Magnesium lithospermate B possesses inhibitory activity on Na+,K+-ATPase and neuroprotective effects against ischemic stroke1
Introduction
Danshen, the dried roots of medicinal plant Salvia miltiorrhiza, is one of the most popular Chinese herbal products widely used in many medicine preparations and formulae taken by people in certain Asian countries. Traditionally regarded as an effective medicine for eliminating blood stasis, relieving pain, promoting blood flow, stimulating menstrual discharge, and relaxing the mind, Danshen has been extensively used in the treatment of coronary heart disease, heart stroke, myocardial infarction, menstrual disorder, and other cerebrovascular diseases[1]. During the past decade, water-soluble components of Danshen have attracted escalating attention on the basis of their reported medicinal potency, although some lipid-soluble constituents, such as tanshi-nones, of this herb have been conventionally considered as the active ingredients[2–4]. Among the water-soluble com-ponents, magnesium lithospermate B (MLB), a derivative of caffeic acid tetramer, is present as the major soluble ingredient in Danshen, and has been demonstrated to possess several medicinal effects, such as vasodilating, antihypertensive, antioxidative, and free radical scavenging activities[5–10].
Cardiac glycosides, such as ouabain and digoxin, have been applied to the treatment of congestive heart failure for more than 2 centuries since William Withering published his famous monograph in 1785[11]. The molecular mechanism responsible for the therapeutic effect of cardiac glycosides lies in their reversible inhibition on the α-subunit of the membrane-bound Na+,K+-ATPase, mainly, but not exclusively, located in human myocardium[12,13]. This inhibition leads to the accumulation of sodium in cardiac cells which are enforced to promote the sodium-calcium exchange system in the cell membrane, thus causing a higher level of intracellular and myocardial calcium. The elevated intracellular calcium leads to an increased inotropism, accentuating the force of myocardial contraction by increasing the velocity and extent of sarcomere shortening, thus translating into increased stroke work for a given filling volume of pressure. Moreover, cardiac glycosides were recently demonstrated to provide neuroprotection against ischemic stroke in a cortical brain slice-based compound screening platform[14].
In light of the consentaneous utilization of Danshen and cardiac glycosides in cardiac therapy, we wondered if any constituents extracted from this Chinese herbal product might possess medicinal potency similar to that of cardiac glycosides via the same molecular mechanism. In this study, partial similarity of molecular configuration was assumed in chemical structures between MLB and ouabain, and their potency of inhibition on Na+,K+-ATPase activity of a commercial product as well as in purified membrane fractions from rat tissues was examined and compared. Neuropro-tection of MLB against ischemic stroke was also examined in a brain slice assay model.
Materials and methods
Structure comparison of ouabain and MLB The structure of ouabain is obtained from DrugBank[15] with accession number APRD00135. The structure of MLB resembling ouabain was constructed by exploring the possible rotamers by rotating the single bonds of MLB, and then the structure was minimized with molecular mechanics and an ab initio method (B3LYP/6-31G) using the Gaussian 03 package (Gaussian, Inc, Pittsburgh PA, USA) consecutively. The 3-D structures of ouabain and MLB were displayed using RasWin Molecular Graphics Windows Version 2.6 (Stevenage, Hertfordshire, UK).
Extraction and purification of MLB Dried roots (Danshen) of Salvia miltiorrhiza plants cultivated in a local farm were prepared in the Herbal Source Biotechnology Company (Tainan County, Taiwan). MLB was purified according to the protocol described by Tanaka et al[3]. The dried roots (8.8 kg) were extracted with 50 L methanol under reflux for 8 h and concentrated to a brown syrup. The syrup was suspended in H2O and partitioned with chloroform. MLB was harvested after repeated column chromatography of the H2O extract using Sephadex LH-20 and H2O as an eluent.
Preparation of plasma membrane from rat brains and heart Male Sprague-Dawley (Narll: SD) rats (3 months old) were purchased from the National Laboratory Animal Center (Nankang, Taipei) and raised under specific pathogen-free conditions. The rats, fed with rat chow (Rodent Laboratory Chow 5001, Purina, MO, USA) and tap water ad libitum, received humane care throughout the studies in accordance with the guidelines of a guidebook for the care and use of laboratory animals. The animals were sacrificed by decapitation, and the brain and the heart organs were removed immediately after complete exsanguination.
The plasma membrane was isolated from the rat brain and heart at 4 °C according to the methods described by Lin and Way[13,16]. Briefly, the brain cortex or heart was homogenized with 10–20 volumes of 0.32 mmol/L sucrose solution containing 5.0 mmol/L 4-(2-hydroxyethyl)-1-piperazine-ethane-sulfonic acid (HEPES) and 1.0 mmol/L EDTA (pH 7.5). The brain or heart homogenate was centrifuged at 1000×g for 10 min, and the resultant supernatant was further centrifuged at 17 000×g for 30 min to precipitate the crude plasma membrane fraction. The latter was washed twice and suspended in 0.32 mol/L sucrose HEPES-buffer and was subjected to centrifugation at 63 000×g for 1 h with a discontinuous sucrose density gradient consisting of successive layers of 0.3, 0.8, and 1.0 mmol/L. The plasma membrane collected at the interface between 0.8 and 1.0 mmol/L sucrose was suspended in 0.32 mol/L sucrose solution and used for enzyme assays within 2 h.
