C16, a novel advanced glycation endproduct breaker, restores cardiovascular dysfunction in experimental diabetic rats1
Introduction
Glucose and other reducing sugars react with proteins by a series of reactions to form a class of heterogeneous, nonenzymatic sugar-amino adducts that are called advanced glycation endproducts (AGE)[1,2]. Numerous studies have indicated that the formation of AGE in long-lived connective tissue and matrix components is a causative factor in the development of diabetic complications and diseases associated with aging[3–6]. In the cardiovascular system, the accumulation of AGE on structural tissue proteins is one of the main mechanisms underlying cardiovascular stiffness[7,8]. Recently, a number of natural or synthetic compounds that target AGE, including AGE inhibitors and breakers, have been discovered and are being further developed[9–11]. Amino-guanidine (AG) was the first compound designed to inhibit AGE formation and cross-linking in vitro and in vivo, and is currently undergoing phase III clinical trials[12,13]. ALT-711, a well-known AGE breaker, has also been reported to be effective in in vitro and animal studies, and is currently undergoing phase II clinical trials[14–19]. Therefore, treatment targeting AGE is believed to be a potential effective therapeutic option for cardiovascular dysfunction[10].
Based on the hypothesis that AGE crosslinks could be cleaved with N-phenacylthiazolium bromide[20], the lead compound ALT-711, novel AGE breakers were synthesized in our laboratory by using computer-aided drug design. Preliminary biological screening tests demonstrated that 3-[2-(4-bromo-phenyl)-1-methyl-2-oxo-ethyl]-4,5,6,7-tetrahydro-benzothiazol-3-ium bromide (C16; Figure 1) had the ability to break AGE crosslinks in vitro[21]. C16 produced a concentration-dependent release of bovine serum albumin (BSA) from preformed AGE-modified BSA (AGE-BSA)-collagen complexes and C16 treatment decreased the red blood cell (RBC)-immunoglobulin G (IgG) crosslinks (unpublished data). Therefore, the aim of the present study was to investigate the effects of C16 on the cardiovascular system in experimental diabetic rats. Furthermore, the action site of C16 in vivo was explored by comparison with that of ALT-711.
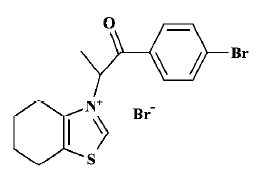
Materials and methods
Reagents and compounds 3-[2-(4-Bromo-phenyl)-1-methyl-2-oxo-ethyl]-4,5,6,7-tetrahydro-benzothiazol-3-ium bromide (C16), whose structure (Figure 1) was identified by nuclear magnetic resonance spectroscopy-mass spectroscopy and elemental analysis, and ALT-711 were synthesized at the Beijing Institute of Pharmacology and Toxicology, as described previously[21]. Streptozotocin (STZ) was purchased from Sigma. AGE antibody was kindly donated by the Beijing Institute of Radiation Medicine. All other chemicals and substances were of analytical grade unless stated otherwise.
Animals Diabetes was induced in 9-10-week-old male Wistar rats by ip injection of 70 mg/kg of STZ after an overnight fast. Only animals that developed blood glucose levels >15 mmol/L were used. After 12 weeks of diabetes, the animals were used for studies. For the hemodynamic study of the left ventricle, diabetic rats were divided into 4 groups (8 rats in each group) to assess the exact hemodynamic changes in the left ventricle (LV) that were caused by the diabetic state. Rats were given either vehicle or 25 mg/kg per day of ALT-711, or 25 or 50 mg/kg per day of C16 (ig) for 4 weeks. In another hemodynamic study, 6 groups of diabetic rats (n=8) received either vehicle or 12.5 mg/kg per day of ALT-711, or 12.5, 25 or 50 mg/kg per day of C16 (ig) for 4 weeks, or 50 mg/kg per day of C16 (ig) for 2 weeks to assess the possible reversal of diabetes-induced cardiovascular abnormalities. ALT-711 and C16 were dissolved in distilled water immediately before administration. An additional group of age-matched nondiabetic rats served as normal controls, and were observed in parallel for each study.
