Internalization and distribution of three α1-adrenoceptor subtypes in HEK293A cells before and after agonist stimulation1
Introduction
α1-adrenoceptors (α1-AR) are Gq/11-coupled receptors that respond to the neurotransmitters and hormones norepinephrine and epinephrine to mediate physiological effects such as prostate smooth muscle contraction and myocardial hypertrophy by activating phospholipase C and generating second messengers that release stored intracellular Ca2+ and stimulate protein kinase C[1,2]. α1-AR are also involved in the regulation of growth-promoting responses via the mitogen-activated protein kinase family[3].
Three human α1-AR subtypes have been cloned: α1A-, α1B-, and 1D-AR. Although α1-AR family members are highly homologous (eg 75% amino acid identity in transmembrane domains), they share little homology at their amino and carboxyl termini[3,4], suggesting that α1-AR subtypes have different expression, function, and subcellular distribution.
α1-AR expression was found in many human tissues such as the brain, heart, and vascular smooth muscles[5]. Several reports have shown that α1B-AR was mainly localized on the cell surface[6–9], and agonists induced its phosphorylation and internalization. G-protein-coupled receptors (GPCR), kinases 2 and 3, seem to be involved in the phosphorylation of agonist-bound α1B-AR during homologous desensitization[10,11]. The phosphorylation sites involved have been located at Ser404, Ser408, and Ser440 in the receptor C terminus[11]. Several researchers found that α1A-AR was mainly located on the cell surface and the intracellular compart-ments[9]. However, α1D-AR was mainly localized in the intracellular compartments[12], but could be induced to translocate to the cell surface by co-expressed α1B-AR[13]. Unfortunately, the molecular determinants of desensitization and internalization for α1A-AR and α1D-AR were largely unknown. Vazquez-Prado et al[14] showed that α1A-AR could undergo agonist-mediated phosphorylation, but not to the same extent as α1B-AR. Yang and colleagues[15] stably transfected fibroblasts with each α1-AR and observed that increases in inositol phosphates mediated by α1A and α1B-AR could be desensitized, whereas the increase mediated by α1D-AR was refractory to agonist-mediated desensitization. In contrast, Garcia-Sainz et al[16] found that α1D-AR could be phosphorylated and desensitized. Recent reports showed that cell trafficking (desensitization and redistribution) was an important step in the regulation of GPCR, particularly in response to receptor stimulation by agonists. The ultimate effect on receptor signaling and the fate of sequestered receptors varies with receptor type, duration of agonist exposure, and cellular environment[17]. In this study, we examined subcellular distribution of the 3 α1-AR subtypes and their internalization and trafficking upon agonist stimulation in human embryonic kidney (HEK) 293A cells. We also compared the distribution and trafficking property between total receptors and functional receptors.
Materials and methods
Flag-tagged human α1A-, α1B-, and α1D-AR were gifts from Prof KP MINNEMAN (Emory University, Atlanta, USA). Dulbecco’s modified Eagle’s medium (DMEM) and fetal bovine serum (FBS) were obtained from Hyclone (Rockville, MD, USA). Geneticin, an anti-flag antibody, and phenylephrine were obtained from Sigma-Aldrich (St Louis, MO, USA). [125I]-BE2254 and [3H]-prazosin were obtained from Amersham Biosciences (Buckinghamshire, UK). α1-AR green fluorescent protein (GFP) vectors were constructed by ligating the coding region of human α1A-,α1B-, and α1D-AR into the EcoR I–BamH I site of the basic pEGFP-C2 protein fusion vector (Clontech, USA) as described previously[7].
Cell culture and transfection HEK293A cells were cultured in DMEM with sodium pyruvate supplemented with 10% FBS, 100 U/mL streptomycin, and 100 U/mL penicillin in a humidified atmosphere with 5% CO2. Cells for transient transfection were grown in culture dishes with a glass coverslip. When grown to approximately 60% confluence, cells were transfected with 5 μg cDNA encoding α1A-, α1B-, α1D-AR/GFP fusion protein using Lipofectamine 2000 (Invitrogen, Carlsbad, CA, USA), respectively. Cell lines stably transfected with α1A-, α1B-, and α1D-AR/Flag, which were cloned and established before[18], were grown on 6-well plates and maintained with geneticin (200 mg/mL). As previously described, the Bmax of α1A-AR/Flag, α1B-AR/Flag, and α1D-AR/Flag were 4.8, 20.8, and 0.24 pmol/mg protein, respectively, on cell membranes as determined by [125I]-BE2254 binding assay[18].