Western blot analysis For SDS-PAGE, Na+,K+-ATPase samples were mixed with sample buffer containing 62.5 mmol/L Tris-HCl (pH 6.8), 2% SDS, 0.02% bromophenol blue, 10% glycerol, and 5% β-mercaptoethanol according to the Bio-Rad (Hercules, CA, USA) instruction manual. The samples were boiled for 5 min and stored at 4 °C prior to electrophoresis. For the Western blot analysis, the proteins resolved in SDS-PAGE were transferred to a PVDF (polyvinyli-dene difluoride) membrane (PerkinElmer, Boston, MA, USA) in a Bio-Rad Trans-Blot system (USA) according to the manufacturer’s instructions. The membrane was subjected to immunodetection using an antibody against the β-subunit of Na+,K+-ATPase raised in rabbits (Sigma, St Louis, MO, USA) and anti-rabbit IgG raised in goats and conjugated with alkaline phosphatase (Jackson ImmunoResearch, West Grove, PA, USA) as the primary and secondary antibodies, respectively.
Measurement of Na+,K+-ATPase activity The activity of Na+,K+-ATPase was determined by measuring the amount of inorganic phosphate (Pi) liberated from ATP according to Muszbek et al[17]. A commercial Na+,K+-ATPase from porcine cerebral cortex (Sigma, USA, 0.3 units/mg) or plasma membrane fraction purified from rats was incorporated into a reaction mixture of 1 mL containing 3 mmol/L ATP, 5 mmol/L MgCl2, 80 mmol/L NaCl, 20 mmol/L KCl, and 40 mmol/L Tris-HCl (pH 7.4); the enzymatic reaction was terminated by 200 µL of 30% (w/v) trichloroacetic acid after 15 min. After centrifugation at 3300×g for 15 min, 500 µL supernatant was taken to measure the inorganic phosphate by the spectrophotometric method described by Goldberg and Fernander[18]. Activity was expressed as mmol Pi liberated from ATP by 1 mg of Na+,K+-ATPase during 1 h. Protein content was quantified with a Bradford protein assay kit (Sigma, USA). To observe the potency of the inhibitors, commercial Na+,K+-ATPase or the purified plasma membrane fraction was incubated with ouabain or MLB of various concentrations at 37 °C for 10 min prior to incorporation into the reaction mixture.
Cerebral ischemia of gerbils Eighteen male gerbils (65–80 g) were randomly divided into 3 groups: the control group and 2 MLB groups, and fed regular meals (20 mg·kg-1·d-1). In the present study, the focal cerebral ischemia was performed by occlusion of the right common carotid artery (CCA) and the right middle cerebral artery (MCA) as modified from the method of Yang et al[19]. All gerbils were anesthetized with chloral hydrate (360 mg/kg, ip) and allowed to breathe spontaneously. A thermostatically-regulated heating pad (CMA/150, Carnegie Medicine, Stockholm, Sweden) was used to maintain body temperature at 37 °C during ischemia/reperfusion. The gerbils’ heads were placed in a stereotaxic frame (Stoelting, Wood Dale, IL, USA) and the right CCA, exposed through a ventral midline incision in the neck, was carefully separated from the vago-sympathetic trunks for later occlusion by a mini-aneurysm clip. Following a midline incision, the skull was craniectomized to expose the right MCA. An 8–0 suture was positioned so that it encircled the right MCA for later occlusion. A focal ischemic/reperfusion lesion was made by simultaneous occlusion of the right CCA and the ipsilateral MCA for 60 min. The suture and the mini-aneurysm clip were released, and followed by 23 h reperfu-sion. Either distilled water (0.6 mL) or MLB (0.6 or 6 mg/kg in 0.6 mL) were fed via an intragastric route 1 h after reperfusion according to the research protocol.
Visualization of infarct size in gerbil brains For the determination of infarct sizes, the gerbils were sacrificed and the brains were removed and sliced. Slices 2 mm were immersed in a 2% solution of 2,3,5-triphenyltetrazolium chloride (TTC) stain as described by Bederson et al[20]. After 20 min, the slices were placed in 10% buffered formalin in the dark and refrigerated until photographed. The slices were projected and traced. The infarct size was quantified by cutting out and weighing the traced normal and infarct area using the Optimal computer software.
Statistical analysis The data are expressed as mean± SEM and analyzed by ANOVA and Student’s t-test. Differences were considered statistically significant at P<0.05.
Results
Structural comparison between ouabain and MLB Having a steroid backbone, ouabain, as well as other cardiac glycosides, possesses a rigid structure (Figure 1). MLB, a derivative of caffeic acid tetramer, also has a relatively rigid structure due to the formation of salt bridges between Mg2+ and the 4 oxygen atoms of carboxyl groups originating from the 4 caffeic acid fragments. The molecular organization and configuration of ouabain and MLB in 3-D structures are somewhat similar from a particular viewpoint (lower portions of the 2 3-D structures in Figure 1), although they are totally different compounds with distinct molecular weights (584.65 and 740.67 for ouabain and MLB, respectively).