Hemodynamic study of the left ventricle Details regarding the surgical procedure and hemodynamic measurements have been described elsewhere[22]. In summary, animals were anesthetized with 50 mg/kg of pentobarbital (ip). A fluid-filled catheter was introduced through the right carotid artery into the left ventricle. Tracings of LV pressure were digitized at a rate of 2000 samples/s with a commercially available analog-to-digital converter (MP150WS, BIOPAC Systems) and a personal computer using dedicated software (Acknowledge, Version 3, BIOPAC Systems). The digitized LV pressure recording was used to calculate the maximal rate of pressure rise (+dp/dtmax) and the maximal rate of pressure fall (-dp/dtmax).
Hemodynamic study of cardiovascular system After animals were anesthetized with 50 mg/kg of pentobarbital (ip), a midsternal thoracotomy was performed, and the ascending aorta was dissected free. The pressure transducer was advanced into the ascending aorta. An adapted Doppler probe was positioned around the vessel to measure phasic aortic blood flow. The system was allowed to stabilize for 10 min before aortic blood flow and pressure were digitized at a rate of 2000 samples/s with a commercially available analog-to-digital converter and a personal computer using dedicated software. All parameters were calculated on a beat-to-beat basis for 30 s and then averaged. In steady-state conditions, measurements were obtained of systolic and diastolic blood pressure (SBP, DBP), cardiac output (CO), and heart rate (HR). Total peripheral resistance (TPR) was determined as the quotient of mean arterial blood pressure and CO[23]. Systemic arterial compliance (SAC) was calculated from the quotient of stroke volume and pulse pressure[24].
RBC-IgG assay Detailed methods have been described elsewhere[14]. Briefly, blood samples were collected, before hemodynamic studies of the left ventricle and RBC-IgG determinations were performed by using an anti-IgG enzyme-linked immunosorbent assay (ELISA) adapted for use with cellulose ester membrane-sealed 96-well microtiter plates (Multiscreen-HA, Millipore). Heparinized blood was washed 3 times with phosphate-buffered saline (PBS), then the packed RBC were diluted 1:250−1:500 in PBS. Membrane-containing wells were blocked with 0.3 mL Superblock (Pierce), then washed with 0.3 mL PBS/0.05% Tween, followed by 0.1 mL PBS. RBC were gently vortexed and 50 µL aliquots were pipetted into wells. Cells were then washed, and 50 µL of a polyclonal rabbit anti-rat IgG (Sigma, diluted 1:25 000) was added. After incubation at room temperature for 2 h, the cells were washed 3 times with PBS, once with Tris-buffered saline, and 0.1 mL of p-nitrophenyl phosphate substrate was added (1 mg/mL in 0.1 mol/L diethanolamine buffer, pH 9.5). The plates were read in a microplate reader (Bio-Rad 550) at 410 nm. The content of RBC-IgG was expressed as OD410.
Tail tendon collagen solubility assay The solubility of tail tendon collagen was measured by using a previously reported method with modifications[25]. Briefly, after performing a hemodynamic study on the left ventricle, the rats’ tails were removed and the tail tendon was removed by gentle pulling. The tendons were cleaned of debris and fat in 0.9% NaCl over ice. The tendons were rolled into a ball, patted dry on paper towels, then lyophilized. Following lyophilization, tail tendons were stored at -70 oC in desiccated sealed containers until use. Collagen samples (2 mg) were weighed and digested with pepsin (5.0 µg pepsin/mg collagen in 0.5 mol/L acetic acid) for 2 h at 4 °C. After digestion, the samples were centrifuged at 40 000×g for 60 min at 4 oC. The supernatant was collected and both the volume of the supernatant and pellet were determined. Aliquots (500 µL) of the supernatant and all of the pellets were acid hydrolyzed and analyzed for their hydroxyproline content[26], which was assumed to make up 14% of the collagen by weight. The recoverable collagen was defined as the sum of collagen in the supernatant and pellet after digestion and percentage solubility was defined as the amount of collagen in the supernatant fraction in relation to the total recoverable collagen.
Morphological study of arterial collagen distribution After performing the hemodynamic study on the rats’ cardiovascular systems, 2 to 3 cm segments from the rats’ descending thoracic aortas were fixed in 10% formalin in saline, and embedded in paraffin for morphological and immunohistochemical studies. Seven-micron sections of aorta were stained with picrosirius red (Direct Red 80, Aldrich, in aqueous picric acid) for 4 h. The collagen type III/I ratio for the aortic media wall was measured by using polarizing light microscopy (Nikon, E600POL) according to previously published methods[27,28].