Western blot assay HEK293A cells transiently expressing α1A-, α1B-, or α1D-AR were treated with phenylephrine (10 µmol/L) or prazosin and phenylephrine or vehicle. Cells were lysed with ice in cold lysis buffer containing 20 mmol/L Tris-HCl (pH 7.4), 150 mmol/L NaCl, 2.5 mmol/L edetic acid, 50 mmol/L NaF, 0.1 mmol/L Na4P2O7, 1 mmol/L Na3VO4, 1% TritonX-100, 10% glycerol, 0.1% SDS, 1% deoxycholic acid, 1 mmol/L phenylmethylsulfonyl fluoride, and 1 mg/mL aprotinin for 20 min, sonicated for 20 s, and centrifuged at 12 870×g for 15 min. The supernatant is the whole cell protein. The lysate [30 µg, extracellular signal-related kinase (ERK) assay] was separated by electrophoresis using 10% SDS-PAGE and transferred onto nitrocellulose membrane or polyvinylidene difluoride membrane. Nonspecific IgG was blocked with 5% fat-free milk, and the membrane was incubated with the antibody to phospho-ERK (Cell Signaling Technology, Inc, Beverly, MA, USA); horseradish peroxidase-conjugated anti-mouse IgG was used as a second antibody (Jackson ImmunoResearch Laboratories, West Grove, PA, USA). The peroxidase reaction products were visualized by LumiGLO Chemiluminescent Substrate (New England Biolabs, Beverly, MA, USA). The same membrane was then stripped and reprobed with anti-ERK to determine the total protein.
Confocal microscopy and image quantification HEK293A cells transiently transfected with GFP-tagged construct were grown on sterile coverslips, fixed for 30 min with 4% paraformaldehyde in 0.1 mol/L phosphate buffer, pH 7.4, and rinsed 3 times with PBS. Cells were imaged with a Spectra-Physics laser scanning confocal microscope with a Plan-Apo 40× oil immersion objective lens (Leica, Wetzlar, Germany). The software used to collect the images was the Leica TCS NT, version 1.6.587. The images were transferred to a computer and analyzed with Adobe Photoshop version 5.0 (Adobe Systems, Mountain View, CA, USA). The setting on the laser was constant for all experiments. Fluorescence was excited using an argon laser at a wavelength of 488 nm, and the absorbed wavelength was detected at 510–520 nm. The extent to which the α1-AR/GFP was internalized after exposure to agonists was quantified by the intracellular immunofluorescence intensity. The extent of α1-AR/GFP receptor internalization was defined as the rate of the fluorescence intensity before and after exposure to agonists.
Internalization assay The HEK293A cells stably expressing α1-AR were seeded at a density of 2×105/well onto poly-L-lysine-coated 24-well plates. The next day, the cells were pre-incubated with 1 µg of mouse anti-flag antibody for 1 h in DMEM free of serum at 37 °C and washed twice using serum-free DMEM. Cells were then treated with 10 µmol/L phenylephrine for different time periods in the serum-free DMEM. Subsequently, the cells were fixed and incubated with peroxidase-conjugated anti-mouse antibody (1:1000, Santa Cruz, CA, USA) for 2 h at room temperature. After washing, the plates were developed with 250 µL of o-phenylenediamine dihydrochloride (OPD) solution (4 mg OPD in 10 mL PBS, 15 µL 30% H2O2). After 10–30 min, 200 µL of the substrate solution from each well was transferred to 96-well plates and analyzed at 490 nm using a microplate reader (Bio-Rad, Hercules, CA, USA).