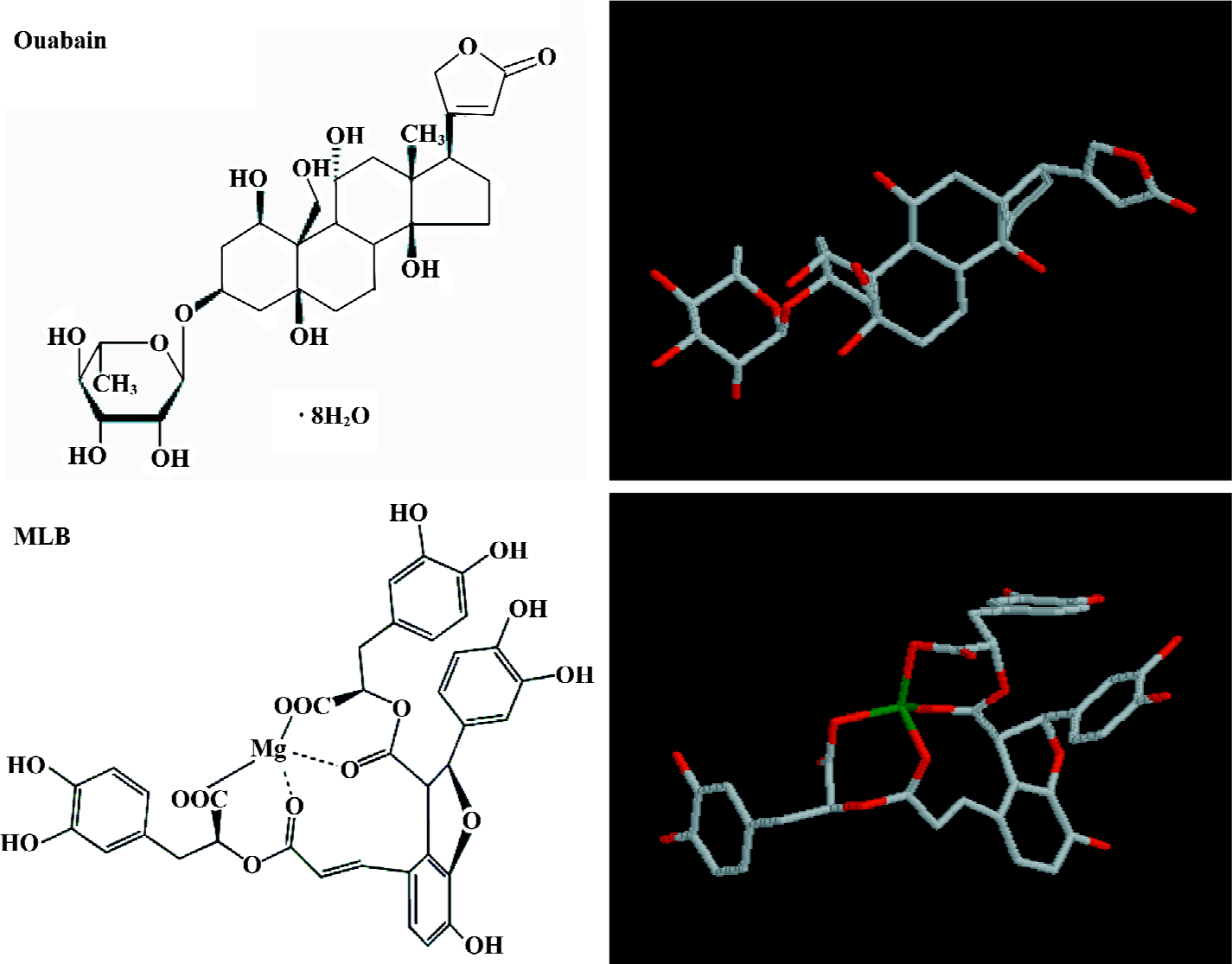
Inhibition of porcine Na+,K+-ATPase by ouabain and MLB To test if MLB could lead to a similar therapeutic effect via the same mechanism triggered by ouabain, that is, accentuating the force of myocardial contraction by elevating calcium concentration via the inhibition of Na+,K+-ATPase, a commercial Na+,K+-ATPase from porcine cerebral cortex was used to evaluate the inhibitory potency of MLB using ouabain as a control. The results showed that both ouabain and MLB could inhibit the commercial Na+,K+-ATPase in a dose-dependent manner (Figure 2). In our assay condition, the inhibition potency on Na+,K+-ATPase, equivalent to that for ouabain, was observed for MLB of approximately half dosage by weight.