Immunohistochemistry for AGE Four-micron sections of aorta were used for AGE staining. Briefly, the sections were rehydrated and treated with 3% H2O2/methanol followed by incubation in blocking buffer (Superblock, Pierce) for 20 min at room temperature. The sections were then incubated with the anti-AGE antibody (diluted 1:100) for 2 h at room temperature, washed in PBS, and incubated with goat anti-rabbit IgG/horse radish peroxidase (Zymed). The staining was visualized by reaction with diaminobenzidine tetrahydrochloride (Sino-American Biotechnology).
Statistical analysis All results are expressed as mean±SD. Statistical analysis was performed by one-way ANOVA analysis with SPSS. P<0.05 was considered statistically significant.
Results
Hemodynamic study of the left ventricle Left ventricular systolic pressure (LVSP), +dp/dtmax, and -dp/dtmax were decreased significantly (P<0.01 vs normal control) in the vehicle-treated diabetic group (Table 1). C16 treatment did not result in significant weight or fasting blood glucose levels changes (P>0.05 vs vehicle-treated diabetic rats). However, treatment with C16 (25 or 50 mg/kg) for 4 weeks resulted in a significant increase in all of these 3 parameters (P<0.05 or P<0.01, Table 1) as compared with vehicle-treated diabetic rats. There was no difference between C16-treated groups (25 or 50 mg/kg) or between the C16-treated groups and the ALT-711-treated group (25 mg/kg).
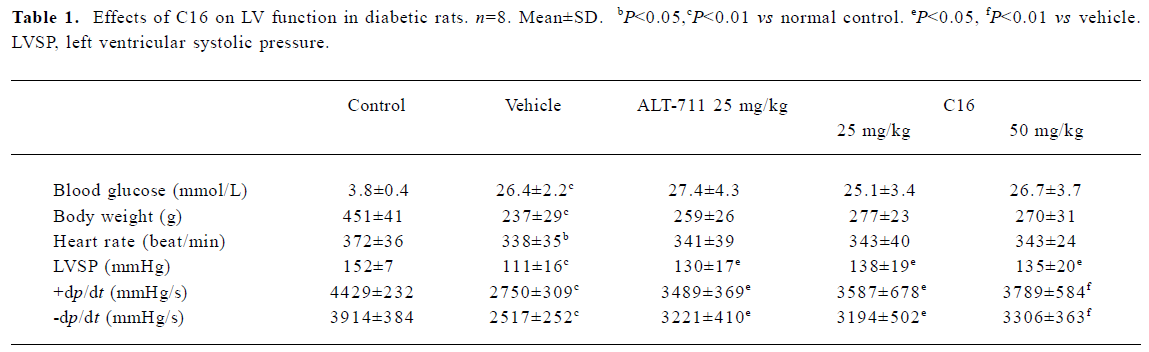
Full table
Hemodynamic study of the cardiovascular system In comparison with the normal controls, the body weights and HR of the vehicle-treated diabetic rats were lower (P<0.01 and P<0.05, respectively), whereas the TPR and TPR index were significantly higher (P<0.01). CO and SAC were significantly lower (P<0.01 vs normal control) in vehicle-treated diabetic rats. SBP, DBP, and HR were not significantly different in the 6 groups of diabetic rats (Table 2). Treatment with C16 for 4 weeks resulted in a dose-dependent significant increase in CO and the CO index (P<0.05 or P<0.01), a reduction in TPR and the TPR index (P<0.05 or P<0.01), and an increase in SAC (P<0.05 or P<0.01) as compared with the vehicle-treated rats. After 4 weeks of treatment, similar results were found in ALT-711-treated (12.5 mg/kg) rats. The effects of C16 on the cardiovascular system resembled those of ALT-711. Treatment with C16 (50 mg/kg) for 2 weeks produced values that were slightly different from those produced by the other treatment regimen. Although the difference did not reach statistical significance for the majority of the parameters, SAC was significantly increased (P<0.01 vs vehicle group).