Whole cell binding assay To further determine the subcellular distribution of the 3 α1-AR subtypes prior to, or after incubation with phenylephrine for 1 h, radioligand binding was performed on whole cells stably transfected with the flag-tagged 3 α1-AR constructs as described by Eason MG et al[19]. The cells were re-suspended in ice-cold DMEM medium, then incubated with increasing concentrations (0.01–2 nmol/L) of [3H]-prazosin alone, or in the presence of the lipophilic competitor, phentolamine (10 µmol/L), or in the presence of the hydrophilic competitor, adrenaline (100 mmol/L), for 4 h at 4 °C. With the intact cells, specific binding, as defined with phentolamine, represented the total cellular amount of α1-AR, because it is able to penetrate the plasma membrane and scatter about the whole cell, whereas specific binding, as defined with adrenaline, represents only cell surface receptors owing to its hydrophilic properties, that is, adrenaline is unable to penetrate the plasma membrane, especially at 4 °C. The intracellular pool of receptors is defined as the total number of receptors minus those on the cell surface. Saturation and displacement curves were analyzed by non-linear regression analysis with Prism (GraphPad Software, San Diego, CA, USA).
Statistical analysis Data from confocal quantity were analyzed using the unpaired t-test with statistical significance defined as P<0.05. Data from Western and internalization assays were analyzed by GraphPad Prism using the 2-tailed paired t-test. Significant differences were defined as P<0.05.
Results
ERK 1/2 were activated by phenylephrine in the HEK293A cells transiently transfected with α1-AR/GFP fusion protein To demonstrate that the α1-AR/GFP fusion proteins expressed in HEK293A cells were functional, the HEK293A cells transfected with α1A-, α1B-, and α1D-AR/GFP, respectively, were treated with phenylephrine, and the phosphorylation of ERK 1/2 was measured by Western blot. The levels of the phospho-ERK1/2 in HEK293A cells transfected with α1A-, α1B-, or α1D-AR/GFP significantly increased after treatment with phenylephrine (PE, 10 µmol/L), an agonist of α1-AR, for 30 min. Pretreatment with prazosin (1 µmol/L), an antagonist of α1-AR, however, greatly attenuated the effects of phenylephrine on the activation of ERK1/2 in the transfected HEK293A cells. These results suggested that the α1-AR/GFP fusion proteins expressed in the HEK293A cells were biologically active (Figure 1).
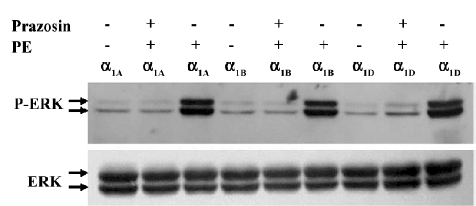
