Addition of tanshinone IIA to UW solution decreases skeletal muscle ischemia-reperfusion injury1
Introduction
Ischemia-reperfusion (I/R) injury can compromise the clinical outcome of patients undergoing replantation, release of compartment syndrome, free tissue transfer, or other revascularization procedures, even in technically successful operations[1]. Thus, I/R injury has been very important in the field of reconstructive microsurgery. Although some progress has occurred, the problem has not been totally overcome[2,3]. In revascularization procedures, a certain period of ischemia is inevitable until blood flow is reestablished. Skeletal muscle is particularly susceptible to the deleterious effects of ischemia and reperfusion. A special strategy for muscle preservation is necessary to pursue good functional recovery.
Ischemia followed by reperfusion can lead to the production of free radicals that can scavenge endothelium-derived nitric oxide (NO) and induce endothelial dysfunction associated with loss of regulatory and protective properties[4]. Vascular dysfunction can ultimately lead to tissue injury. It has been reported that organ storage in preservation solutions with powerful antioxidant capacity might decrease or prevent I/R injury associated with oxygen-derived free radicals[5]. University of Wisconsin (UW) solution has been widely used in the setting of liver, pancreas, and kidney transplantation. However, it is arguable whether or not UW solution is beneficial for preserving skeletal muscle[6–9].
Tanshinone IIA is the major component extracted from the traditional herbal medicine Salvia miltiorrhiza Bunge. Tanshinone IIA has been shown to eliminate oxygen-derived free radicals, to stabilize vascular endothelial function, and to ameliorate microcirculation. It is widely used to treat various cardiovascular and cerebrovascular diseases[10–12]. We hypothesized that adding tanshinone IIA to UW solution would increase the anti-oxidant and anti-ischemic properties of UW solution, which would in turn decrease the severity of I/R injury in skeletal muscle. In this study, we used a rat ischemic limb model to investigate whether tanshinone IIA could improve the effect of UW solution for skeletal muscle preservation and to determine the dose range of tanshinone IIA providing optimal protection from I/R injury.
Materials and methods
Animals Forty adult male Sprague–Dawley rats (weight, 400–450 g) were obtained from the Experimental Animal Center, Sun Yat-Sen University of China. Rats were housed in pairs on a 12:12 h (light-dark) cycle and were provided with a standard rodent diet and water. The National Research Council’s guidelines for use and care of laboratory animals were followed and the study design was approved by the Ethics Committee of China.
Surgical procedure Operations were performed under anesthesia (induction with ip injection of 45 mg/kg sodium pentobarbital, followed by additional injections of 15 mg/kg as needed). After induction of anesthesia, the fur was completely removed from the left-hind limb with an electric shaver. A left groin incision was made from the tip of the scrotum to the left anterior superior iliac spine (ASIS), exposing the inguinal ligament and the deepest part of the abdominal wall. Under an operating microscope (×10), the femoral artery and vein and superficial epigastric artery and vein were dissected. Except the femoral artery and vein, all tissues were transected to eliminate collateral blood supply to the pelvis. The femoral artery and vein were spared for clamping, and the superficial epigastric artery and vein were cannulated with PE-50 polyethylene tubes for perfusion washout and blood sampling. During the entire experiment, body and experimental limb temperatures were maintained at 36 °C to 37 °C and 32 °C to 33 °C, respectively, with use of heating lamps. At the end of the experiment, the animals were killed with an intraperitoneal overdose of sodium pentobarbital.
Groups The animals were randomly divided into one of five groups. In the control, I/R group (n=8), blood flow to the left-hind limb was stopped by placing Acland-type microvascular clamps on the femoral artery and vein for 4 h. No perfusion washout was used, and limb surface temperature was kept at 32–33 °C. After 4 h of ischemia, clamps were released to restore natural blood flow to the limb for a 6-h reperfusion period. In the second, UW perfusion group (n=8), there was a 3.5-h period of ischemia, after which the ischemic limb was continuously perfused for 0.5 h via the left superficial epigastric artery with UW solution (Via Span, Du Pont, Wilmington, DE, USA) at 25 °C. A gravity perfusion apparatus at a height of 75 cm produced sufficient pressure to wash out stagnant blood rapidly (1–2 mL/min). Subsequently, natural perfusion was allowed to return for 6 h. In the three other groups, the ischemic limb was perfused for 0.5 h with UW solution plus tanshinone IIA (UW+T) at 0.05 mg/mL (UW+T0.05; n=8), UW+T at 0.1 mg/mL (UW+T0.1; n=8), or UW+T at 0.2 mg/mL (UW+T0.2; n=8), respectively. Other experimental procedures were the same as for the UW group.