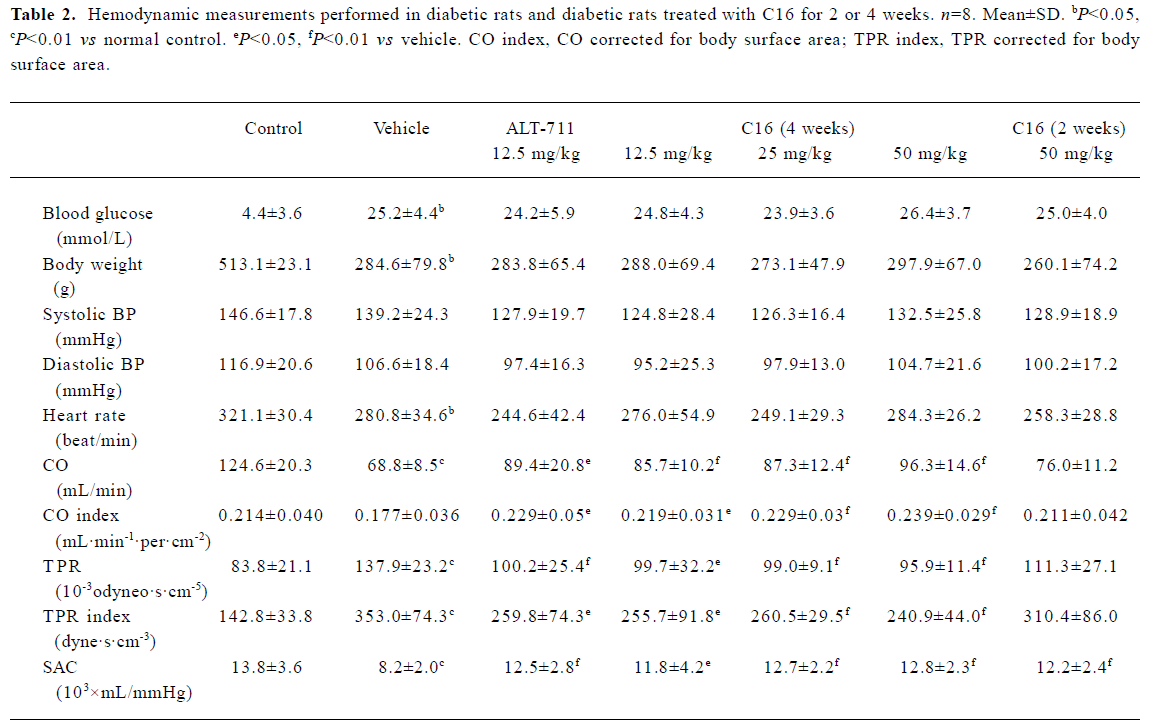
Full table
RBC-IgG assay The RBC-IgG content of normal control rats was 0.21±0.01 and the content of vehicle-treated diabetic rats was 0.61±0.04 (n=6; P<0.01, Figure 2). Treatment with C16 (25 or 50 mg/kg) resulted in a significant reduction of RBC-IgG content (0.51±0.06, 0.41±0.06; P<0.05, P<0.01, respectively) in comparison with vehicle-treated diabetic rats. ALT-711 treatment (25 mg/kg) also significantly reduced RBC-IgG (0.48±0.07, P<0.01).
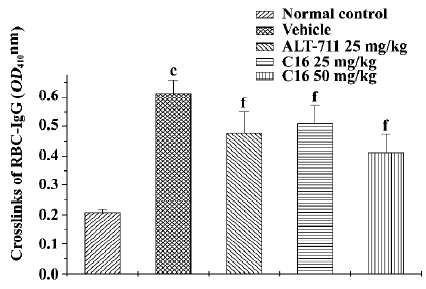
Collagen solubility assay In comparison with normal control animals, the tail tendon collagen solubility of the vehicle-treated animals tended to fall (from 63.9%±7.3% to 37.7%±10.0%, P<0.01; Figure 3). Compared with vehicle-treated animals, collagen solubility was increased significantly after treatment with C16 (25 mg/kg, 48.0%± 9.0%; 50 mg/kg, 56.8%±7.4%; P<0.05, P<0.01, respectively). ALT-711 treatment resulted in a significant increase in collagen solubility (57.7%±6.2%, P<0.01).