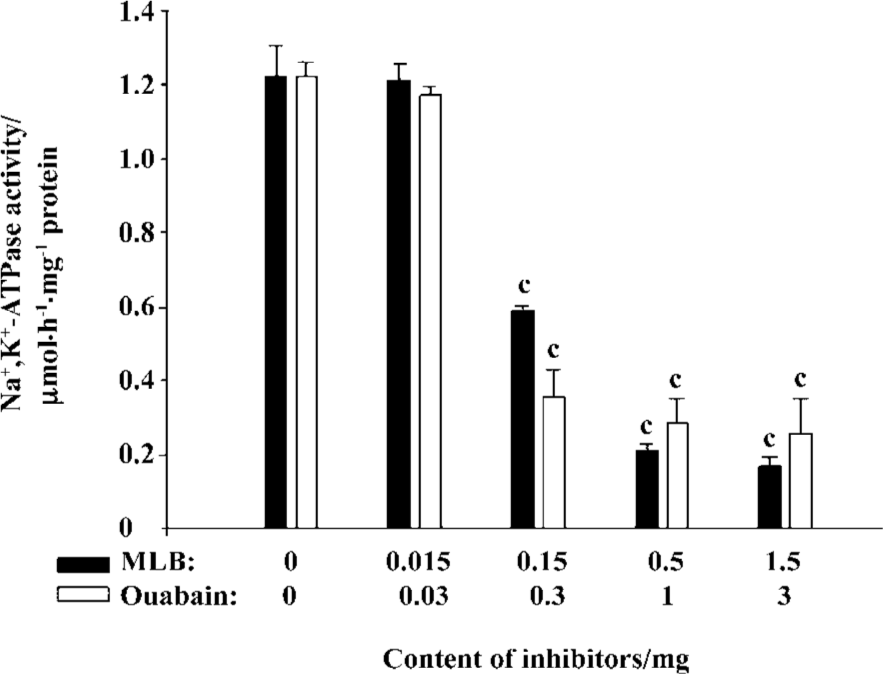
Inhibition of Na+,K+-ATPase in the purified plasma membrane from rat tissues The presence of Na+,K+-ATPase in the plasma membrane purified from rat brains was confirmed by Western blot analysis using the commercial porcine Na+,K+-ATPase as a positive control (Figure 3). Under the same assay conditions, the relative potency of ouabain and MLB was also observed for their inhibition on Na+,K+-ATPase activity of the plasma membrane purified from rat brains, although these 2 inhibitors exhibited lower competence in comparison with their inhibition on commercial Na+,K+-ATPase (Figure 4). No apparent difference was detected for MLB inhibition on Na+,K+-ATPase activity of the plasma membranes purified from rat heart and brain tissues, respectively (data not shown).
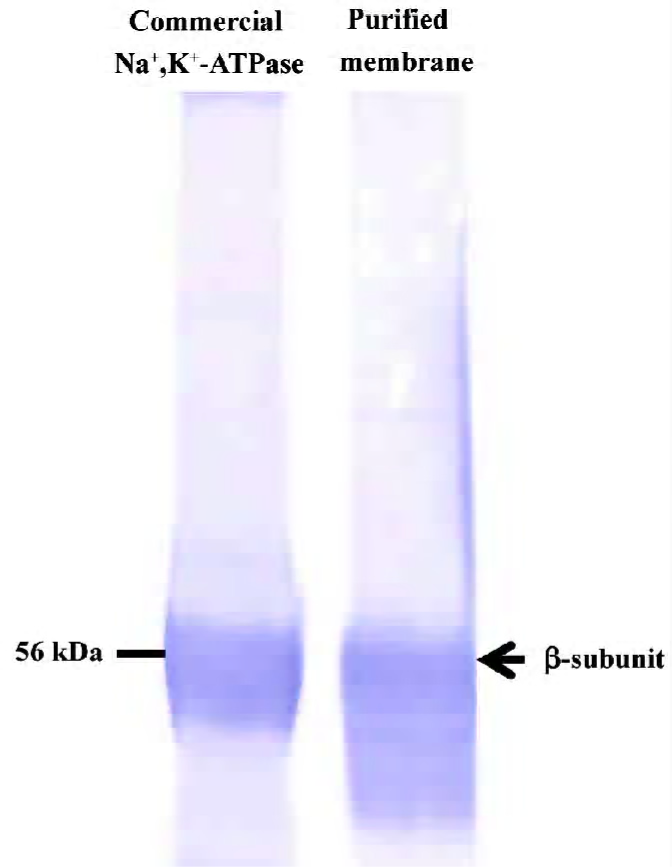
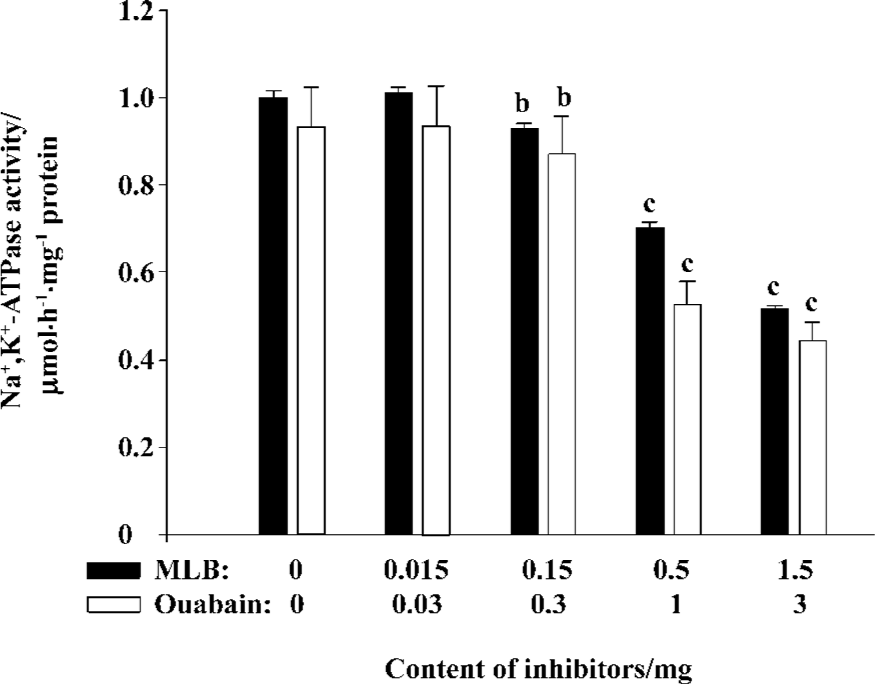
Neuroprotection of MLB on cerebral ischemic damage in gerbils Recent observations of the neuroprotection of cardiac glycosides against ischemic stroke[14] provoked an inquiry as to whether MLB could also act as anti-ischemic agents in vivo. To examine this possibility, gerbils were administered with 2 different concentrations of MLB after a focal cerebral ischemia. All the treated animals were found to have infarction in the cortex and caudate-putamen. The mean total infarct sizes, visualized by TTC staining (Figure 5), in the 3 groups (control, 6 mg MLB, and 0.6 mg MLB) were 17.35%±0.51%, 7.42%±1.40%, and 7.67%±2.35%, respectively (Figure 6). These results indicate that post-treatment with 6 mg or 0.6 mg MLB significantly reduced the infarct sizes of gerbil brains in cerebral ischemia by 57.3% and 55.8%, respectively (P<0.01).
