Mycophenolic acid inhibits SLE-associated cytokine expression and promotes apoptosis of peripheral blood mononuclear cells from patients with systemic lupus erythematosus1
Introduction
Systemic lupus erythematosus (SLE) is a chronic autoimmune disease, characterized by the over-activation of self-reactive T and B lymphocytes and mass production of auto-antibodies. Abnormal immune and inflammatory responses account for most clinical manifestations of SLE patients, though the mechanism of SLE still remains to be elucidated. In recent years, accumulated evidence demonstrated that defects in apoptosis and/or the removal of apoptotic substance can be the key points that initiate SLE[1]. Bengtsson et al confirmed that SLE serum had a strong and apparently disease-specific apoptosis-inducing capacity, which could contribute to a high load of potential autoantigen[2]. The high percentage of apoptotic T cells in SLE patients may be related to the high levels of IL-10 in SLE serum, as IL-10 may induce the abnormally activated T cells to trigger apoptosis via the Fas-FasL pathway[3]. Inflammatory cytokines such as TNF-α, IL-6, IL-12, and IFN are increased in SLE serum. These cytokines play an important role in inflammation and tissue damage. The serum levels of IL-12 and IFN-γ were found to be elevated and to be correlating with disease activity in SLE[4,5].
The current therapy for SLE is mainly a combined application of immunosuppressants based on corticosteroids. Prednisone has long been the cornerstone of lupus therapy. Other immunosuppressive agents, such as cyclophosphamide, methotrexate, and azathioprine, have serious side-effects. Amongst these side-effects, of particular note are cytopenias, effects on fertility and an increased risk of infection and malignancy with cyclophosphamide; hypertension and nephrotoxicity with cyclosporin; and hypertension, impaired glucose tolerance, obesity and osteoporosis with corticosteroids[6]. There is an urgent need for effective immunosuppressive agents with more favorable side-effect profiles. Several lines of evidence now indicate that mycophenolate mofetil (MMF) exerts a beneficial effect in SLE. After years of clinical verification of its high efficacy and low side-effects, as compared with other immunosuppressive drugs, MMF was recommended as a first-line drug for treating SLE by the American Committee of Rheumatism in October, 2003. Mycophenolic acid (MPA), the active metabolite of MMF, non-competitively and reversibly inhibits inosine monophosphate dehydrogenase (IMPDH), which catalyses a rate-limiting step in the de novo synthesis pathway of purine nucleotides. Unlike other eukaryotic cells, lymphocytes are dependent on the de novo pathway and hence MPA has a relatively specific effect on lymphocytes. MPA affects several crucial aspects of the inflammatory response and, given the relative specificity of its effect, has little impact on other tissues with high proliferative activity, such as the skin, intestine and bone marrow. But evidence on MPA therapy for SLE at cellular levels is still lacking. On the basis of the advancement of SLE pathogenesis and treatment, our experiment aimed to evaluate the effects of MPA on the cytokine production and the apoptosis of peripheral blood mononuclear cells (PBMC) of SLE patients to provide some laboratory reference for the reasonable prescription of MPA in clinical cases.
Materials and methods
Subjects Forty-one SLE patients, who were out- or in-patients from the hematology, rheumatology, or nephrology department of Huai’an first hospital affiliated to Nanjing Medical University, Jiangsu province, China, from March 2003 to April 2004, provided blood samples. All patients were in line with the SLE diagnostic standards revised by the American Rheumatism Association in 1992. Among the patients recruited, 9 were newly-onset who had received no drug treatment before admission, and others were returning patients with a relapse of disease and had ceased any drug treatment at least one week before blood donation. All patients were female, aged from 14 to 56 (35±12) years old. Twenty-two healthy controls were sex- and age-comparable, all female and aged from 23 to 42 (32±7) years old. All of the donors gave informed consent.
Reagents RPMI(Roswell Park Memorial Institute) medium 1640 is a GIBCO BRL (NY, USA) product and Ficoll-Hypaque was obtained from Tianjing Hematology Research Institute of China. The mitogenic lectin phytohaemagglutinin (PHA) was purchased from Guangzhou Bio-products Company (Guangzhou, China). MPA and dexamethasone (DEX) were purchased from Sigma (MO, USA). IL-10, IL-12p40/p70, IFN-γ, sFas and sFasL ELISA kit were obtained from Diaclone (Besan, France). GolgiStop reagent (containing monensin) and reagents for cell fixation and permeabilization (IC fixation buffer and permeabilization buffer, respectively) were purchased from e-bioscience Company (CA, USA). The annexin V-FITC kit system for detection of apoptosis was purchased from Beckman Coulter (CA, USA). MPA was dissolved in undiluted dimethyl sulfoxide, and then was further diluted with culture medium to give a final concentration of 10μmol/L. DEX was dissolved in dehydrated alcohol, and was further diluted with physiological saline to the final concentration of 10μmol/L when it was going to be used. PHA was directly dissolved in culture medium to get a final concentration of 10 μg/mL.
Antibodies The following anti-human monoclonal antibodies were used for the detection of Th subsets: anti-CD4- phycoerythrin (PE)/Cy5[mouse immunoglobulin G1 (IgG1)], anti-IFN-γ-fluorescein isothiocyanate (FITC) (mouse IgG1), anti-IL-10- PE (rat IgG1), and appropriate isotype controls were purchased from e-bioscience.
PBMC preparation Heparinized blood 10 mL obtained from each patient and control subjects were collected and diluted (1:1) with culture medium. Diluted blood 10 mL on 5 mL Ficoll-Hypaque was centrifuged at 400×g for 20 min at 20 °C. PBMC were collected at the interface and washed twice with the medium.
Cell culture To measure the cytokines, PBMC isolated from both SLE patients and healthy controls were resuspended in RPMI-1640 medium supplemented with 10% fetal calf serum, 100 U/mL penicillin and 100 mg/mL streptomycin at 2×106 cell/mL, seeded in 24-well flat-bottomed cell culture plate (Nunclon Surface, Denmark), and cultured at 37 °Cin a humidified atmosphere containing 5% CO2. Cells stimulated with or without PHA were treated with MPA (10 μmol/L) or DEX (10 μmol/L) in different wells. To determine the Th subsets, PBMC in some wells were cultured for 24 h, and at the twelfth hour of culture monensin (×1000) was added to inhibit cytokine secretion. At the 24 h, cells were collected by centrifugation at 300×g for 5 min for antibody staining. Cells in other wells were cultured for 48 h and then the cell suspensions were centrifuged at 300×g for 5 min. Supernatants were collected, and stored at –20 °C for future use. Cells were used for analysis of apoptosis.
ELISA IL-10, IL-12p40/p70, IFN-γ, sFas, and sFasL in supernatants of cell culture for 48 h were analyzed with sandwich ELISA, according to the procedures specified by each kit.
Three-color flow cytometry analysis of CD4+ subsets expressing different cytokines At the indicated time of culture (24 h), the cells were harvested and washed once in ice-cold phosphate-buffered saline (PBS), suspended in a small amount of PBS, and distributed (100 μL per tube) to the 12 mm?75 mm polystyrene round-bottom tubes for immuno-labeling. Anti-CD4-PE/Cy5 20 μL were added to each tube (including the isotype control tube), which was then incubated for 20 min at room temperature in the dark. The samples were centrifuged (300×g for 5 min) at room temperature and washed once with 2 mL of staining buffer after the supernatants were aspirated and discarded. Fixation solution 100 μL were added into each tube to fix the cells. The tubes were then vortexed, and incubated in the dark at room temperature for 20 min. After that, each tube was centrifuged for 5 min after 1 mL of permeabilizaton buffer was added, and then the supernatants were aspirated. Cells were resuspended in 100 μL of permeabilizaton buffer and incubated in the dark at room temperature for 5 min. Anti-IFN-γ-FITC and anti-IL-10-PE or IgG1-FITC and IgG1-PE 20 μL were added into each corresponding tube, which was then incubated in the dark at room temperature for 20 min. The cells were washed once again by adding 1 mL of permeabilizaton buffer and centrifuging for 5 min, and the supernatants were aspirated again. Finally, the cell pellets were resuspended in 0.5 mL of staining buffer and run on a flow cytometer to be analyzed.
Samples were analyzed in an EPICS XL flow cytometer (Beckman Coulter, USA) by using SYSTEM II software. List mode data for 5000 events for lymphocytes were acquired. The percentage of CD4 positive cells were evaluated after forward scatter and side scatter gating on lymphocytes. The IFNγ positive or negative, and IL-10 positive or negative subpopulations were further analyzed within CD4 positive lymphocytes. Statistical analysis was carried out using isotype-matched controls as a reference.
Two-color flow cytometry analysis of apoptosis The 10-fold concentrated binding buffer was diluted with distilled water and placed on ice. The 250 μg propidium iodide (PI) was dissolved in 1 mL of diluted binding buffer and placed on ice. Cell samples were washed with ice-cold PBS after centrifugation for 5 min at 500×g at 4 °C. After the supernatant was discarded, the cell pellet was suspended in ice-cold, diluted binding buffer to 5×105‒5×106/mL and then kept on ice. Annexin V-FITC solution 5 μL and 2.5 μL of dissolved PI were added to 100 μL of the cell suspension prepared as above. After being mixed gently, the sample tube was kept on ice and incubated for 10 min in the dark. Finally, 150 μL of ice-cold, diluted binding buffer was added into the cell sample. The cell sample was then analyzed by flow cytometry.
Statistical analysis Differences between groups were evaluated using compared or independent Student’s t-test with SPSS (version 10.0) software. Results are presented as mean±SD. P<0.05 was considered statistically significant.
Results
Effects of MPA and DEX on IL-10, IL-12, and IFN-γ levels in supernatants of cultured PBMC Twenty samples from 20 patients with SLE were utilized. Among them, 12 samples of PBMC were applied to observe the effect of MPA, 8 samples were used to test the effect of DEX in order to make a comparison. Eight normal samples were used as the control. Levels of IL-10, IL-12, and IFN-γ in the supernatants were significantly elevated after the PBMC from SLE patients were cultured for 48 h, in contrast to those from normal controls. MPA could markedly reduce the secretion of the above three cytokines. The inhibitory effects of DEX on the levels of IL-12 and IFN-γ were insignificant. However, DEX promoted the production of IL-10 induced by PHA (Table 1).
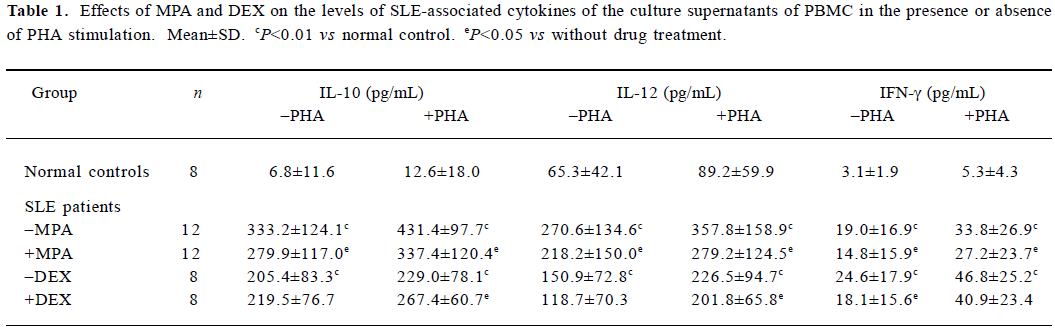
Full table
Effects of MPA and DEX on the percentages of IL-10 or IFN-γ producing CD4+ cells in PBMC Seventeen samples of SLE PBMC and 6 samples of normal control PBMC were used to analyze the change of subsets of IL-10 or IFN-γ producing CD4+ cells. The percentages of CD4+IFN-γ-+I L-10+ and CD4+IFN-γ+IL-10+ subsets in the PBMC cultured for 24 h were significantly increased in SLE, compared with those in normal controls. MPA could decrease the percentages of CD4+IFN-γ-+IL-10+ and CD4+IFN-γ+IL-10+ as well as CD4+IFN-γ+IL-10- subsets (Table 2), according to the records of the experiments with 9 PBMC samples from SLE patients. DEX increased the percentage of CD4+IFN-γ+IL-10+ subset, and reduced the subset of CD4+IFN-γ+IL-10-, especially under PHA stimulation (Table 2), according to another experiment with 8 PBMC samples from SLE.
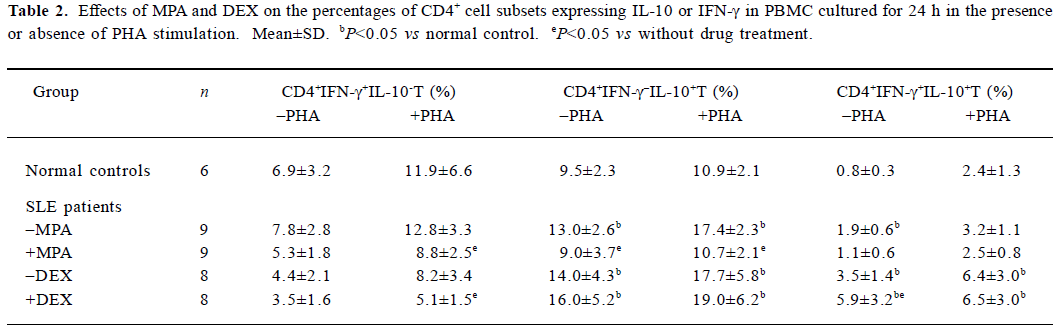
Full table
Effects of MPA and DEX on levels of sFas and sFasL in supernatants of cultured PBMC The culture supernatants of 12 SLE PBMC samples and 8 normal PBMC samples mentioned above were tested for sFas and sFasL levels by sandwich ELISA. The results showed sFasL level of SLE PBMC cultured for 48 h was significantly higher than that of normal PBMC. MPA and DEX could reduce the production of sFas and sFasL by SLE PBMC (Table 3).
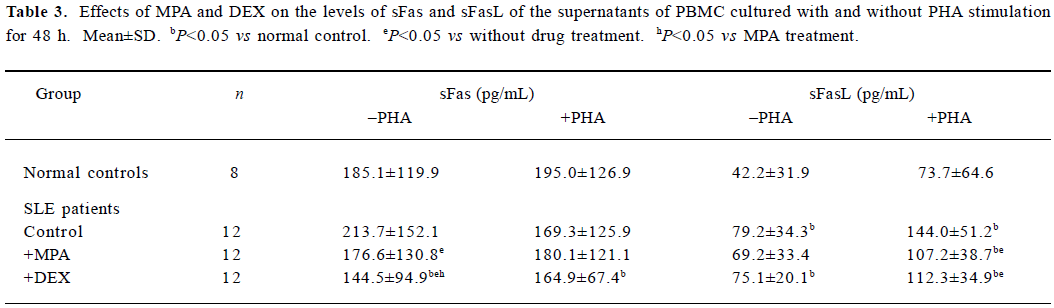
Full table
Effects of MPA and DEX on apoptosis of cultured PBMC Twelve SLE PBMC and 8 normal PBMC from different individuals were used to test the effects of MPA and DEX on apoptosis. Compared with normal controls, the apoptosis of SLE PBMC were significantly increased after being cultured with or without PHA stimulation for 48 h. MPA and DEX could significantly promote apoptosis of SLE PBMC, while DEX showed a much stronger effect in inducing apoptosis of SLE PBMC (Table 4, Figure 1).
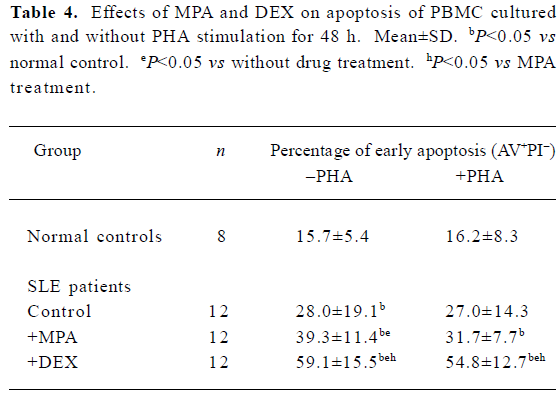
Full table
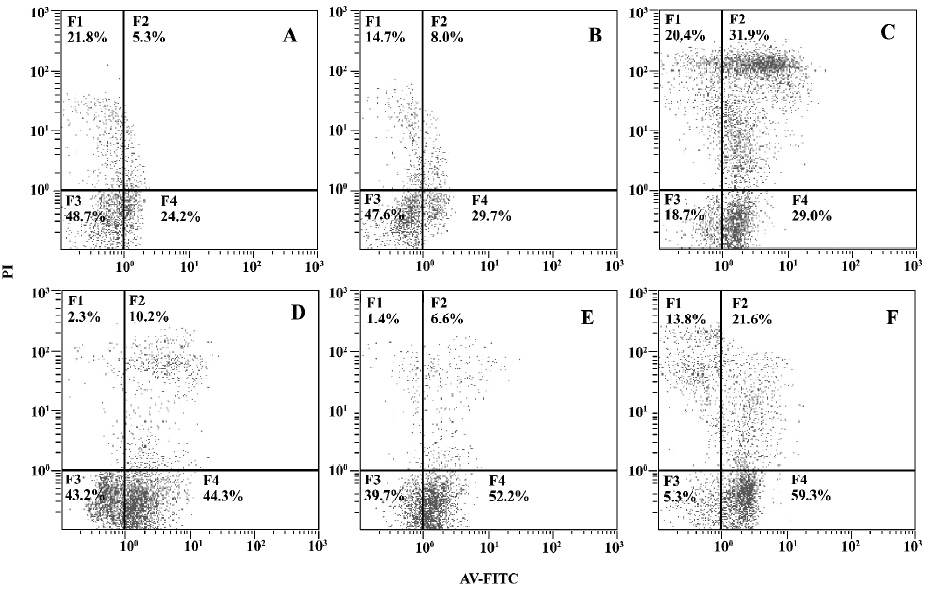
Discussion
Corticosteroids have remained the drug of choice for SLE therapy, but they sometimes need to be combined with other immunosuppressants such as azathioprine, cyclophospha-mide, methotrexate, cyclosporin A, FK506, and mycophenol-ate mofetil, in order to inhibit the abnormally enhanced immune and inflammatory reactions more effectively. The efficacy of these drugs is basically empirical and needs more rigorous and scientific experiments and clinical evaluation.
The novel immunosuppressive agent MMF is originally used to prevent rejection. Taken orally, MMF is metabolized to MPA, and the latter non-competitively and reversibly inhibits IMPDH, which catalyses a rate-limiting step in the de novo synthesis pathway of purine nucleotides. As lymphocytes are highly dependent on the de novo pathway, MMF has a relatively potent effect on lymphocytes[7]. Allison and Eugui suggested that MMF inhibits the proliferation of both B and T lymphocytes and decreases antibody production[7]. In addition, MPA affects several crucial aspects of the inflammatory response and has little adverse impact on other tissues because of its relatively specific effect[8,9].
It is well-known that in SLE there is a severe disorder in the cytokine network and a significantly elevated level of inflammation-related cytokines such as TNF-α, IL-6, IL-10, IL-12, IL-18, IFN-α, and IFN-γ[5]. It has been confirmed that IL-10 enhances B-cell proliferation and differentiation and stimulates the production of pathogenic anti-DNA autoantibody in SLE. Moreover, IL-10 levels correlate with the disease activity of SLE[5]. IL-10 also promotes apoptosis of T lymphocytes through Fas-FasL pathway by inducing Fas-FasL expression[3]. Recently, the role of IFN-γ or IL-12 in SLE pathogenesis has been noted. IFN-γ or IL-12 has been confirmed to be positively correlated with the disease activity of SLE[5], and may participate in the inflammatory damage in local tissues. Previous studies on animals have corroborated the inhibitive effect of MPA on both T and B lymphocytes[10,11]. In the present study, we demonstrated that MPA, at a concentration of 10 μmol/L, could significantly reduce the abnormally elevated levels of IL-10, IL-12, and IFN-γ produced by cultured PBMC from SLE patients, and decrease the percentage of CD4+ cells producing IL-10 or producing IFN-γ, especially of CD4+IFN-γ-+I L-10+ subset, in cultured PBMC of SLE patients. In this regard, the effect of MPA was very different from that of DEX. In contrast to MPA, DEX promoted IL-10 production of SLE PBMC induced by PHA (10 μg/mL) and increased IL-10 producing cell percentage, while the inhibitory effect of DEX on IL-12 and IFN-γ production was not as obvious as that of MPA. As a result of the lack of cell numbers, we observed some effects of MPA or DEX on different patients. Though patients in the tables belong to different cohorts, each table indicates a trend by itself. It is not the absolute cell number but the trend the result implied that really counts.
Regulatory T (Tr) cells can actively inhibit the activation and proliferation of those self-reactive T cells evading the immunologic tolerance and thus become a hot topic in immunologic research. Tr can be categorized into three subsets, Tr1, Th3, and CD4+CD25+Treg[12]. Dieckmann et al reported that Tr could secrete IL-10 and IFN-γ simultaneously, and inhibit the function of the Th1 subset[13]. Levings et al defines Tr1 as CD4+IFN-γ+IL-10+T cell. CD4+CD25+Treg comprising a unique lineage of thymus-derived regulatory T cells that have a potent contact-dependent, cytokine-independent (IL-10,TGF-β) mechanism of inhibitory action on CD4+CD25-T cells[14,15]. Tr are believed to be involved in the pathogenesis of SLE and to be increased in SLE with a disorder function by breaking its anergic/suppressive state[16,17]. Our previous study demonstrated that the percentage of CD4+IFN-γ+IL-10+ subset in fresh peripheral blood from active SLE patients were significantly increased, but were decreased after glucocorticoid therapy[18]. In the present study, the increase of CD4+IFN-γ+IL-10+ subset was confirmed once more. However, MPA did not have a significant effect on the percentage of CD4+IFN-γ+IL-10+ subset, while DEX could promote its elevation. Maybe it is reasonable to suspect that MPA and DEX do not directly suppress CD4+IFN-γ+IL-10+ Tr cells. In vivo, a decrease of CD4+IFN-γ+IL-10+ subset after glucocorticoid therapy may be due to the breaking of abnormal activation of self-reactive T lymphocytes.
The apoptosis of PBMC, especially of active lympho-cytes, from patients with SLE was demonstrated to participate in the pathogenesis. But apoptosis may have a dual role in the pathogenesis of SLE. On one hand, this process may be integral in the clonal deletion of self-reactive lymphocytes and maintenance of peripheral tolerance. Defects in inducing apoptosis could lead to the persistence of autoreactive T- or B-cells. On the other hand, apoptosis generates altered self-antigens which can stimulate an autoimmune response in sensitive persons[19].
Our study showed that apoptosis of PBMC from SLE patients was significantly increased compared with that from normal controls, in company with increases of sFas and sFasL, which could be on account of lymphocyte activation. The present study also showed that MPA could decrease the levels of sFas and sFasL in the supernatants of cultured SLE PBMC while enhancing the apoptosis of SLE PBMC. DEX showed a much stronger promoting effect on the apoptosis, but did not show any promoting effect on sFasL levels. These data implicated that MPA and DEX induced apoptosis of SLE PBMC through a pathway different from Fas-FasL pathway, although the inhibitory effect of DEX on sFas secretion was favorable for apoptosis owing to the reduction of interference with apoptotic process by sFas. MPA and DEX may inhibit Fas and FasL expression directly or indirectly by inhibiting the activation of T lymphocytes. sFasL is an apoptosis-promoting cytokine. The fact that MPA and DEX increase apoptosis of PBMC while decreasing the sFasL level could be explained by the hypothesis that MPA and DEX mediate apoptosis of activated T lymphocytes by a Fas-FasL-independent pathway. That MPA and DEX are beneficial in SLE treatment while promoting apoptosis of SLE PBMC may seem contradictory, but reasonable if we postulate that these two drugs promote the apoptosis of those self-reactive lymphocytes.
In conclusion, MPA could inhibit the expression of SLE-associated cytokines such as IL-10, IL-12, and IFN-γ by PBMC, reduce the abnormal elevation of the percentage of CD4+IFN-γ+IL-10+ subset and promote apoptosis of SLE PBMC by a Fas-FasL-independent pathway. These effects may partly explain the efficacy of the drug for treating SLE. It is worth pointing out that MPA has superiority over DEX in reducing IL-10 production and inhibiting proliferation of IL-10-producing CD4+ T subset.
References
- Marai I, Zandman-Goddard G, Sheonfeld Y. Apoptosis in systemic lupus erythematosus. Harefuah 2003; 142: 844?7. 877, 876.
- Bengtsson AA, Sturfelt G, Gullstrand B, Truedsson L. Induction of apoptosis in monocytes and lymphocytes by serum from patients with systemic lupus erythematosus — an additional mechanism to increased autoantigen load? Clin Exp Immunol 2004;135:535-43.
- Wang HJ, Xu J, Ji XH, Yang XF, Sun KY, Liu XH, et al. The abnormal apoptosis of T cell subsets and possible involvement of IL-10 in systemic lupus erythematosus. Cellular Immunol 2005;235:117-21.
- Lauwerys BR, VanSnick J, Houssiau FA. Serum IL-12 in systemic lupus erythematosus: absence of P70 heterodimers but presence of P40 monomers correlating with disease activity. Lupus 2002;11:384-7.
- Jiang YZ, Ji XH, Li YF, Su DL. The abnormal expression of IL-10, IFN-γ, IP-10, and IL-12 in patients with systemic lupus erythematosus and the effects of cyclosporine A and cyclophosphamide on it. Acta Univ Med Nanjing 2005;25:633-6.
- Karim MY, Alba P, Cuadrado MJ, Abbs IC. Mycophenolate mofetil for systemic lupus erythematosus refractory to other immunosuppressive agents. Rheumatology 2002;41:876-82.
- Allison AC, Eugui EM. Mycophenolate mofetil and its mechanisms of action. Immunopharmacology 2000;47:85-118.
- Raab M, Daxecker H, Karimi A, Markovic S, Cichna M, Markl P, et al. In vitro effects of mycophenolica acid on the nucleotide pool and on the expression of adhesion molecules of human umbilical vein endothelial cells. Clin Chim Acta 2001;310:89-98.
- Badid C, Vincent M, McGregor B, Melin M, Hadj-Aissa A, Veysseyre C, et al. Mycophenolate mofetil reduces myofibroblast infiltration and collagen III deposition in rat remnant kidney. Kidney Int 2000;58:51-61.
- Izeradjene K, Revillard JP. Apoptosis of superantigen-activated T cells induced by mycophenolate mofetil treatment. Transplantation 2001;71:118-25.
- Jonsson CA, Carlsten H. Inosine monophosphate dehydrogenase (IMPDH) inhibition in vitro suppresses lymphocyte proliferation and the production of immunoglobulins, autoantibodies and cytokines in splenocytes from MRLlpr/lpr mice. Clin Exp Immunol 2001;124:486-91.
- Jonuleit H, Schmitt E. The regulatory T cell family: distinct subsets and their interrelations. J Immunol 2003;171:6323-7.
- Dieckmann D, Bruett CH, Ploettner H, Lutz MB, Schuler G. Human CD4+CD25+ regulatory, contact-dependent T cells induce interleukin 10杙roducing, contact-independent type 1-like regulatory T cells. J Exp Med 2002;196:247-53.
- Levings MK, Sangregorio R, Galbiati F, Squadrone S, Malefyt R, Roncarolo MG. IFN-alpha and IL-10 induce the differentiation of human type 1 T regulatory cells. J Immunol 2001;166:5530-9.
- Jonuleit H, Schmitt E, Stassen M, Tuettenberg A, Knop J, Enk AH. Identification and functional characterization of human CD4+CD25+T cells with regulatory properties isolated from peripheral blood. J Exp Med 2001;193:1285-94.
- Xu DM, Liu HY, Komai-koma M, Campbell C, McSharry C, Alexander J, et al. CD4+CD25+ regulatory T cells suppress differentiation and functions of Th1 and Th2 cell, Leishmania major infection, and colitis in mice. J Immunol 2003;170:394-9.
- Zhang CB, Yang XF, Wang HJ, Zhang MS, Su DL, Ke Y, et al. Analysis of peripheral blood CD4+CD25+ T cells from the patients with systemic lupus erythematosus. Acta Univ Med Nanjing 2004;24:455-8.
- Ke Y, Wang L, Shen YX, Wang HJ, Liu XH, Ji XH. The pathogenesis significance of IFN-γ+ and IL-10+ cells in patients with systemic lupus erythematosus. J Clin Dermatol 2003;32:638-40.
- Funauchi M, Sugiyama M. A possible role of apoptosis for regulating autoreactive responses in systemic lupus erythematosus. Lupus 2001;10:284-8.