Agonist-induced localization changes of α1-AR subtypes in HEK293A cells By real-time confocal microscopy, we found that the incubation of HEK293A cells transiently expressing α1A- or α1B-AR/GFP with 10 µmol/L phenylephrine caused changes of receptor localization (Figures 2, 3). An accumulation of α1-AR in vesicle-like structures was found in the cytoplasm, and the membrane receptors became uneven. Because coated vesicles are the prelude of GPCR internalization by agonist stimulation, these results suggested that agonist stimulation could induce α1A- and α1B-AR internalization. The receptor localization in cells expressing α1D-AR, however, was unchanged after phenylephrine stimulation (Figure 4).
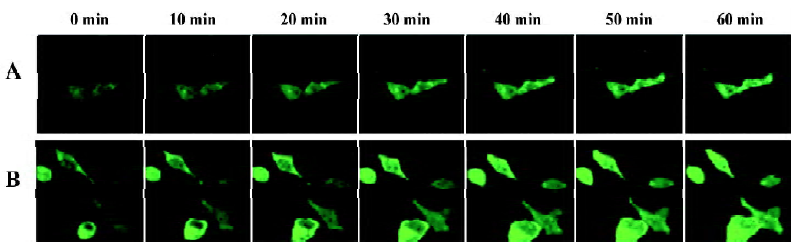
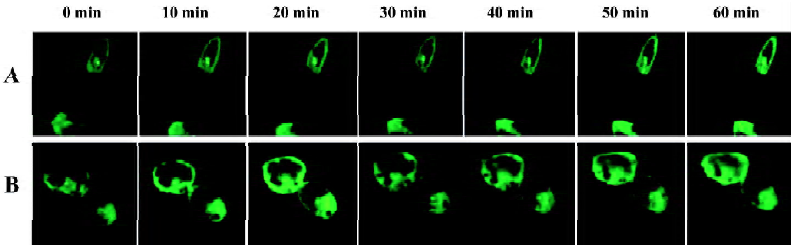
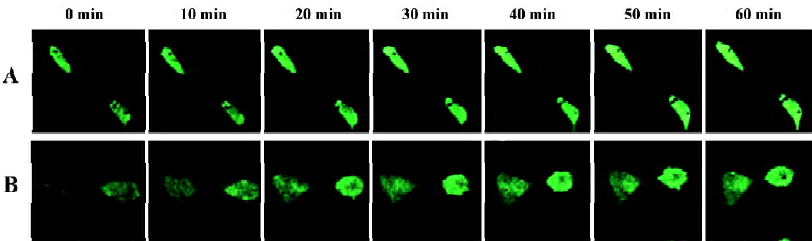
We then measured the signal intensity of intracellular fluorescence to estimate the rate of receptor internalization. The fluorescent intensity of α1A- and α1B-AR was significantly increased in the cytoplasm after phenylephrine stimu-lation, and α1B-AR occurred in a very rapid manner (20 min after phenylephrine stimulation, Figure 5A), while α1A-AR occurred in a slower manner (25 min, Figure 5B). HEK293A cells expressing α1D-AR, however, showed no translocation of the receptors on the cell surface after phenylephrine stimulation (Figure 5C). These results indicated that the location changes of the receptors after phenylephrine stimulation were due to receptor internalization.
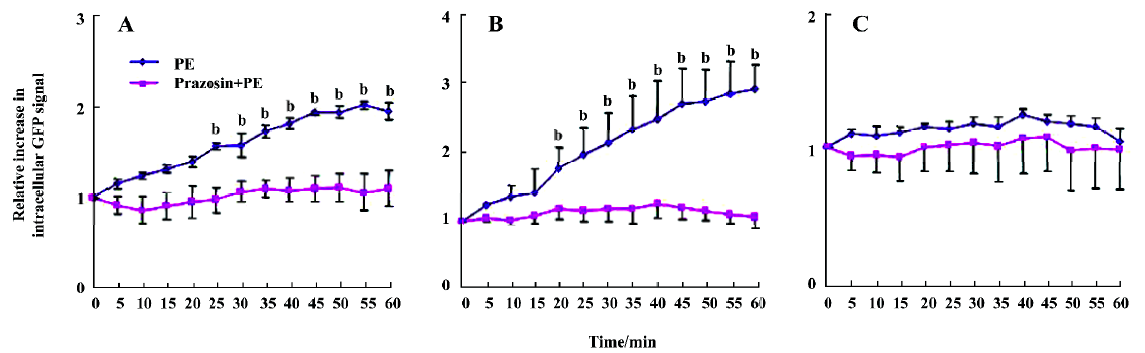
Pretreatment with prazosin prior to phenylephrine stimulation inhibited α1A- and α1B-AR translocation and decreased the fluorescent intensity of cytoplasmic receptors in the transfected HEK293A cells (Figure 5), suggesting that phenylephrine acted directly on α1A- and α1B-AR to induce receptor translocation, a phenomenon called homologous desensitization or internalization.
α1-AR internalization induced by phenylephrine in HEK293A cells We next examined the effects of phenylephrine stimulation on cell surface receptor internalization using HEK293A cells stably expressing α1A-, α1B-, or α1D-AR by intact cell enzyme-linked immunosorbent assay (ELISA). Five minutes after phenylephrine stimulation, the amounts of α1B-, α1A-, and α1D-AR on the cell surface decreased by about 29.2%, 9.7%, and 11.3%, respectively. One hour after the stimulation, they further decreased by about 64.8%, 36.4%, and 32.1%. These results indicated that phenylephrine could induce the internalization of α1A, α1B, and α1D-AR, and the internalization of α1B-AR occurred faster than that of α1A- and α1D-AR (Figure 6).