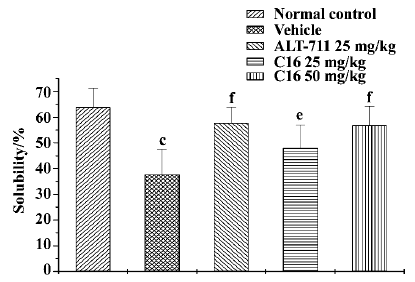
Morphological study of arterial collagen distribution When stained with picrosirius red, different types of collagen in the aortic media wall could be distinguished by polarizing light microscopy, where type I collagen appeared yellow or yellow-red, and type III collagen appeared green (Figure 4). The collagen type III/I ratio of the aortic media wall tended to be greater in rats with diabetes (Figure 4B), but C16 (50 mg/kg) and ALT-711 (12.5 mg/kg) treatment could reverse this alteration (Figure 4C, 4D).
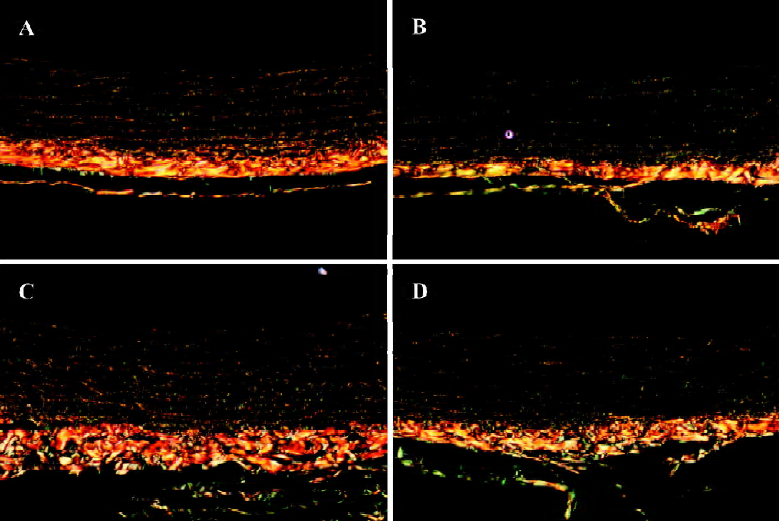
Immunohistochemistry for AGE In comparison with normal control animals, the amount of AGE accumulated in the aortic media wall of the vehicle-treated animals was increased. But the amount of AGE was decreased by C16 (50 mg/kg) and ALT-711 (12.5 mg/kg) treatment (Figure 5).
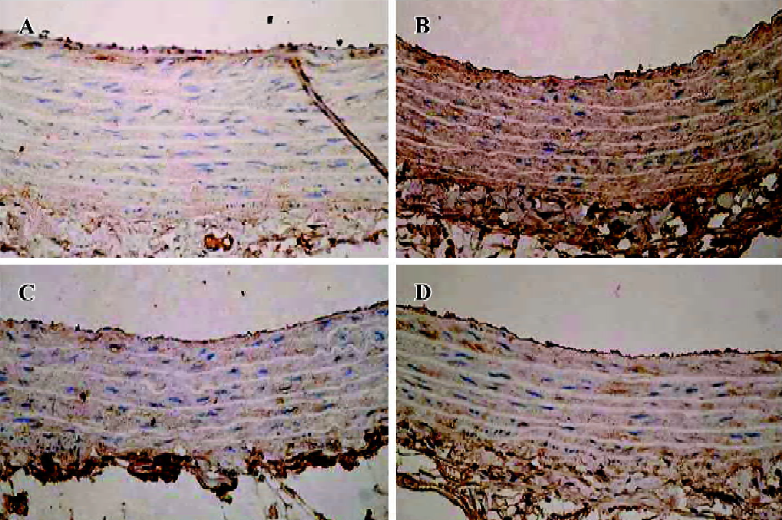
Discussion
Nearly a century ago, glycation was first recognized in the food industry, and became known as the Maillard reaction: a process in which food proteins crosslink and become brown with age. In the 1980s, Brownlee et al first described the harmful consequences of AGE formation on the cardiovascular and renal systems in humans[1,29] and diabetic rats[13]. Recently, it has been thought more and more likely that AGE and AGE crosslinks are linked to the development of many age- and diabetes-related disorders through structural modifications as well as receptor-mediated path-ways, which activate growth factors, induce a number of processes, and initiate inflammatory reactions[30]. