Calcium channel blocking activity of calycosin, a major active component of Astragali Radix, on rat aorta1
Introduction
Astragali Radix, the dry root of Astragalus membranceus (Fisch) Bge, known as Huangqi in China, has been used for centuries as a primary Chinese tonic herb[1]. It contains numerous triterpene saponins, polysaccharides, and flavonoids, which are known to have various biological effects. In modern Chinese medicine, Huangqi is widely used as an immune modulator. It is also used as an adjunctive therapy to chemotherapy and radiation therapy in cancer[2,3]. Recently, a report was published on the vasorelaxation activities of Astragali Radix[4]. Calycosin, an isoflavonoid, is the major active component in Astragali Radix. It has been chosen as one of the marker compounds for the chemical evaluation or standardization of Astragali Radix and its products[5]. Calycosin displayed beneficial effects such as antitumor[6], antioxidation[7], anti-ischemia[8], inhibition of hyperperme-ability induced by low osmolarity[9], scavenging free radicals and neuroprotective activity[10]. However, the effect of calycosin on vasoactivity has not been reported as yet. The purpose of the present study was to investigate the vasoactivity of calycosin and its mechanisms.
Materials and methods
Chemicals and reagents Calycosin (purity 99%) was prepared by Natural Medicinal Chemistry Department of Peking University. Nw-nitro-L-arginine methyl ester (L-NAME) was the product of Sigma-Aldrich chemie Gmbh, Steinheim, Germany; phenylephrine (PHE) was purchased from Shanghai Harvest Pharmaceutical Co (Shanghai, China); indomethacin was the product of China Shijiazhuang Group Pharmaceutical Co; and nifedipine was the product of Tianjin Lisheng Pharmaceutical Co. All inorganic salts were purchased from Beijing Chemical Reagent.
Stock solutions of calycosin in Me2SO (0.1 mol/L) and nifedipine in 95% ethanol (9×10-3 mol/L) were stored at -20 °C and used within one week. Indomethacin was dissolved in 95% ethanol before use. Nifedipine and indomethacin were shielded from light with aluminium foil. The highest concentrations of Me2SO and ethanol, which were 0.1% and 0.001%, respectively, did not affect the response of the aorta rings. The concentrations given are the final concentration in the bath chambers.
Animals Male Sprague-Dawley rats were obtained from the Experimental Animal Center of Peking University. The experimental procedures were approved by the Local Committee on Animal Care and Use.
Tissue preparation Rats weighing 250–300 g were anesthetized with 50 mg/kg sodium pentobarbital (ip) and killed by exsanguination from the carotid artery. The thoracic aorta was isolated and placed into cold Krebs’ solution constantly aerated with 95% O2 and 5% CO2, from where the aorta was cleaned of the fat and connective tissue and cut into 3–5 mm wide rings. The vessel preparations were mounted with one stainless steel wire in the organ bath, and the other wire through the vessel lumen was connected to a force trans-ducer. Isometric tension change was recorded continuously on a two-channel physiological recorder (Chengdu Equipment Factory). The tissue was incubated in Krebs’ solution (pH 7.4) at 37 ºC, bubbled with a 95% O2-5% CO2 mixed gas. The Krebs’ solution contained the following composition (in mmol/L): NaCl 120, KCl 5.5, MgCl2·6H2O 1.2, NaH2PO4 1.56, CaCl2 2.5, NaHCO3 20, D-glucose 10.1, EDTA 0.03. The experimental procedures were performed as described by Chen and Kwan[11].
KCl or PHE-induced contractions on endothelium-intact aortic rings To evaluate the effect of calycosin on the contractions of aortic rings, two different experimental protocols were used. In one protocol, after 60 min equilibration in Krebs’ solution, aortic rings were precontracted with KCl (80 mmol/L) or PHE (1×10-6 mol/L), and when the plateau was attained, calycosin (1×10-6–1×10-4 mol/L) was added cumulatively to induce a concentration-dependent response in rings. In the other protocol, after 60 min of equilibration aortic rings were incubated with calycosin (30 µmol/L) or Me2SO for 20 min and then the concentration-response experiments to KCl (10–100 mmol/L) or to PHE (1×10-9–1×10-5 mol/L) were performed. Each ring was used for one determination.
PHE-induced contractions on endothelium-denuded arterial rings To investigate whether the relaxing response of calycosin depends on the endothelium, its effect was observed in endothelium-denuded arterial rings. Calycosin was added in a cumulative manner (1×10-6–1×10-4 mol/L) during the tonic contraction phase induced by PHE (1×10-6 mol/L). The endothelium was denuded from arterial rings by cannulating the forceps and gently rolling the vessel between the forceps and filter paper. The intactness or the denudation of endothelium was confirmed by the appearance or the disappearance of relaxant response to acetylcholine (10 µmol/L) in PHE (1×10-6 mol/L) pre-contracted rings.
Correlation of endothelial mediators and calycosin-induced relaxation To determine whether calycosin-produced relaxation was mediated by prostanoids or nitric oxide released from endothelial cells, endothelium-intact aortic rings were pre-incubated with the NO synthase inhibitor L-NAME (1×10-4 mol/L) or cyclooxygenase inhibitor indomethacin (1×10-5 mol/L) for 20 min. Cumulative concentrations of calycosin (1×10-6–1×10-4 mol/L) were then applied during the tonic phase of PHE (1×10-6 mol/L)-induced contraction. The effects of these inhibitors were studied by comparing the extent of relaxation induced by calycosin in the absence and the presence of these inhibitors.
Extracellular Ca2+-induced contraction of arterial rings in Ca2+-free Krebs’ solution After 60 min of equilibration in normal Krebs’ solution, the aortic rings with endothelium were incubated for 30 min in Ca2+-free Krebs’ solution with 0.5 mmol/L of EGTA and 40 mmol/L of KCl. The rings were then incubated for 20 min with calycosin or its vehicle control, and concentration-response curves were obtained by cumulatively adding CaCl2 (0.1–3.0 mmol/L).
PHE-induced contraction of arterial rings in Ca2+-free Krebs’ solution The aortic rings were equilibrated for 60 min in normal Krebs’ solution. To assure abundant Ca2+ storage in sarcoplasmic reticulum, contractions were induced by high K+ solution (80 mmol/L). The rings were then allowed to rest for 30 min in Ca2+-free Krebs’ solution. The response of calycosin (30 µmol/L) on PHE (1×10-6 mol/L)-induced contraction was observed.
Interaction of calycosin and nifedipine The rings were pretreated with nifedipine (90 nmol/L), a selective Ca2+ channel blocker, calycosin (30 µmol/L) or nifedipine plus calycosin for 20 min. Contractions were induced by KCl (80 mmol/L) or PHE (1×10-6 mol/L). The contraction altitude of aortic rings treated with these agents was compared to their respective vehicle-controls (Me2SO for calycosin; 0.1% ethanol for nifedipine; Me2SO+0.1% ethanol for calycosin plus nifedipine).
Statistical analysis Relaxant responses are expressed as mean±SD of relaxation percentage from KCl or PHE precontraction levels unless otherwise described in the figure or legends. In experiments involving concentration-response curves, the data were expressed as percentage of control contractile response induced by 80 mmol/L KCl. “n” denotes the number of the rings obtained from different rats. Statistical evaluation was carried out using the Student’s t-test. P<0.05 was considered as significant difference.
Results
Effects of calycosin on aortic ring tension development Calycosin up to 300 µmol/L had no effect on baseline tension in rat aortic rings. On arterial rings with endothelium, precontracted with PHE and KCl, calycosin (1×10-6–1×10-4 mol/L) produced a concentration-dependent and slowly developing relaxation. When pre-contracted with PHE, 100 µmol/L of calycosin produced a maximum relaxation up to 95.85%±2.67%, pD2 was 4.46±0.13. When pre-contracted with KCl, 300 µmol·L-1 of calycosin produced a maximum relaxation up to 99.06%±2.15%, and pD2 was 4.27±0.05 (Figure 1A).
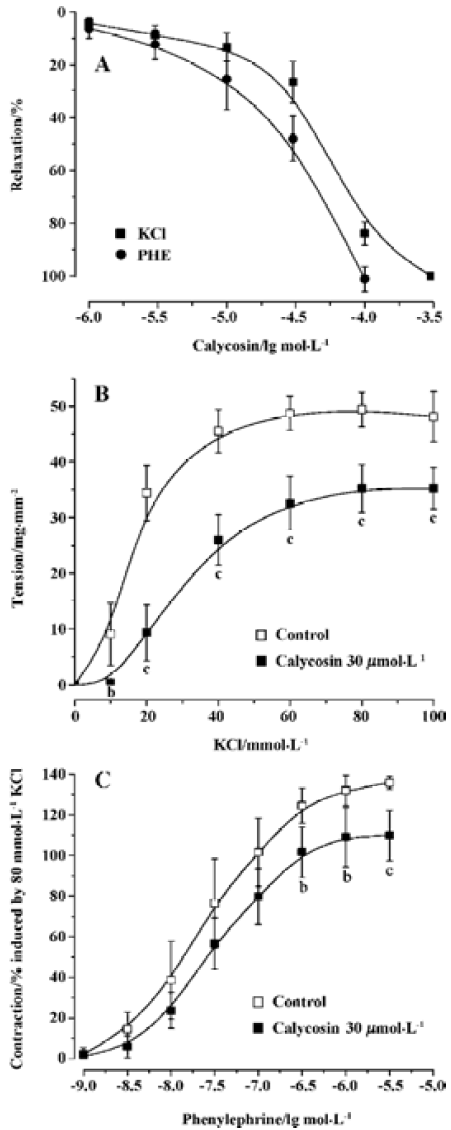
Calycosin shifted cumulative concentration-response curves for PHE or KCl to right, and lowered their Emax from 135.78%±3.21% to 109.77%±12.31% for PHE (P<0.05), and from 48.10±4.50 to35.30±3.7 mg/mm2 for KCl (P<0.01). It also decreased their pD2 from 7.62±0.04 to 7.51±0.05 for PHE (P<0.05), and from 1.88±0.14 to 1.54±0.12 (P<0.01) for KCl (Figure 1B and 1C). These results indicate that the Ca2+ antagonistic effects of calycosin were noncompetitive.
Effects of calycosin on rat aortic rings with or without endothelium To investigate whether calycosin-induced relaxation was endothelium dependent or not, experiments were performed in both endothelium-intact and -denuded aortic rings precontracted by PHE. The results showed that calycosin produced a concentration-dependent depression on PHE-induced contraction both in the endothelium-intact and in the endothelium-denuded aortic rings. The pD2 values for the two cases were 4.46±0.13 and 4.46±0.20, respec-tively. Concentration–response curves for cumulative calycosin treatment showed no difference in differently treated arteries (Figure 2A). It is suggested that the vascular relaxing effect of calycosin is independent from endothelium.
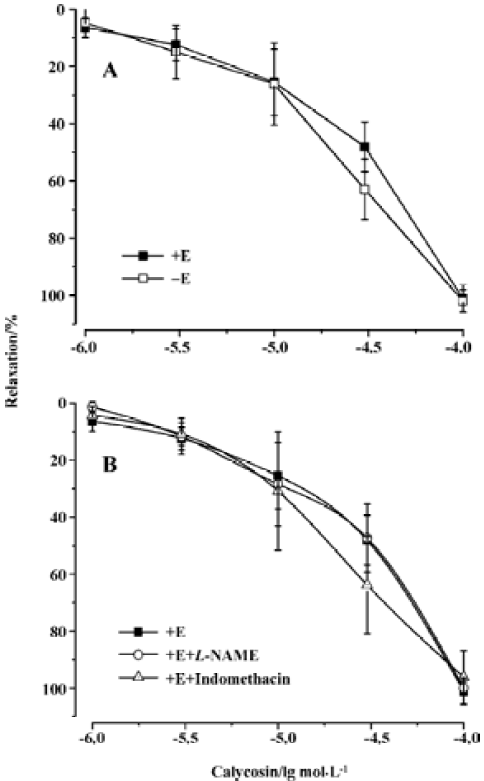
Effects of endothelial mediators on calycosin-induced vascular relaxation Pre-incubation of the rings with L-NAME or indomethacin did not influence calycosin-induced vasorelaxation. No difference was shown in the pD2 values (4.35±0.58 and 4.58±0.002) (Figure 2B), indicating that the vasodilation effect of calycosin is not mediated by nitric oxide or PGI2.
Effects of calycosin on extracellular Ca2+-induced contraction In the Ca2+-free solution, the cumulative addition of CaCl2 induced a concentration-dependent contraction of aortic rings depolarized by 40 mmol/L KCl (Figure 3). When the aortic rings were treated with vehicle, 3 mmol/L of CaCl2 induced the maximum contraction. When treated with calycosin (30 µmol/L), the maximum contraction was reduced by 31.65%±10.8% (P<0.01, compared with control), suggesting that calycosin blocked Ca2+ influx, thus lowering the contraction.
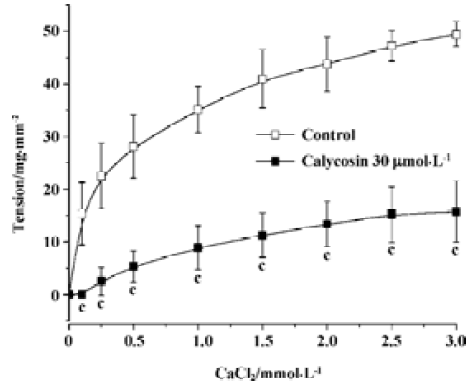
Influence of calycosin on calcium release from sarcoplasmic reticulum induced by PHE When extracellular Ca2+ was removed by using a Ca2+-free solution, PHE induced a transient contraction caused by the release of intracellular Ca2+. In this study, calycosin (30 µmol/L) did not have an effect on PHE (1×10-6 mol/L)-induced aortic ring contraction in Ca2+-free solution compared with the vehicle (contraction: 19.27±6.64 mg/mm2 for control; 19.23±5.73 mg/mm2 for calycosin), indicating that calycosin does not influence Ca2+ release from the sarcoplasmic reticulum.
Comparison of the inhibitory effects of calycosin and nifedipine on KCl- or PHE-induced contraction For the sake of comparison, the blockade of KCl or PHE induced contractions with 90 nmol/L of nifedipine was also observed. KCl- and PHE-induced contractions were all reduced by calycosin or by nifedipine (Figure 4, 5). Despite the great concentration difference between the two agents, nifedipine displayed a more potent effect in blocking KCl-induced contraction than calycosin (calycosin: 29.9±9.2 mg/mm2; nifedipine: 6.4±4.1 mg/mm2). On the PHE-induced contraction, the reduction of tension produced by calycosin was similar to that by nifedipine (41.3±5.7 mg/mm2 for calycosin and 40.8±7.5 mg/mm2 for nifedipine). On PHE-induced contraction, the combined use of calycosin and nifedipine showed more potent inhibitory effect than either agent alone. On KCl-induced contraction, the combined use of the two agents showed more potent inhibitory effect than calycosin, and the effect of combined use of the two agents did not show change compared with nifedipine alone.
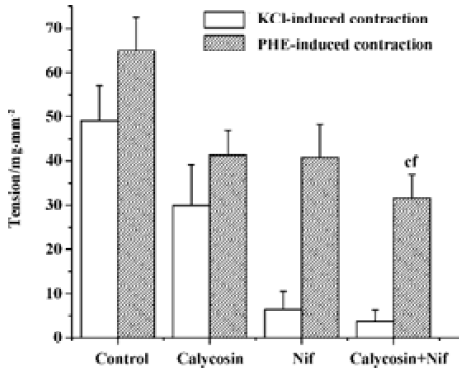
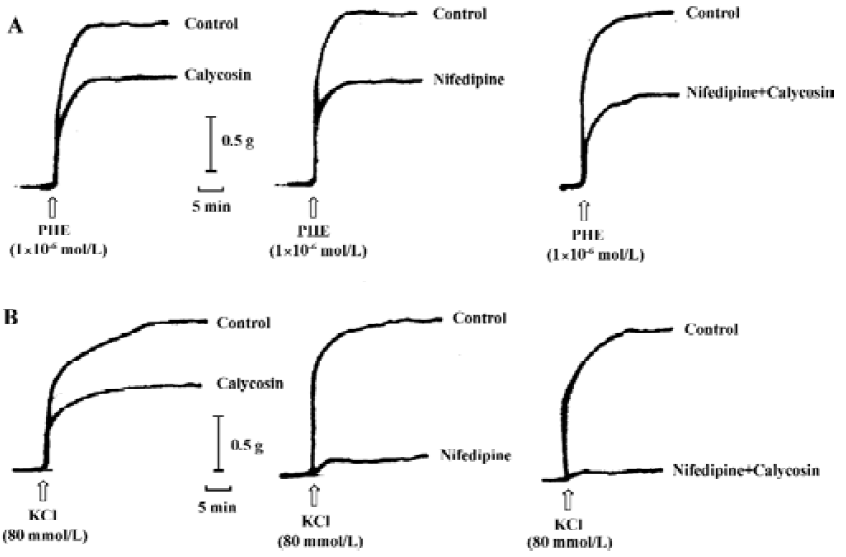
Discussion
Calycosin has been evidenced to have various pharmacological effects[6–10], and we have demonstrated its novel pharmacological activity (eg, vaso-relaxation) in this study. Calycosin produced concentration-dependent relaxation on rat thoracic aortic rings pre-contracted with PHE or KCl, indicating it inhibits voltage-operated Ca2+ channels (VOC), it may also inhibit receptor-operated Ca2+ channels (ROC), but further and sufficient evidence is needed. The pD2 values for endothelium intact rings and endothelium-denuded rings were not different, and its effect on aortic contraction was not mediated by NO or PGI2. It is suggested that the inhibitory effect of calycosin on PHE-induced contraction was endothelium-independent, and the target of calycosin may be on vascular smooth muscle cells but not on endothelial cells. Smooth muscle contracts in response to the activation of voltage-dependent and receptor-operated Ca2+ channels. Ca2+ is also released from intracellular stores upon activation[12]. KCl depolarizes and opens VOC, leading to Ca2+ influx, whilst PHE is able to open ROC to induce calcium influx in aorta without producing membrane depolarization[11,13–15]. Calycosin significantly inhibited KCl-induced vasocontraction, and antagonized Ca2+-induced contraction in aortic rings exposed to KCl, suggesting that calycosin decreased extracellular Ca2+ influx through VOC. Moreover, calycosin significantly inhibited PHE-induced contraction, indicating that it also decreased Ca2+ influx through ROC. Receptor-agonists increase Ca2+ sensitivity of contractile elements by activating the GTP-binding protein to make phosphatidylinositol turnover to generate inositol trispho-sphate (IP3), which releases Ca2+ from intracellular storage sites[16,17]. PHE-induced contraction in a Ca2+ free medium should result only from Ca2+ release from the intracellular store upon activation of IP3-sensitive Ca2+ channels[18]. Calycosin had no influence on PHE-induced transient contraction in a Ca2+-free medium in this study, suggesting that the vasorelaxant effect of calycosin was not related to the sarcolemma Ca2+ channels. It played a role through inhibiting extracellular Ca2+ influx but not intracellular release, that is, it only blocked Ca2+ channel on cell membrane but not on sarcolemma membrane. The inhibitory effect of calycosin on vasocontraction was compared with that of nifedipine, a typical L-type Ca2+ channel blocker. The results showed that calycosin decreased aortic ring contractions induced by KCl and PHE to a similar extent, but nifedipine displayed a more potent inhibitory effect on KCl-induced contraction than on PHE-induced contraction. Auger et al observed that nifedipine (100 nmol/L) produced a more potent depression on KCl (2–100 mmol/L) concentration-response curves than on PHE (1×10-9–1×10-4 mol/L) concentration-response curves[20]. Our results are in accordance with this observa-tion, although only one concentration of vasoconstrictor was used as did Damiani et al[12]. These findings indicate that calycosin had a different action mode from nifedipine, that is, calycosin comparably blocked ROC and VOC, but nifedipine mainly blocked VOC. In order to further confirm the action characteristic of calycosin, we studied the influence of nifedipine on the effect of calycosin. The results showed that the two agents showed additive relaxant effect on KCl-induced vasoconstriction. In the combined use, the presence of nifedipine eliminated voltage-dependent Ca2+ entry[19], thus the enhanced vascular relaxation produced by calycosin must be a result of an additional ion channel including ROC antagonism or K+ channel opening. Calycosin may act as a Ca2+ channel blocker, which may block both voltage-dependent Ca2+ channels and receptor-operated Ca2+ channels; this property is different from that of dihydro-pyridines. However, further study is required to clarify the detailed mechanisms of calycosin on Ca2+ channel.
In conclusion, calycosin is a vasorelaxant and its action is endothelium-independent and is unrelated to intracellular Ca2+ release. It is a noncompetitive Ca2+ channel blocker and the effect of calycosin on Ca2+ channel blockade may be different from that of dihydropyridines. This study demonstrated a novel pharmacological activity of calycosin and supplied a theoretic foundation for Astragali Radix application.
References
- Cathiroe P. Astragalus roots. In: Roy U, editor. American Herbal Pharmacopoeia and Therapeutic Compendium. CA: Santa Cruz; 1999. p 1–25.
- Lei H, Wang B, Li WP, Yang Y, Zhou AW, Chen MZ. Anti-ageing effect of astragalosidea and its mechanism of action. Acta Pharmacol Sin 2003;24:230-4.
- Wang YP, Li XY, Song CQ, Hu ZB. Effect of astragaloside IV on T, B lymphocyte proliferation and peritoneal macrophage function in mice. Acta Pharmacol Sin 2002;23:263-6.
- Zhang BQ, Hu SJ, Shan QX, Sun J, Xia Q. Relaxant effect of Astragalus membranaceus on smooth muscle cells of rat thoracic aorta. J Zhejiang Univ 2005;34:65-8. (Med Sci).
- Lin LZ, He XG, Lindenmaier M, Nolan G, Yang J, Cleary M, et al. Liquid chromatography-electrospray ionization mass spectrometry study of the flavonoids of the roots of Astragalus mongholicus and A membranaceus. J Chromatogr 2000;876:87-95.
- Kim DH, Yu KU, Bae EA, Han MJ. Metabolism of puerarin and daidzin by human intestinal bacteria and their relation to in vitro cytotoxicity. Biol Pharm Bull 1998;21:628-30.
- Toda S, Shirataki Y. Inhibitory effects of isoflavones in roots of Astragalus membranaceus Bunge (Astragali Radix) on lipid peroxidation by reactive oxygen species. Phytother Res 1998;12:59-61.
- Fan Y, Wu DZ, Gong YQ, Zhou JY, Hu ZI. Effects of calycosin on the impairment of barrier function induced by hypoxia in human umbilical vein endothelial cells. Eur J Pharmacol 2003;481:33-40.
- Wu DZ, Fan Y, Hen ZF, Song CQ, Hu ZB. Effect of flavonoids isolated from Astragalus membranaceus on the changes of endothelial permeability induced by low osmolarity. Pharmacol Clin Chin Mater Med 2000;16:16-8.
- Yu DH, Duan YL, Bao YM, Wei CL, An LJ. Isoflavonoids from Astragalus mongholicus protect PC12 cells from toxicity induced by L-glutamate. J Ethnopharmacol 2005;98:89-94.
- Chen CX, Kwan CY. Endothelium-independent vasorelaxation by leonurine, plant alkaloid purified from Chinese motherwort. Life Sci 2001;68:953-60.
- Damiani CE, Rossoni LV, Vassallo DV. Vasodilation effects of eugenol on rat thoracic aorta. Vasc Pharmacol 2003;40:59-66.
- Shi CC, Chen SY, Wang GJ, Liao JF, Chen CF. Vasodilation effect of Harman. Eur J Pharmacol 2000;390:319-25.
- Cicala C, Morello S, Iorio C, Capasso R, Borrelli F, Mascolo N. Vascular effects of caffeic acid phenethyl ester (CAPE) on isolated rat thoracic aorta. Life Sci 2003;73:73-80.
- Perusquia M, Villalon CM. Possible role of Ca2+ channels in the vasodilating effect of 5β-dihydrotestosterone in rat aorta. Eur J Pharmacol 1999;371:169-78.
- Sato K, Ozaki H, Karaki H. Changes in cytosolic calcium level in vascular smooth muscle strip measured simultaneously with contraction using fluorescent calcium indicator Fura 2. J Pharmacol Exp Ther 1988;246:294-300.
- Sato K, Satake N, Shibata S, Adachi Y, Karaki H. Mechanisms of vasoinhibitory effect of cardioprotective agent, CP-060S, in rat aorta. Eur J Pharmacol 2000;400:225-30.
- Islam MO, Yoshida Y, Koga T, Kojima M, Kangawa K, Imai S. Isolation and characterization of vascular smooth muscle inositol 1,4,5-trisphosphate receptor. Biochem J 1996;316:295-302.
- Mekata F. Current spread in smooth muscle of rabbit aorta. J Physiol 1974;242:143-55.
- Auger K, Beausejour A, Brochu M, St-Louis J. Increased Na+ intake during gestation in rats is associated with enhanced vascular reactivity and alterations of K+ and Ca2+ function. Am J Physiol Heart Circ Physiol 2004;287:H1848-56.