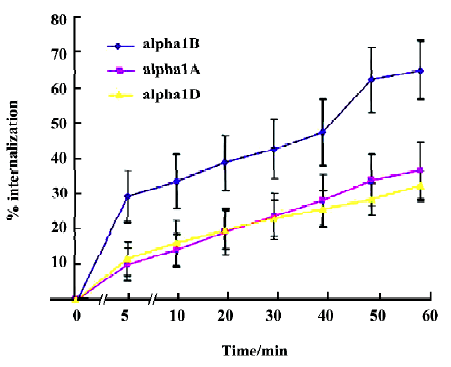
Agonist-induced distribution changes in binding of functional α1-AR subtypes in HEK293A cells To test whether membrane and cytoplasm functional receptors changed under phenylephrine stimulation, whole cell [3H]-prazosin binding assay was used. Functional α1B-AR was mainly expressed on the surface of transfected HEK293A cells, which was about 92.4% of the total receptor. α1D-AR was mainly expressed in the cytoplasm, about 69.5% of total receptor, and α1A-AR was expressed both on the cell surface and in the cytoplasm, which was consistent with the results of the confocal analysis. One hour after phenylephrine stimulation, functional α1D-AR did not change compared with the control. α1A- and α1B-AR on the cell surface, however, decreased significantly: α1A-AR from 53.7% to 35.5% of the total receptor, and α1B-AR from 92.4% to 30.2% of the total receptor, which suggested that phenylephrine stimulation could induce the internalization of functional α1A-AR and α1B-AR proteins (Table 1).
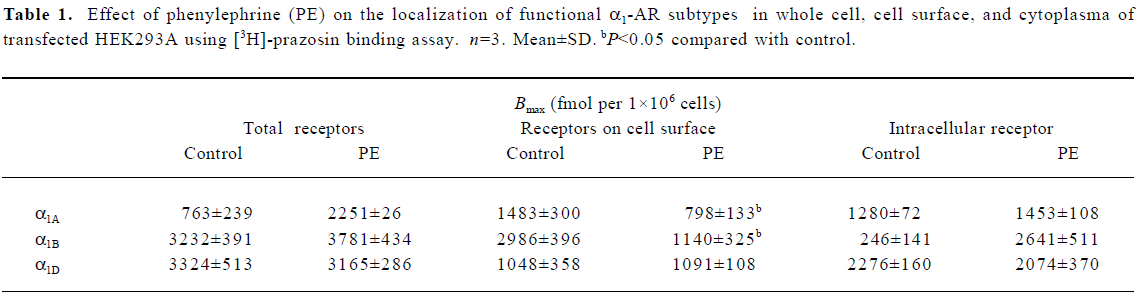
Full table
Discussion
In this report, we investigated the trafficking profile of the 3 α1-AR. We created a fusion construct consisting of N-terminal GFP-tagged α1-AR and transfected this construct into HEK293A cells, which allowed us not only to identify the 3 subtype receptors by confocal microscopy, but also to compare the surface and total receptor by intact cell ELISA and [3H]-prazosin binding assay before and after phenylephrine stimulation.
As described in the Methods and in our previous work, the Bmax of α1B-AR on the membrane was found to be the highest, followed by α1A-AR and α1D-AR, respectively[18]. These results are consistent with the previous report in which the functional binding of wild type α1-AR was examined[20].
Previous studies with the α1B-AR/GFP construct demonstrated that it was fully functional and internalized in the same manner as a non-GFP tagged α1B-AR construct[21]. Both the α1A- and α1B-AR were associated with the activation of ERK[22]. In this study, all the α1A-, α1B-, and α1D-AR, when coupled to GFP, promoted the increase in ERK1/2 phosphorylation, suggesting that the α1-AR were functional and retained their ability to activate cellular signaling when conjugated to the GFP.
Cellular localization and trafficking is very important for α1-AR to accomplish their physiological functions. Results obtained over the past years have shown that there is a significant difference in the subcellular distribution among the α1-AR subtypes. Fonseca et al[6] observed that α1B-AR was expressed predominantly on the cell membrane and α1A-AR was expressed both on the membrane and cytoplasm in the stably-transfected fibroblasts by immunocytochemistry. This was confirmed by Awaji et al[21] who used α1B-AR/GFP fusion proteins to verify cell membrane localization of α1B-AR in COS-7 cells. McGrath et al[23] used BODIPY-FL-labeled prazosin to image α1-AR subtypes in cultured prostate smooth muscle cells and fibroblasts stably transfected with each subtype and found that the 3 α1-AR subtypes were expressed in the cytoplasm of fibroblasts. Very little α1D-AR expression was detected on the cell surface. In this study, we transfected HEK293A cells with cDNA encoding α1-AR/GFP. Living cells were then visualized by real-time laser scanning confocal microscopy. We found that α1B-AR/GFP fluorescence was predominant on the cell surface, whereas the α1A-AR expression was detected both on the cell surface and in the cytoplasm. α1D-AR was mainly localized in intracellular compartments, suggesting that the localization of the 3 α1-AR subtypes was different in the transfected HEK293A cells.
