of the VGluT1-LI terminals contained abundant small clear round vesicles, and a number of VGluT1-LI terminals made asymmetric synaptic contacts with PAG-positive dendritic profiles (Figure 2A). No VGluT1-LI axon terminals containing flattened vesicles and forming symmetrical synapses were found. On the other hand, in the material processed for dual immunohistochemical labeling of GAD/PAG, most of the GAD-LI terminals made symmetric synapses with PAG-LI dendritic profiles (Figure 2B).
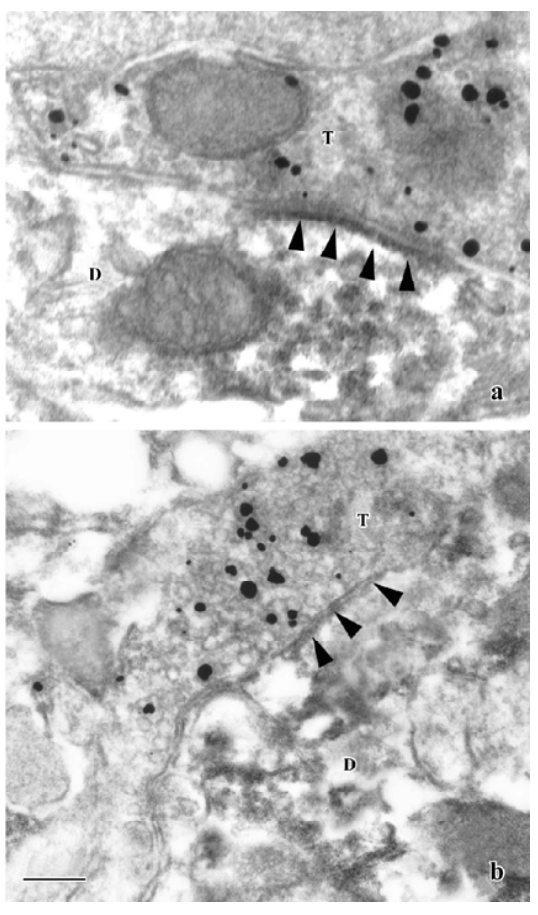
It was examined whether or not VGluT1-IR is expressed in primary afferent fibers. After unilateral trigeminal rhizotomy, it was clearly shown that VGluT1-IR was less intense in the Vpd (Figure 3A) on the side ipsilateral to the trigeminal rhizotomy compared to the contralateral side (Figure 3B, 3C). Thus, the trigeminal rhizotomy experiments indicated that VGluT1 was expressed in primary afferent fibers terminating within the Vpd.
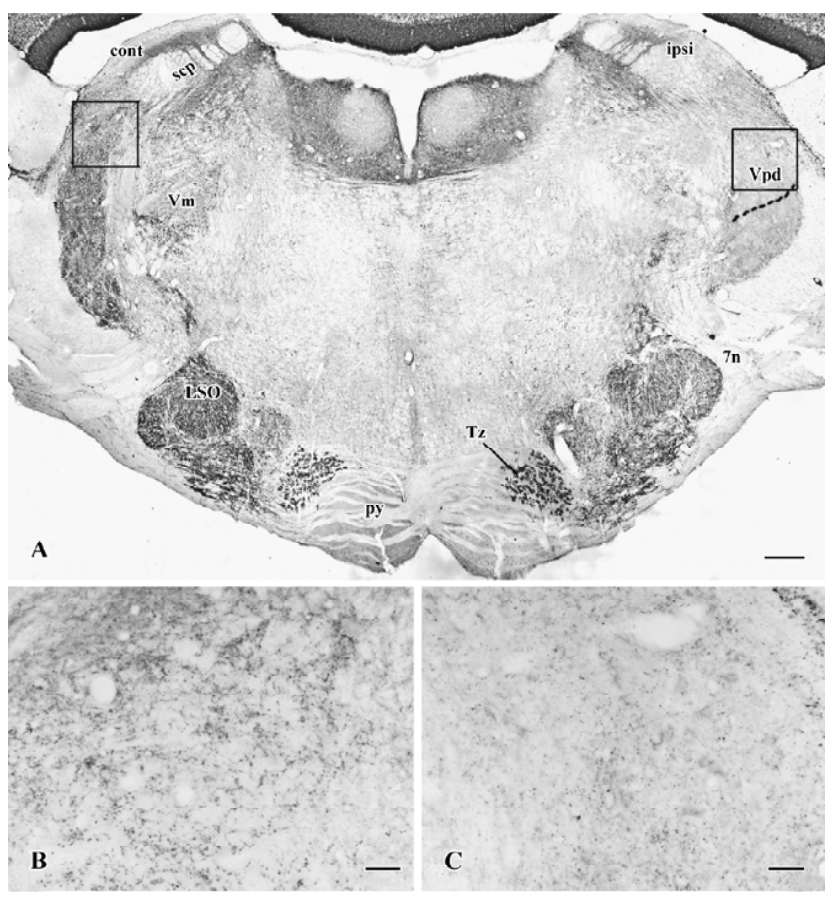
Discussion
The results of present study show that PAG-LI neurons receive orofacial sensory information transmitted from the VGluT1-LI axon terminals, mainly arising from primary afferent fibers of the trigeminal ganglion. It has been reported that most of the neurons in the Vp projecting to the ventro-posterior medial nucleus of the thalamus are glutamatergic[9,11] and that glutamate-IR is present in some primary afferent terminals and functions as an important excitatory transmitter involved in the relay of sensory information to the Vp[10]. Recently, Varoqui et al suggested that mechanoreception at the Vp predominantly involved VGluT1 expressing axonal terminals[17]. Combined with the previous studies, our present results suggest that glutamatergic axon terminals might act on the PAG-LI neurons in the Vpd and transmit the orofacial region non-nociceptive sensory information to them.