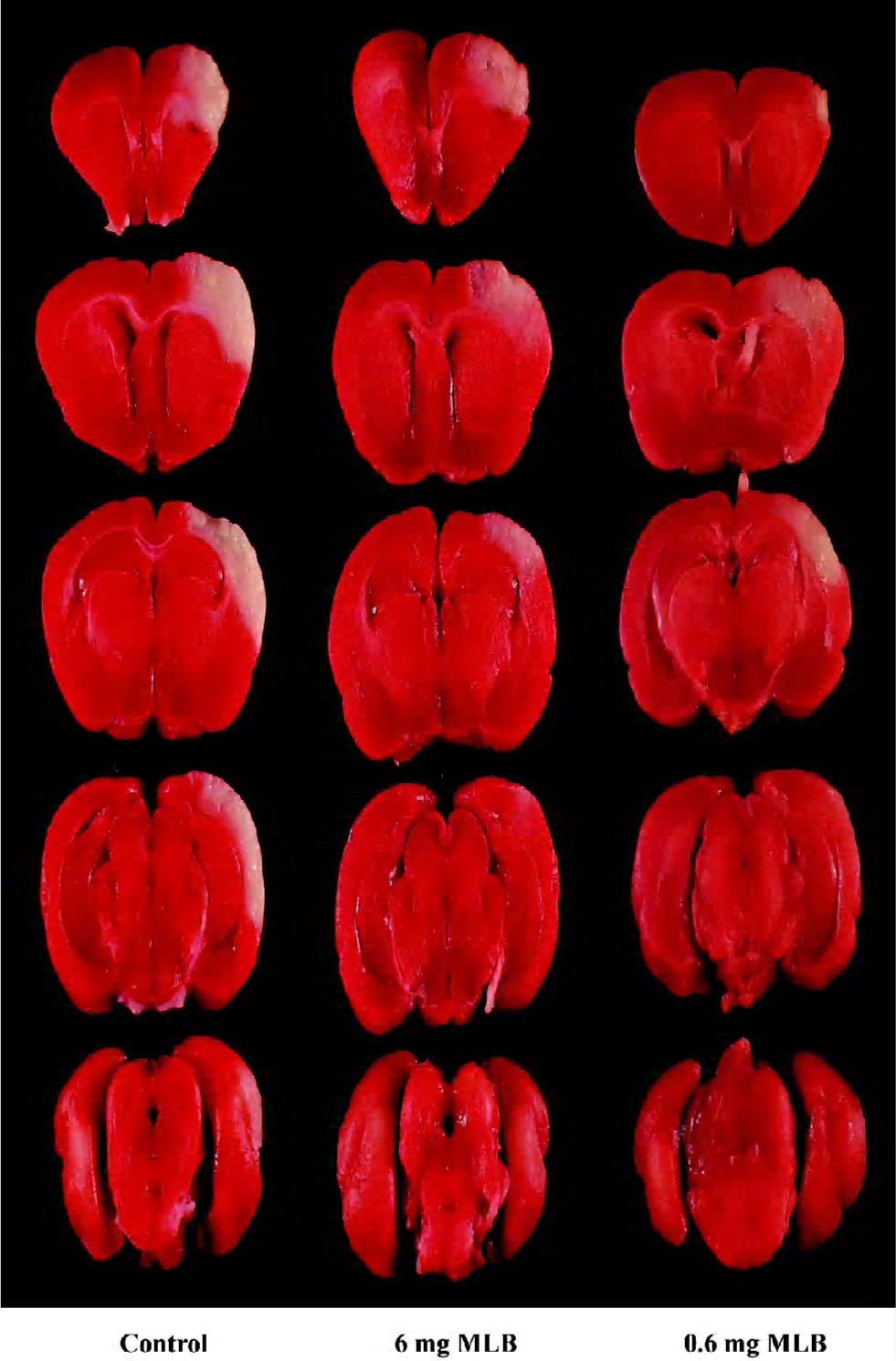
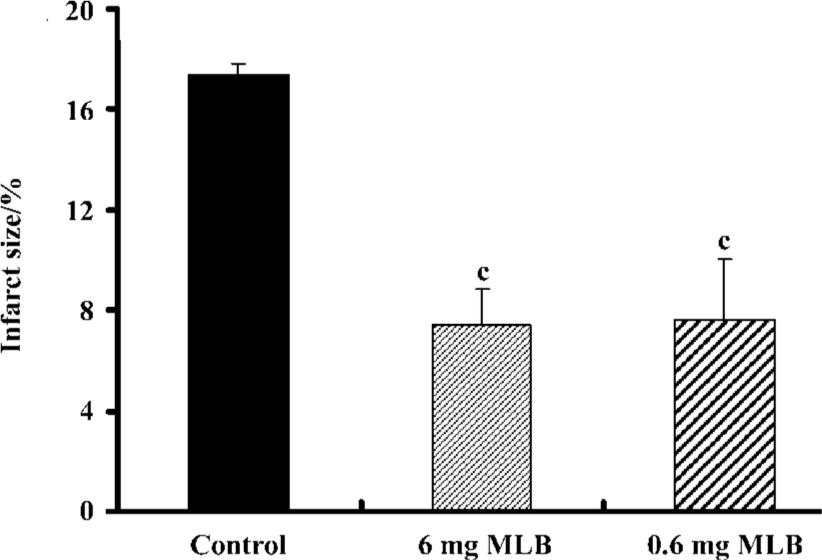
Discussion
Cardiac glycosides are drugs clinically used to relieve the symptoms of congestive heart failure[21]. Although these compounds unquestionably improve the conditions of patients, safe administration of these drugs has been regarded as a difficult task due to their narrow safety margin and severe side effects. Extensive efforts have been made to develop novel cardiotonic agents, such as new digitalis-like molecules through chemical synthesis and modification[21–25]. As these derivatives possess the same or similar steroid backbone, side-effects are unlikely to be eliminated. In this study, MLB extracted from Danshen, presumably partially mimicking the steroid structure of cardiac glycosides on the basis of its relatively rigid structure stabilized by the salt bridges formed between Mg2+ and carboxyl groups, was demonstrated to inhibit Na+,K+-ATPase more potently than ouabain in vitro. In contrast with the side effects of cardiac glycosides, MLB has been considered an antioxidant without significant adverse effects[2]. Therefore, we believe that MLB is of great potential to replace cardiac glycosides, after clinical trials, for the treatment of congestive heart failure.