α1-AR are subject to dynamic regulation by a variety of mechanisms, including phosphorylation, protein-protein interactions, protein trafficking, and transcription. One of the most intensive studies of these mechanisms is internalization and desensitization, a general phenomenon in which the intensity of a biological response wanes over time, despite continued stimulus. Desensitization can be further characterized as either homologous, where receptor response wanes upon continuous exposure to its agonist, or hetero-logous, where agonist-mediated stimulation of a receptor can attenuate the response by other receptors mediating similar cellular events[24]. With regard to the α1-AR subtypes, the desensitization, downregulation, and internalization characteristics of the α1B-AR have been extensively examined. For example, agonist-mediated phosphorylation and internalization of the α1B-AR have been demonstrated, and the domains of the receptors involved in internalization have been identified[6,10,11]. We know much less about the molecular determinants of desensitization, downregulation, and internalization for α1A- and α1D-AR. Here, we transfected HEK293A cells with cDNA encoding α-AR/GFP. Living cells were then visualized by real time laser scanning confocal microscopy under phenylephrine stimulation. Stimulation of α1-AR with phenylephrine induced the changes of localization of α1A-AR and α1B-AR, but not the localization of α1D-AR.
Confocal image quantification analysis found that stimulation of α1B-AR with phenylephrine promoted a rapid internalization of α1B-AR, which began at about 20 min, and a slower internalization of α1A-AR, which began at about 25 min. However, α1D-AR internalization did not occur after phenylephrine stimulation. We only assayed the changes of fluorescence intensity of the plasma, but α1D-AR was mainly localized in intracellular compartments. Therefore, this method can not determine whether α1D-AR was internalized under phenylephrine stimulation.
To confirm the results of confocal microscopy, the internalization of membrane receptors was detected by intact cell ELISA. This does not change the permeability of cell membrane, so the antibodies to α1-AR subtypes only bind to the membrane surface of stably-transfected HEK293A cells. The internalization percentage of α1A-AR was about 9.7% at 5 min and about 36.4% at 1 h after phenylephrine stimulation. The internalization percentage of α1B-AR was about 29.2% at 5 min and about 64.8% at 1 h after phenylephrine stimulation. Intact cell ELISA assay also demonstrated α1D-AR internalization after phenylephrine stimulation. The internalization percentage of α1D-AR was about 11.3% at 5 min and about 32.1% at 1 h after phenylephrine stimulation. Although Garcia-Sainz et al[22] showed that α1D-AR could be phosphorylated and internalized, many other studies reported that α1D-AR could not be internalized under agonist stimulation. Our results by ELISA are the same as Garcia-Sainz et al. As mentioned earlier, cell-intact ELISA only detects the receptors located on the outside surface of the membrane, but confocal imaging analysis mainly assesses the changes of fluorescence intensity of the plasma. When internalization occurs, α1D-AR may just move from the outside to the inside of the membrane. Although some α1D-AR may translocate from the membrane to the plasma, the internalized α1D-AR are negligible compared with the total α1D-AR because most α1D-AR are expressed in the plasma. This may explain why α1D-AR internalization can be detected by ELISA rather than confocal imaging analysis. However, further studies are needed to elucidate this discrepancy.