Measurement of blood flow During the 6-h reperfusion period, blood flow to the skeletal muscles in the hind limb was measured by laser Doppler probe (Periflux Pf5001, Perimed, Jarfalla, Sweden). Measurements of reperfusion blood flow were expressed as the ratio of the experimental limb relative to the contralateral control limb (E/C ratio) of the same animal.
Laboratory testing Venous blood samples were taken from the superficial epigastric vein before ischemia and after 2 h, 4 h, and 6 h of reperfusion. Samples were centrifuged at 3000 rpm for 5 min to collect serum and then stored at -20 °C. Serum levels of creatine phosphokinase (CPK), aspartate aminotransferase (AST), and lactate dehydrogenase (LDH) were measured with an auto-biochemical analyzer (Toshiba, Tokyo, Japan).
Biochemical analyses Gastrocnemius muscle and tibialis anterior muscle were obtained from the experimental limb after 4 h of ischemia and after 6 h of reperfusion, respectively, for detection of water content, superoxide dismutase (SOD), malondialdehyde (MDA), and adenosine triphosphatase (ATPase) in skeletal muscle. Biopsy specimens of 20 to 30 mg skeletal muscle were taken, put on wax paper, and dried to a constant weight at 85 °C for 24 h. Tissue water content was calculated according the following equation: % water= (wet tissue weight–dry tissue weight)×100/wet tissue weight. Weighed segments of tissue were thawed, homogenized in ice-cold buffers, and then SOD, MDA, and ATPase levels in the muscle homogenate were analyzed following the instructions of the test kits obtained from Nanjing Jiancheng Bioengineering Institute (Nanjing, China).
Immunohistochemical examination Muscle samples were fixed in 10% neutral buffered formalin, embedded in paraffin, and cut into 4-μm thick sections. With the streptavidin-biotin complex (SABC) method for detection of ICAM-1 expression in skeletal muscle, immunohistochemical staining was performed using rabbit anti-rat ICAM-1 polyclonal antibody (Sigma, St Louis, MO, USA) as a primary antibody and goat anti-rabbit IgG monoclonal antibody as a secondary antibody (Sigma). The percentage of positive vessels was obtained by observing 20 consecutive non-overlapping eye-fields. Terminal deoxynucleotidyl transferase-mediated (dUTP) nick end-labeling (TUNEL) staining of sections was performed for apoptotic nuclei with an in situ apoptosis detection kit (Sigma), according to the manufacturer’s instructions and examined by light microscopy. Control sections of rat ovary were used as a positive control[13] (Palumbo & Yeh 1994) and showed widespread nuclear staining within degenerating follicles. Negative controls of ischemic-reperfused muscle, in which biotinylated dUTP had been omitted from staining, showed no TUNEL-positive nuclei. The percentage of apoptotic nuclei was measured and this represents the apoptotic index (AI).
Drugs Tanshinone IIA (molecular formula: C19H18O3, molecular weight: 294.33) was obtained from Shanghai Pharmaceutical Co (Shanghai, China).
Data presentation and statistical analysis All data are expressed as the mean±SD. The data were analyzed by one-way analysis of variance (ANOVA), and all comparisons were tested by the Student–Neuman–Keuls’ multiple comparisons test. Statistical differences were considered significant at the P<0.05 level.
Results
Measurement of blood flow findings In I/R (control) rats, skeletal muscle luxury perfusion was observed after reperfusion. Reperfusion blood flow increased and peaked during the first hour of reperfusion. Peak flow was 216%±22% of the contralateral, intact, limb. Then blood flow gradually decreased. It was (63%±10%) lower than that in the contralateral limb after 6 h of reperfusion (Figure 1). In the four perfusion groups, no skeletal muscle luxury perfusion was observed, and blood flow slightly waved after reperfusion. After 6 h of reperfusion, blood flow in the UW group was only 78%±12% of that in the contralateral limb, while blood flow was 94%±14% in UW+T0.05 group (P<0.05 versus UW), 101%±15% in UW+T0.1 group (P<0.01 versus UW), and 103%±13% in UW+T0.2 group (P<0.01 versus UW). Reperfusion blood flow in the three UW+T groups was increased by 15% to 25% compared with blood flow in the UW group (Figure1).
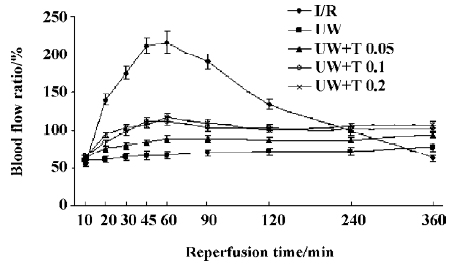
Laboratory findings Before induction of ischemia, there was no difference in the serum levels of CPK, LDH, and AST across all five groups. After reperfusion, serum levels of CPK, LDH, and AST were increased in I/R (control) rats, with an upward trend as duration of reperfusion increased (Figure 2), suggesting muscle damage was aggravated during reperfusion. Following perfusion washout with UW solution during the ischemic period, serum levels of CPK, LDH, and AST were significantly decreased by approximately 20% to 30% compared with levels in I/R rats during the first 2 h of reperfusion (P<0.01). However, there was no significant difference between UW and I/R rats after 4 h or 6 h of reperfusion (P>0.05). In contrast, perfusion using UW solution with tanshinone IIA (0.05, 0.1, or 0.2 mg/ mL) resulted in a significant reduction of serum levels of CPK, LDH, and AST compared with those in I/R rats at each reperfusion time point (2-h and 4-h, P<0.01; 6-h, P<0.05, P<0.01). In addition, UW solution with a higher dose of tanshinone IIA (0.1 and 0.2 mg/mL) produced greater reductions in leakage of intracellular enzymes than UW solution during perfusion (P<0.01; Figure 2).
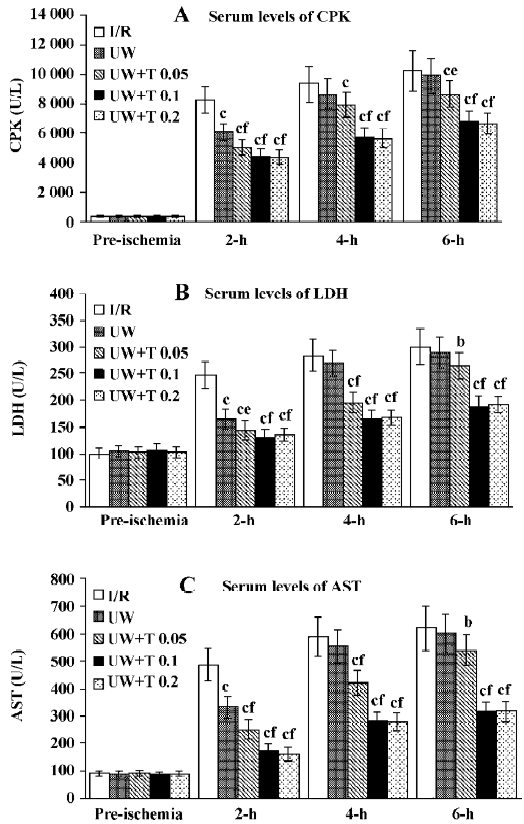
Biochemical findings After 4 h of ischemia, values for all four perfusion groups (UW or UW+T) of tissue water content, MDA, SOD, and ATPase were significantly different from those in the I/R group (P<0.05, P<0.01; Table 1). However, after 6 h of reperfusion there was no difference in any parameter between UW and I/R rats. In contrast, significant differences in all parameters for the three UW+T (0.05, 0.1, and 0.2 mg/mL) perfusion groups were observed in comparison to those for the I/R group after 6 h of reperfusion (P<0.01). Furthermore, all parameters for the three UW+T groups were significantly different from those for the UW group at 6 h of reperfusion (P<0.05, P<0.01; Table 1).
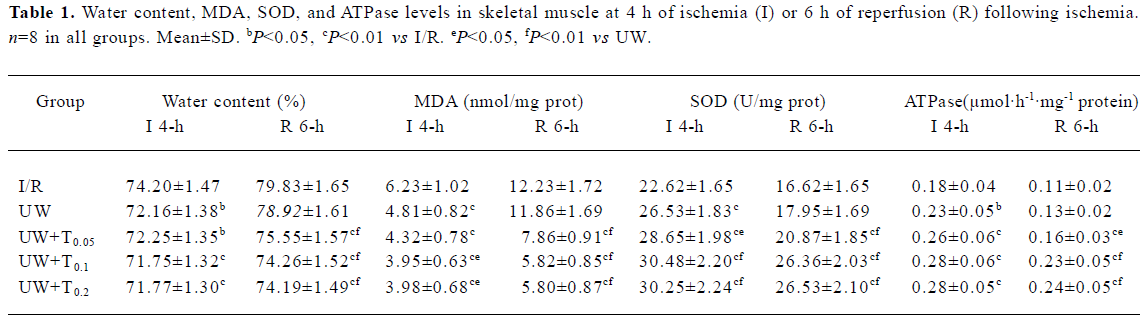
Full table
Effects on ICAM-1 expression Expression of ICAM-1 in vascular endothelium was observed in the same experimental animals using polyclonal antibody against ICAM-1 for immunostaining. After 6 h of reperfusion, there was a qualitative increase in expression of ICAM-1 in capillary, venous, and arterial endothelium of all experimental skeletal muscle sections obtained from the I/R group (Figure 3). The percentage of positively stained vessels was 57.24%±7.45% in I/R rats. Perfusion with UW or UW+T decreased the percentage of positively stained vessels to 45.18%±5.56% for UW rats (P<0.05 versus I/R), to 37.69%±4.57% for UW+T0.05 rats (P<0.01 versus I/R), to 33.40%±3.82% in UW+T0.1 rats (P<0.01 versus I/R), and to 33.76%±3.90% in UW+T0.2 rats (P<0.01 versus I/R), indicating that perfusion washout with UW and UW+T inhibits expression of ICAM-1, especially perfusion with UW+T (UW+T0.05 versus UW, P<0.05; UW+ T0.1and UW+T0.2 versus UW, P<0.01).