It has been shown that cardiac glycosides inhibit Na+,K+-ATPase by binding to its α-subunit from the extracellular side[26]; however, the detailed binding site has not been illustrated. Based on molecular modeling and docking, 2 different locations in the α-subunit of Na+,K+-ATPase were proposed for ouabain binding. Cerri et al[27] proposed that ouabain penetrated into and bound with transmembrane regions, that is, both the extracellular loops of the α-subunit and the interprotodimeric cleft constituted by the transmembrane helices of both subunits. In contrast, Qiu et al[28] predicted that ouabain lay on the surface of the transmembrane ion channel without penetration, that is, ouabain was mainly located in the area surrounded by some extracellular loops of α-subunit and a few amino acid residues of the transmembrane helices exposed to the extracellular space. Based on the current study, we propose that MLB may trigger the same molecular mechanism responsible for the therapeutic effect of cardiac glycosides via the reversible inhibition on Na+,K+-ATPase. Further studies are indispensable to see if MLB and cardiac glycosides bind to the same or different region of Na+,K+-ATPase. Although the inhibition of MLB on Na+,K+-ATPase is possibly responsible for the cardiac therapeutic effect of Danshen, the molecular mechanism triggered by this inhibitory activity is unlikely responsible for other known medicinal effects of MLB, such as vasodilating, antihypertensive, antioxidative, and free radical scavenging activities[5–10].
In a recent study using a cortical brain slice-based compound screening platform, cardiac glycosides were demonstrated to provide neuroprotection against ischemic stroke[14]. In that work, neuroprotective activity and delayed therapeutic potential were observed for neriifolin as well as other cardiac glycosides in this brain slice assay model. The same phenomenon was observed when we examined the neuro-protective effect of MLB against ischemic stroke in a similar brain slice assay model (Figures 5, 6). Furthermore, the protective effects against cerebral ischemia-reperfusion injury and attenuation of the infarction area in a middle cerebral artery occlusion animal model by a mixture of total salvianolic acids extracted from Danshen were also reported previously[29]. Recent studies indicate that Mg2+ possesses a neuroprotec-tive effect on brain trauma in rats[30] and is capable of modulating the L-type calcium channel in the myocardium[31]. Since MLB is composed of 2 portions, an organic moiety of caffeic acid tetramer and the cation Mg2+, it is also possible that the Mg2+ salt-bridged against MLB may partly play a role to protect the brain against ischemic injury. It remains to be investigated whether cardiac glycosides and MLB exert neuro-protection against ischemic stroke via the same mechanism triggered by the inhibition of Na+,K+-ATPase.
Although blocking excitotoxicity-induced calcium overload of neurons after ischemic injury has been the focus of many therapeutic efforts, abnormally low levels of cytosolic calcium may cause the death of neuronal cells, as proposed in the “calcium set-point hypothesis”[32]. As suggested by Lee et al[33], calcium starvation and apoptosis may be the predominant causes of cell death in the penumbra, particularly at later time intervals. Therefore, it is possible that the neuroprotection of MLB against ischemic stroke may partly be a result of an increasing intracellular calcium level through the inhibition of Na+,K+-ATPase (A diagram was shown on the cover of this issue).
It is known that the biological mechanisms underlying brain damage during reperfusion subsequent to ischemia are presumably attributed to energy failure, free radical damage, and intracerebral synthesis of the platelet activating factor. It is possible that the neuroprotection of MLB observed in this study may have partly resulted from the reduction of ATP consumption via the inhibition of Na+,K+-ATPase, consistent with the concept of a defense strategy against hypoxia proposed by Hochachka[34]. Edema is one of the major complications of cerebral ischemia, being at the time an aggravating factor. MLB may limit the formation of cerebral edema and suppress its neurological changes. In brief, our results in the present study support the notion that MLB provides anti-ischemic neuroprotection in gerbils subjected to focal ischemia and reperfusion. Of course, identification of the signal transduction pathway downstream from the inhibition of Na+,K+-ATPase by MLB and assessment of other stimulatory effects of this potential drug in brain are also interesting and challenging tasks.
Acknowledgments
We thank Professor Chih-ning SUN for critical reading of the manuscript and Mr Chi-ming WU for assistance in preparation of the rat plasma membrane.
References
- Li XC, Yu C, Sun WK, Liu GY, Jia JY, Wang YP. Pharmacokinetics of magnesium lithospermate B after intravenous administration in beagle dogs. Acta Pharmacol Sin 2004;25:1402-7.
- Lu Y, Foo LY. Polyphenolics of Salvia–a review. Phytochemistry 2002;59:117-40.
- Tanaka T, Morimoto S, Nonaka G, Nishioka I, Yokozawa T, Chung HY, et al. Magnesium and ammonium-potassium lithospermates B, the active principles having a uremia-preventive effect from Salvia miltiorrhiza. Chem Pharm Bull 1989;37:340-4.
- Shigematsu T, Tajima S, Nishikawa T, Murad S, Pinnell SR, Nishioka I. Inhibition of collagen hydroxylation by lithospermic acid magnesium salt, a novel compound isolated from Salviae miltiorrhizae Radix. Biochim Biophys Acta 1994;25:79-83.
- O K. Lynn EG, Vazhappilly R, Au-Yeung KK, Zhu DY, Siow YL. Magnesium tanshinoate B (MTB) inhibits low density lipoprotein oxidation. Life Sci 2001;68:903-12.
- Yokozawa T, Lee TW, Oura H, Nonaka G, Nishioka I. Effect of magnesium lithospermate B in rats with sodium-induced hypertension and renal failure. Nephron 1992;60:460-4.
- Fung KP, Wu J, Zeng LH, Wong HN, Lee CM, Hon PM. Lithospermic acid B as an antioxidant-based protector of cultured ventricular myocytes and aortic endothelial cells of rabbits. Life Sci 1993;53:189-93.
- Yokozawa T, Chung HY, Dong E, Oura H. Confirmation that magnesium lithospermate B has a hydroxyl radical-scavenging action. Exp Toxicol Pathol 1995;47:341-4.
- Kasimu R, Tanaka K, Tezuka Y, Gong ZN, Li JX, Basnet P. Comparative study of seventeen Salvia plants: aldose reductase inhibitory activity of water and MeOH extracts and liquid chromatography-mass spectrometry (LC-MS) analysis of water extracts. Chem Pharm Bull 1998;46:500-4.
- Wu XJ, Wang YP, Wang W, Sun WK, Xu YM, Xuan LJ. Free radical scavenging and inhibition of lipid peroxidation by magnesium lithospermate B. Acta Pharmacol Sin 2000;21:855-8.
- Withering W. An account of the foxglove and some of its medical uses: with practical remarks on dropsy, and other diseases. London: GGJ and Robinson J; 1785.
- Li-Saw-Hee FL, Lip GY. Digoxin revisted. QJM 1998;91:259-64.
- Lin SC, Way EL. A high affinity Ca2+ ATP-ase enriched nerve-ending plasma membranes. Brain Res 1982;235:387-92.
- Wang JK, Portbury S, Thomas MB, Barney S, Ricca DJ, Morris DL, . Cardiac glycosides provide neuroprotection against ischemic stroke: Discovery by a brain slice-based compound screening platform. Proc Natl Acad Sci USA 2006; 103: 10 461–6.
- Wishart DS, Knox C, Guo AC, Shrivastava S, Hassanali M, Stothard P, et al. DrugBank: a comprehensive resource for in silico drug discovery and exploration. Nucleic Acids Res 2006;34:D668-72.
- Lin SC, Way EL. Characterization of calcium-activated and magnesium-activated ATP-ase of brain nerve endings. J Neuro-chem 1984;42:1697-706.
- Muszbek L. A highly sensitive method for the measurement of the ATPase activity. Anal Biochem 1977;77:286-8.
- Goldberg H, Fernander A. Simplified method of the estimation of inorganic phosphorus in body fluids. Clin Chem 1996;12:871-5.
- Yang DY, Tsai TH, Cheng CH, Lee CW, Chen SH, Cheng FC. Simultaneous monitoring of extracellular glucose, pyruvate, lactate and glutamate in gerbil cortex during focal cerebral ischemia by dural probe microdialysis. J Chromatogr A 2001;913:349-54.
- Bederson JB, Pitts LH, Germano SM, Nishmura MC, Davis RL, Bartkowski HM. Evaluation of 2,3,5-triphenyltetrazolium chloride as a stain for detection and quantification of experimental cerebral infarction in rats. Stroke 1986;17:1304-8.
- Kjeldsen K, Norgaard A, Gheorghiade M. Myocardial Na,K-ATPase: the molecular basis for the hemodynamic effect of digoxin therapy in congestive heart failure. Cardiovasc Res 2002;55:710-3.
- Quadri L, Cerri A, Ferrari P, Folpini E, Mabilia M, Melloni P. Synthesis and structure-activity relationships of 17-(hydrazono-methyl)-5-androstane-3,14-diol derivatives that bind to Na+,K+-ATPase receptor. J Med Chem 1996;39:3385-93.
- De Munari S, Barassi P, Cerri A, Fedrizzi G, Gobbini M, Mabilia M, et al. New approach to the design of novel inhibitors of Na+,K+-ATPase: 17-substituted seco-D 5-androstane as cassaine analogues. J Med Chem 1998;41:3033-40.
- Gobbini M, Barassi P, Cerri A, De Munari S, Fedrizzi G, Santagostino M, et al. 17-O-aminoalkyloxime derivatives of 3, 14-dihydroxy-5-androstane and 3-hydroxy-14-oxo-seco-D-5-androstane as inhibitors of the digitalis receptor on Na+,K+-ATPase. J Med Chem 2001;44:3821-30.
- Cerri A, Almirante N, Barassi P, Benicchio A, De Munari S, Marazzi G, et al. Synthesis and inotropic activity of 1-(O-aminoalkyloximes) of perhydroindene derivatives as simplified digitalis-like compounds acting on the Na+,K+-ATPase. J Med Chem 2002;45:189-207.
- Ball WJJ, Farr CD, Paula S, Keenan SM, Delisle RK, Welsh WJ. Three-dimensional structure-activity relationship modeling of digoxin inhibition and docking to Na+,K+-ATPase. Ann N Y Acad Sci 2003;986:296-7.
- Cerri A, Almirante N, Barassi P, Benicchio A, Fedrizzi G, Ferrari P, et al. 17 -O-aminoalkyloximes of 5-androstane-3,14-diol with digitalis-like activity: synthesis, cardiotonic activity, structure-activity relationships and molecular modeling of the Na+,K+-ATPase receptor. J Med Chem 2000;43:2332-49.
- Qiu LY, Koenderink JB, Swarts HG, Willems PH, De Pont JJ. Phe, Thr, and Asp in transmembrane hairpin M5-M6 of Na,K-ATPase play a key role in ouabain binding. J Biol Chem 2003; 278: 47 240–4.
- Jiang RW, Lau KM, Hon PM, Mak TC, Woo KS, Fung KP. Chemistry and biological activities of caffeic acid derivatives from Salvia miltiorrhiza. Curr Med Chem 2005;12:237-46.
- Johnson EM Jr, Deckwerth TL. Molecular mechanisms of developmental neuronal death. Annu Rev Neurosci 1993;16:31-46.
- Browne KD, Leoni MJ, Iwata A, Chen XH, Smith DH. Acute treatment with MgSO4 attenuates long-term hippocampal tissue loss after brain trauma in the rat. J Neurosci Res 2004;77:878-83.
- Wang M, Berlin JR. Channel phosphorylation and modulation of L-type Ca2+ currents by cytosolic Mg2+ concentration. Am J Physiol Cell Physiol 2006;291:C83-92.
- Lee JM, Grabb MC, Zipfel GJ, Choi DW. Brain tissue responses to ischemia. J Clin Invest 2000;106:723-31.
- Hochachka PW. Defense strategies against hypoxia and hypothermia. Science 1986;231:234-41.