Bae et al[9] tested whether GABA could act on the glutamatergic primary afferents through the axoaxonic synapses to modulate the transmission of the orofacial sensory information in the Vpd. In the present study, we observed that the GAD-LI axon terminals formed symmetric synapses with PAG-LI neurons and inferred that the GABA functioned as an inhibitory transmitter acting on the neurons of the Vpd in addition to the primary afferent fibers.
In summary, the present results, together with the previously reported ones, provide strong anatomical evidence that Vpd neurons receive trigeminal orofacial sensory information from VGluT1 expressing glutamatergic terminals and that GABAergic postsynaptic regulation might occur at the level of the Vpd neurons.
References
- Olszewski J. On the anatomy and functional organization of the spinal trigeminal nucleus. J Comp Neurol 1950;92:401-13.
- Luo PF, Wang BR, Peng ZZ, Li JS. Morphological characteristics and terminating patterns of masseteric neurons of the mesencephalic trigeminal nucleus in the rat: an intracellular horseradish peroxidase labeling study. J Comp Neurol 1991;303:286-99.
- Minnery BS, Simons DJ. Response properties of whisker-associated trigeminothalamic neurons in rat nucleus principalis. J Neurophysiol 2003;89:40-56.
- Shigenaga Y, Nakatani Z, Nishimori T, Suemune S, Kuroda R, Matano S. The cells of origin of cat trigeminothalamic projec-tions: especially in the caudal medulla. Brain Res 1983;277:201-22.
- Moritani M, Yoshida A, Honma S, Nagase Y, Takemura M, Shigenaga Y. Morphological differences between fast and slowly adapting lingual afferent terminations in the principal and oral nuclei in the cat. J Comp Neurol 1998;396:64-83.
- Shigenaga Y, Okamoto T, Nishimori T, Suemune S, Nasution ID, Chen IC, et al. Oral and facial representation in the trigeminal principal and rostral spinal nuclei of the cat. J Comp Neurol 1986;244:1-18.
- Smith RL. Axonal projections and connections of the principal sensory trigeminal nucleus in the monkey. J Comp Neurol 1975;163:347-75.
- Takemura M, Sugimoto T, Shigenaga Y. Difference in central projection of primary afferents innervating facial and intraoral structures in the rat. Exp Neurol 1991;111:324-31.
- Bae YC, Ihn HJ, Park MJ, Ottersen O, Moritani M, Yoshida A, et al. Identification of signal substances in synapses made between primary afferents and their associated axon terminals in the rat trigeminal sensory nuclei. J Comp Neurol 2000;418:299-309.
- Clements JR, Beitz AJ. An electron microscopic description of glutamate-like immunoreactive axon terminals in the rat principal sensory and spinal trigeminal nuclei. J Comp Neurol 1991;309:271-80.
- Magnusson KR, Clements JR, Larson AA, Madi JE, Beitz AJ. Localization of glutamate in trigeminothalamic projection neurons: a combined retrograde transport-histochemical study. Somatosens Res 1987;4:177-90.
- Kaneko T, Itoh K, Shigemoto R, Mizuno N. Glutaminase-like immunoreactivity in the lower brainstem and cerebellum of the adult rat. Neuroscience 1989;32:79-98.
- Kaneko T, Mizumo N. Immunohistochemical study of glutaminase-containing neurons in the cerebral cortex and thalamus of the rat. J Comp Neurol 1988;267:590-602.
- Bellocchio EE, Reimer RJ, Fremeau RT, Edwards RH. Uptake of glutamate into synaptic vesicles by an inorganic phosphate transporter. Science 2000;289:957-60.
- Fremeau RT, Troyer MD, Pahner I, Nygaard G, Tran CH, Reimer RJ, et al. The expression of vesicular glutamate transporters defines two classes of excitatory synapse. Neuron 2001;31:247-60.
- Fremeau RT, Voglmaier S, Seal RP, Edwards RH. VGLUTs define subsets of excitatory neurons and suggest novel roles for glutamate. Trends Neurosci 2004;27:98-103.
- Gras C, Herzog E, Bellenchi GC, Bernard V, Ravassard P, Pohl M, et al. A third vesicular glutamate transporter expressed by cholinergic and serotoninergic neurons. J Neurosci 2002;22:5442-51.
- Varoqui H, Schäfer MKH, Zhu HM, Weihe E, Erickson JD. Identification of the differentiation-associated Na+/Pi transporter as a novel vesicular glutamate transporter expressed in a distinct set of glutamatergic synapses. J Neurosci 2002;22:142-55.
- Li JL, Kaneko T, Nomura S, Li YQ, Mizuno N. Association of serotonin-like immunoreactive axons with nociceptive projection neurons in the caudal spinal trigeminal nucleus of the rat. J Comp Neurol 1997;384:127-41.
- Kaneko T, Urade Y, Watanabe Y, Mizuno N. Production, characterization, and immunohistochemical application of monoclonal antibodies to glutaminase purified from rat brain. J Neurosci 1987;7:302-9.
- Fujiiyama F, Furuta T, Kaneko T. Immunocytochemical localization of candidates for vesicular glutamate transporters in the rat cerebral cortex. J Comp Neurol 2001;435:379-87.
- Li YQ, Wang ZM, Zheng HX, Shi JW. Central origins of substance P-like immunoreactive fibers and terminals in the spinal trigeminal caudal subnucleus in the rat. Brain Res 1996;719:219-24.