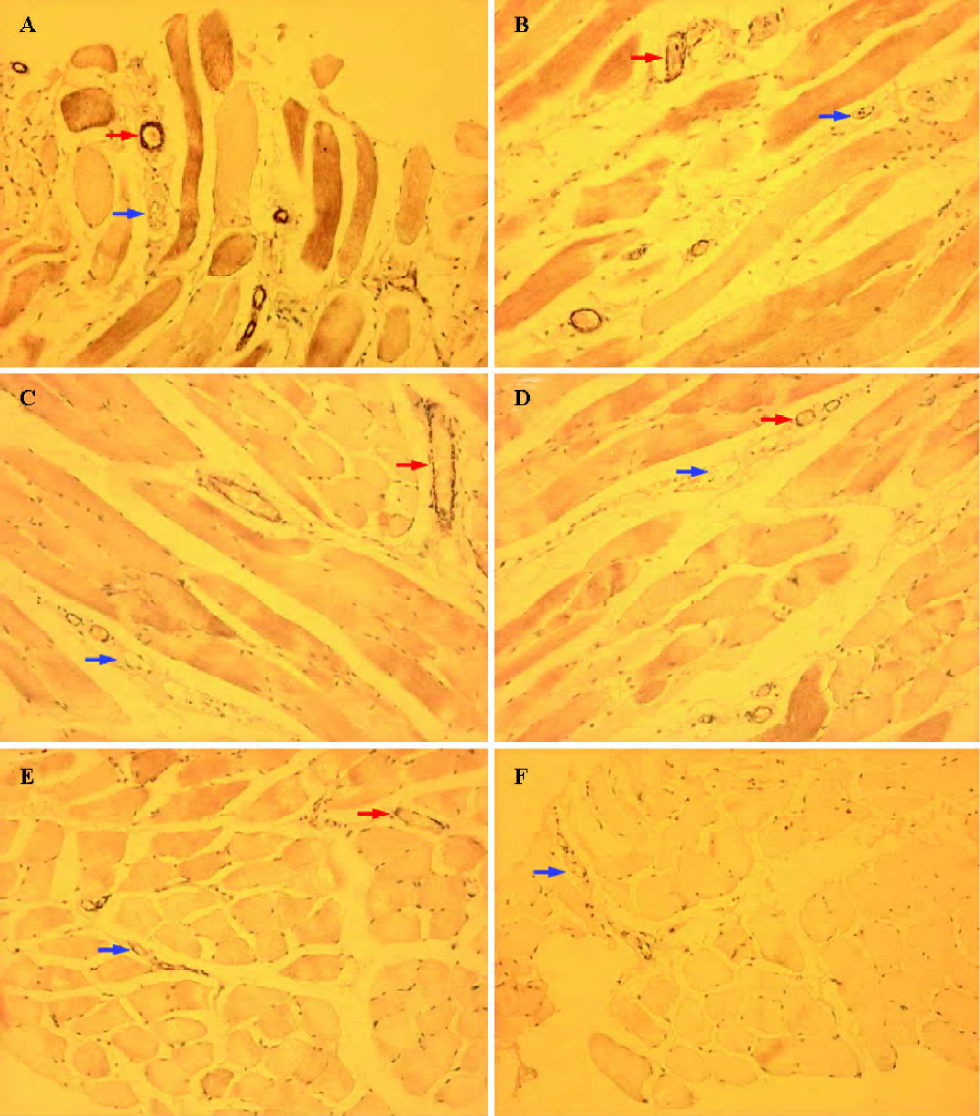
In situ detection of apoptotic cells After 6 h of reperfusion, many TUNEL positively stained nuclei were detected in I/R rats (apoptotic nuclei, 21.85%±6.84%; Figure 4). Perfusion with UW or UW+T significantly decreased the prevalence of apoptotic nuclei, with apoptotic indexes decreased to 15.70%±4.15% in UW rats (P<0.05 versus I/R), to 12.35%±3.95% in UW+T0.05 rats (P<0.05 versus I/R), to 9.40%±2.75% in UW+T0.1 rats (P<0.01 versus I/R), and to 8.87%±2.60% in UW+T0.2 rats (P<0.01 versus I/R). Furthermore, apoptotic indexes for the UW+T0.1and UW+T0.2 groups were significantly better (ie, less apoptosis visible) than the index for the UW group (P<0.01; Figure 4).
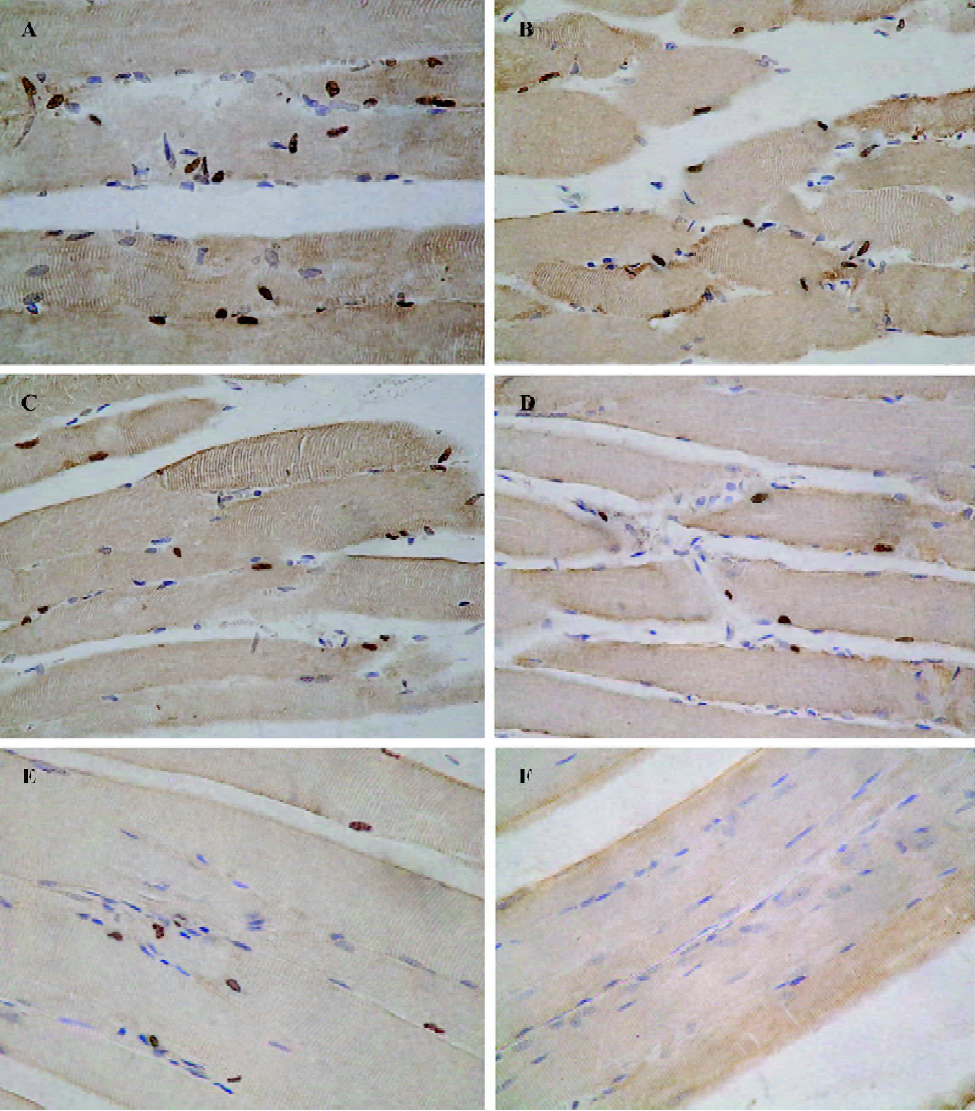
Discussion
In reconstructive surgery, revascularization procedures involving skeletal muscle are always subject to a certain period of ischemia followed by post-ischemic reperfusion. This may lead to significant injury and a reduced survival of skeletal muscle, a phenomenon at least partly mediated by the microvascular consequences of ischemia and reperfusion[14]. Thus I/R-induced injury to skeletal muscle has become a common clinical problem associated with significant morbidity and mortality[15]. Free hemoglobin in stagnant blood has been implicated as a mediator of hydroxyl radical production by means of an iron-catalyzed Fenton reaction. The hydroxyl radical attacks membrane phospholipids in a free radical process, resulting in phospholipid peroxidation and loss of membrane integrity[16]. For better preservation of ischemic tissue, stagnant blood may need to be removed and replaced with an appropriate organ preservation solution. UW solution is the most frequently used preservation solution for the liver, kidney, and pancreas, and it is associated with excellent clinical and experimental preservation results[17]. UW solution contains lactobionate and raffinose, both relatively large impermeant molecules that regulate cell volume, whereas reduced glutathione and allopurinol are included to prevent oxygen-derived free radical injury and adenosine is added to improve energy production. These components in UW solution are important for preserving tissues. In the present study, we investigated whether perfusion of rat ischemic hind limbs using UW solution or UW solution with tanshinone IIA (UW+T) prior to reperfusion could reduce I/R injury in skeletal muscle.
In this study, a luxury perfusion of skeletal muscle was observed in I/R rats during the first 2 h of reperfusion. Blood flow of skeletal muscle quickly increased after reperfusion, which could aggravate skeletal muscle damage due to the influence of reoxygenation and peroxidation reactions. However, we found that perfusion with UW and UW+T not only prevented skeletal muscle luxury perfusion, but that perfusion also inhibited cellular edema, the destruction of ATPase, phospholipid peroxidation, and intracellular enzyme leakage after ischemia and reperfusion. These results suggest that perfusion with UW or UW+T might be effective for preserving skeletal muscle viability in the skeletal setting. Tsuchida et al also reported that skeletal muscle ATP in amputated rat hind limb following 5 h of ischemia was well preserved by perfusion with UW solution[18]. However, in the current study there was no significant difference in laboratory test and biochemical analyses observed between UW group and I/R group after 4 h and 6 h of reperfusion. Furthermore, we found that the blood flow ratio in UW rats was significantly lower than that in other groups during the entire reperfusion period. These results suggest that the protective effect of UW solution for skeletal muscle might be diminished by insufficient blood supply in the affected skeletal muscle. Other studies with different animal models have also found that reperfusion blood flow was decreased after perfusion with UW solution, and these authors hypothesized that UW solution might have a detrimental effect on vascular endothelial function resulting in blood supply diminution[19,20]. Ge et al also demonstrated that use of UW solution resulted in deteriorated endothelial function of porcine and human coronary artery, and they concluded that the mechanism of this effect was related to reduced conductance of KCa and KATP channels[9].
It is well known that vascular endothelium plays a key role in regulating local blood supply by the release of various endothelium-derived relaxing factors[21]. Vascular endothelial function might be influenced by UW solution perfusion because it is the first tissue to make contact with the solution when perfusion is initiated. Tanshinone IIA is a pharmacologically active component isolated from the rhizome of the Chinese herb Salvia miltiorrhiza Bunge. Zhou et al reported that tanshinone IIA could stimulate mitochondrial NADH oxidation dose-dependently and partly restore NADH oxidation in the presence of a respiratory inhibitor. They thought it likely that tanshinone IIA could accept electrons from complex I similar to ferricyanide and be converted to its semiquinone form, which could then reduce oxygen molecules. Thus, tanshinone IIA might protect against I/R injury through an electron transfer reaction in mitochondria that protects against the formation of reactive oxygen radicals[12]. Jiang et al also reported that tanshinone IIA could inhibit expression of adhesion molecules (ICAM-1, P-selectin) in endothelial cells and exert a beneficial effect on vascular endothelium[22]. In the present study, we added various doses of tanshinone IIA to UW solution to investigate the effect of tanshinone IIA on the preservation of vascular endothelium. Our results showed that adding tanshinone IIA to UW solution increased reperfusion blood flow compared with UW solution alone, and tanshinone IIA improved all test parameters, indicating that UW solution with tanshinone IIA (0.05, 0.1, or 0.2 mg/mL) was more effective in reducing skeletal muscle damage than UW solution alone. In addition, we observed that the higher doses of tanshinone IIA (0.1 and 0.2 mg/mL) provided an even better effect, and this range probably provides us with an optimal dose for further research.
Some studies have demonstrated that activated polymorphonuclear leukocytes (neutrophils) and oxygen free radicals produced in ischemic tissue during reperfusion play important roles in the development of skeletal muscle injury[23-27]. It is a well-known phenomenon that I/R stimulates leukocyte-endothelium interactions through the upregulation of ICAM-1[28]. This eventually leads to increased transmigration of activated leukocytes into the affected tissue. Data from a study by Forbes et al suggested that the onset of parenchymal injury in skeletal muscle following ischemia is caused by the presence of activated leukocytes[29]. In our study, perfusion with UW and UW+T, especially UW+T at 0.05, 0.1 and 0.2 mg/mL, effectively inhibited the expression of ICAM-1, which might be beneficial in alleviating skeletal muscle damage induced by activated leukocytes by inhibition of leukocyte-endothelium interactions. Free radicals are produced mainly by activated neutrophils and the xanthine dehydrogenase/xanthine oxidase enzyme system after reperfusion, especially in activated neutrophils[24]. It is well known that free radicals cause apoptosis by a series of cell reactions involving DNA damage[30,31].We also observed inhibition of apoptosis following perfusion with UW or UW+T in this study, especially UW+T with higher doses of tanshinone IIA (eg, 0.1 and 0.2 mg/mL), indicating that these solutions were effective against apoptosis induced by free radicals.
This study did not attempt to elucidate the mechanism by which tanshinone IIA improved vascular endothelial function and decreased skeletal muscle damage. It is assumed that the anti-ischemic and antioxidant properties of tanshinone IIA played a key role in regulating local blood supply and reducing phospholipid peroxidation. The relevant mechanisms will be determined in further studies. The influence of hypothermia during ischemia and reoxygenation after ischemia was not considered in this study. Furthermore, appropriate perfusion conditions are also important to obtain successful treatment. Further study on those aspects is necessary for improved skeletal muscle preservation in the clinical setting.
In summary, the overall results in this study indicate that perfusion with UW solution is effective in preserving skeletal muscle integrity against I/R insult. However, skeletal muscle injury is augmented, probably due to the deterioration of vascular endothelial function, resulting in blood supply diminution. Tanshinone IIA as a beneficial adjunct to UW solution improves vascular endothelial function and increases the protective effect of UW solution. In conclusion, UW solution perfusion alone is not adequate for skeletal muscle preservation. To better preserve skeletal muscle, an appropriate dose of tanshinone IIA (0.1 or 0.2 mg/mL) added to UW solution is required.
References
- Allen DM, Chen LE, Seaber AV, Urbaniak JR. Calcitonin gene-related peptide and reperfusion injury. J Orthop Res 1997;15:243-8.
- Akbas H, Ozden M, Kanko M, Maral H, Bulbul S, Yavuz S, et al. Protective antioxidant effects of carvedilol in a rat model of ischaemia-reperfusion injury. J Int Med Res 2005;33:528-36.
- Troitzsch D, Vogt S, Abdul-Khaliq H, Moosdorf R. Muscle tissue oxygen tension and oxidative metabolism during ischemia and reperfusion. J Surg Res 2005;128:9-14.
- Mittermayr R, Valentini D, Fitzal F, Hallstrom S, Gasser H, Redl H. Protective effect of a novel NO-donor on ischemia/reperfusion injury in a rat epigastric flap model. Wound Repair Regen 2003;11:3-10.
- Perrault LP, Nickner C, Desjardins N, Dumont E, Thai P, Carrier M. Improved preservation of coronary endothelial function with Celsior compared with blood and crystalloid solutions in heart transplantation. J Heart Lung Transplant 2001;20:549-58.
- Wagh M, Pantazi G, Romeo R, Hurley JV, Morrison WA, Knight KR. Cold storage of rat skeletal muscle free flaps and pre-ischemic perfusion with modified UW solution. Microsurgery 2000;20:343-9.
- Norden MA, Rao VK, Southard JH. Improved preservation of rat hindlimbs with the University of Wisconsin solution and butanedione monoxime. Plast Reconstr Surg 1997;100:957-65.
- Fowler JD, Li X, Cooley BC. Brief ex vivo perfusion with heparinized and/or citrated whole blood enhances tolerance of free muscle flaps to prolonged ischemia. Microsurgery 1999;19:135-40.
- Ge ZD, He GW. Comparison of University of Wisconsin and St. Thomas’ hospital solutions on endothelium-derived hyperpolarizing factor-mediated function in coronary microarteries. Transplantation 2000;70:22-31.
- Xia WJ, Yang M, Fok TF, Li K, Chan WY, Ng PC, et al. Partial neuroprotective effect of pretreatment with tanshinone IIA on neonatal hypoxia-ischemia brain damage. Pediatr Res 2005;58:784-90.
- Lam BY, Lo AC, Sun X, Luo HW, Chung SK, Sucher NJ. Neuroprotective effects of tanshinones in transient focal cerebral ischemia in mice. Phytomedicine 2003;10:286-91.
- Zhou G, Jiang W, Zhao Y, Ma G, Xin W, Yin J, et al. Sodium tanshinone IIA sulfonate mediates electron transfer reaction in rat heart mitochondria. Biochem Pharmacol 2003;65:51-7.
- Luderer U, Diaz D, Faustman EM, Kavanagh TJ. Localization of glutamate cysteine ligase subunit mRNA within the rat ovary and relationship to follicular apoptosis. Mol Reprod Dev 2003;65:254-61.
- Adanali G, Ozer K, Siemionow M. Early and late effects of ischemic preconditioning on microcirculation of skeletal muscle flaps. Plast Reconstr Surg 2002;109:1344-51.
- Duran WN, Takenaka H, Hobson RW. Microvascular pathophysiology of skeletal muscle ischemia-reperfusion. Semin Vasc Surg 1998;11:203-14.
- Blaisdell FW. The pathophysiology of skeletal muscle ischemia and the reperfusion syndrome: a review. Cardiovasc Surg 2002;10:620-30.
- Janssen H, Janssen PH, Broelsch CE. UW is superior to Celsior and HTK in the protection of human liver endothelial cells against preservation injury. Liver Transpl 2004;10:1514-23.
- Tsuchida T, Kato T, Yamaga M, Ikebe K, Oniki Y, Irie H, et al. Effect of perfusion during ischemia on skeletal muscle. J Surg Res 2001;101:238-41.
- Tsuchida T, Kato T, Yamaga M, Ikebe K, Oniki Y, Irie H, et al. The effect of perfusion with UW solution on the skeletal muscle and vascular endothelial exocrine function in rat hindlimbs. J Surg Res 2003;110:266-71.
- Alper M, Ozek C, Erdem O, Denk M, Colen LB, Prewit R. Effect of University of Wisconsin and lactated Ringer’s solutions to ischemia-reperfusion injury in isolated cremaster flap. Microsurgery 2002;22:69-73.
- Mombouli JV, Vanhoutte PM. Endothelial dysfunction: from physiology to therapy. J Mol Cell Cardiol 1999;31:61-74.
- Jiang KY, Ruan CG, Gu ZL, Zhou WY, Guo CY. Effects of tanshinone II-A sulfonate on adhesion molecule expression of endothelial cells and platelets in vitro. Acta Pharmacol Sin 1998;19:47-50.
- Buttemeyer R, Philipp AW, Schlenzka L, Mall JW, Beissenhirtz M, Lisdat F. Epigallocatechin gallate can significantly decrease free oxygen radicals in the reperfusion injury in vivo. Transplant Proc 2003;35:3116-20.
- Rose S, Fiebrich M, Weber P, Dike J, Buhren V. Neutrophil activation after skeletal muscle ischemia in humans. Shock 1998;9:21-6.
- Nanobashvili J, Neumayer C, Fuegl A, Punz A, Blumer R, Mittlbock M, et al. Combined L-arginine and antioxidative vitamin treatment mollifies ischemia-reperfusion injury of skeletal muscle. J Vasc Surg 2004;39:868-77.
- Buttemeyer R, Philipp AW, Schlenzka L, Mall JW, Beissenhirtz M, Lisdat F. Epigallocatechin gallate can significantly decrease free oxygen radicals in the reperfusion injury in vivo. Transplant Proc 2003;35:3116-20.
- Peake J, Suzuki K. Neutrophil activation, antioxidant supplements and exercise-induced oxidative stress. Exerc Immunol Rev 2004;10:129-41.
- Menger MD, Laschke MW, Amon M, Schramm R, Thorlacius H, Rucker M, et al. Experimental models to study microcirculatory dysfunction in muscle ischemia-reperfusion and osteomyocu-taneous flap transfer. Langenbecks Arch Surg 2003;388:281-90.
- Forbes TL, Harris KA, Jamieson WG, DeRose G, Carson M, Potter RF. Leukocyte activity and tissue injury following ischemia reperfusion in skeletal muscle. Microvasc Res 1996;51:275-87.
- Dizdaroglu M. Base-excision repair of oxidative DNA damage by DNA glycosylases. Mutat Res 2005;591:45-59.
- Tharakan B, Dhanasekaran M, Manyam BV. Antioxidant and DNA protecting properties of anti-fatigue herb Trichopus zeylanicus. Phytother Res 2005;19:669-73.