The confocal imaging and ELISA analyses demonstrated the localization changes and internalization of the 3 α1-AR subtypes. Receptor proteins include receptors with binding activity and receptors without binding activity. Past studies generally did not differentiate these 2 categories. In this study, we detected the Bmax of the total cell surface and intracellular functional α1-AR by whole cell [3H]-prazosin binding assay. We found that the total Bmax were the same in the 3 subtypes, and not different before and after phenylephrine stimulation. α1A functional receptors were not only on the cell surface, but also in intracellular compartments. α1B functional receptors were predominantly on the cell surface; α1D functional receptors were mainly in intracellular compart-ments. In general, AR are G-protein-coupled membrane receptors. From the previous point of view, only the receptors on the membrane have the binding function, whereas the receptors in the cytoplasm are immature and do not have binding ability. However, the present study demonstrated that there were functional receptors in the cytoplasm. Stimulation of α1A- and α1B-AR with phenylephrine for 1 h promoted internalization of α1A- and α1B-AR, but not α1D-AR. This apparent discrepancy in α1D-AR internalization, as determined by different methods, may be partly explained by the different observed parameters. Both confocal imaging and internalization assay determine receptor protein, whereas whole cell binding assay determines functional receptors. Since only a few α1D-AR are located on the cell surface, in order to maintain the reactivity as strongly as possible, most of them are probably functional receptors. On the contrary, since both α1A- and α1B-AR on the cell surface are quite abundant, the amount of their functional receptors would be far more than that of α1D-AR, even though their functional receptors may only account for a small fraction of the whole receptors. It is supposed that when internalization occurs, there would be as many mature functional α1D-AR as possible sorted on the cell surface to complement the internalized ones in order to maintain the maximal reactivity. However, even if quite a lot of functional α1A- or α1B-AR were internalized upon phenylephrine stimulation, the residual functional receptors on the cell surface would be enough to maintain the same reactivity as before, so it would not be necessary to sort the mature functional receptors on the cell membrane. Therefore, internalization could only be observed on α1A- and α1B-AR, but not α1D-AR by confocal imaging. However, the exact mechanism remains to be explored.
These results also explain why the total amount of the 3 α1-AR subtypes was equal by whole cell [3H]-prazosin binding assay, while their densities on the membrane were quite different when detected by traditional [125I]-BE2254 binding assay. Because [125I]-BE2254 binding assay only reflected the receptors on the membrane, a part of the whole cell receptors, [3H]-prazosin binding assay reflected the total amount of receptors, including both receptors on the cell membrane and those in the cytoplasm. The discrepancy between the 2 methods offers evidence that the 3 α1-AR subtypes have different distribution, localization, and trafficking characteristics, which is consistent with that examined by other methods in this study.
In summary, in transfected HEK293A cells, α1A-AR evenly distribute in the cytoplasm and membranes, but phenylephrine stimulation causes their internalization. α1B-AR are mainly located on the membrane, however, phenylephrine can result in rapid internalization, and the number of internalized α1B-AR is more than α1A-AR. α1D-AR predominantly exist in the cytoplasm, and their internalization upon phenylephrine stimulation could be detected only by intact-cell ELISA, but not by other methods. Therefore, a definitive answer regarding the internalization characteristics of α1D-AR requires additional study. Furthermore, we compared the distribution between functional receptors and total receptor proteins. Because there were functional receptors in the cytoplasm, we suggested that the receptors in the plasma act as a receptor’s storage pool.
References
- Milligan G. Constitutive activity and inverse agonists of G protein-coupled receptors: a current perspective. Mol Pharmacol 2003;64:1271-6.
- Graham RM, Perez DM, Hwa J, Piascik MT. α1-Adrenergic receptor subtypes. Molecular structure, function and signaling. Circ Res 1996;78:737-49.
- Zhong H, Minneman KP. Differential activation of mitogen-activated protein kinase pathways in PC12 cells by closely related alpha1-adrenergic receptor subtypes. J Neurochem 1999;72:2388-96.
- Schwinn DA, Johnston GI, Page SO, Mosley MJ, Wilson KH, Worman NP, et al. Cloning and pharmacological characterization of human α1-adrenergic receptors: sequence corrections and direct comparison with other species homologues. J Pharmacol Exp Ther 1995;272:134-42.
- Rokosh DG, Stewart AF, Chang KC, Bailey BA, Karliner JS, Camacho SA, et al. α1-Adrenergic receptor subtype mRNAs are differentially regulated by α1-adrenergic and other hypertrophic stimuli in cardiac myocytes in culture and in vivo. J Biol Chem 1996;271:5839-43.
- Fonseca MI, Button DC, Brown RD. Agonist regulation of α1B-adrenergic receptor subcellular distribution and function. J Biol Chem 1995;270:8902-9.
- Hirasawa A, Sugawara T, Awaji T, Tsumaya K, Ito H, Tsujimoto G. Subtype-specific differences in subcellular localization of α1-adrenoceptors (ARs): chloroethylclonidine preferentially alkylates the accessible surface α1-ARs irrespective of subtype. Mol Pharmacol 1997;52:764-70.
- Hrometz SL, Edelmann SE, McCune DF, Olges JR, Hadley RW, Perez DM, et al. Expression of multiple alpha1-adrenergic receptors on vascular smooth muscle: correlation with the regulation of contraction. J Pharmacol Exp Ther 1999;290:452-63.
- Sugawara T, Hirasawa A, Hashimoto K, Tsujimoto G. Differences in the subcellular localization of alpha1-adrenoceptor subtypes can affect the subtype selectivity of drugs in a study with the fluorescent ligand BODIPY FL-prazosin. Life Sci 2002;70:2113-24.
- Diviani D, Lattion AL, Larbi N, Kunapuli P, Pronin A, Benovic JL, et al. Effect of different G protein-coupled receptor kinases on phosphorylation and desensitization of the alpha1B-adrenergic receptor. J Biol Chem 1996;271:5049-58.
- Diviani D, Lattion AL, Cotecchia S. Characterization of the phosphorylation sites involved in G protein-coupled receptor kinase- and protein kinase C-mediated desensitization of the alpha1B-adrenergic receptor. J Biol Chem 1997;272:28712-9.
- Chalothorn D, McCune DF, Edelmann SE, Garcia-Cazarin ML, Tsujimoto G, Piascik MT. Differences in the cellular localization and agonist-mediated internalization properties of the α1-adrenoceptor subtypes. Mol Pharmacol 2002;61:1008-16.
- Hague C, Uberti MA, Chen Z, Hall RA, Minneman KP. Cell surface expression of α1D-adrenergic receptors is controlled by heterodimerization with α1B-adrenergic receptors. J Biol Chem 2004; 279: 15 541–9.
- Vazquez-Prado J, Medina LC, Romero-Avila MT, Gonzalez-Espinosa C, Garcia-Sainz JA. Norepinephrine and phorbol ester-induced phosphorylation of α(1a)-adrenergic receptor. J Biol Chem 2000;275:6553-9.
- Yang M, Ruan J, Voller M, Schalken J, Michel MC. Differential regulation of human α1-adrenoceptor subtypes. Naunyn-Schmiedeberg's Arch Pharmacol 1999;359:439-46.
- Garcia-Sainz JA, Vazquez-Cuevas FG, Romero-Avila MT. Phosphorylation and desensitization of α1D-adrenergic receptors. Biochem J 2001;353:603-10.
- Ferguson SS. Evolving concepts in G protein-coupled receptor endocytosis: the role in receptor desensitization and signaling. Pharmacol Rev 2001;53:1-24.
- Wang S, Song Y, Xu M, Hao T, Han Q, Zhang Y. Redistribution of three α1-adrenergic receptor subtypes in the stably transfected HEK 293A cells upon agonist stimulation. Acta Physiol Sin 2004;57:480-5.
- Eason MG, Liggett SB. Subtype-selective desensitization of alpha 2-adrenergic receptors. Different mechanisms control short and long term agonist-promoted desensitization of alpha 2C10, alpha 2C4, and alpha 2C2. J Biol Chem 1992; 267: 25 473–9.
- Minneman KP, Esbenshade TA. Alpha 1-adrenergic receptor subtypes. Annu Rev Pharmacol Toxicol 1994;34:117-33.
- Awaji T, Hirasawa A, Kataoka M, Shinoura H, Nakayama Y, Sugawara T, et al. Real-time optical monitoring of ligand-mediated internalization of α1b-adrenoceptor with green fluorescent protein. Mol Endocrinol 1998;12:1099-111.
- Garcia-Sainz JA, Vazquez-Prado J, Villalobos-Molina R. α1-adrenoceptors: subtypes, signaling and roles in health and disease. Arch Med Res 1999;31:449-58.
- McGrath JC, Mackenzie JF, Daly CJ. Pharmacological implications of cellular localization of alpha1-adrenoceptors in native smooth muscle cells. J Auton Pharmacol 1999;19:303-10.
- Bunemann M, Lee KB, Pals-Rylaarsdam R, Roseberry AG, Hosey MM. Desensitization of G-protein-coupled receptors in the cardiovascular system. Annu Rev Physiol 1999;61:169-92.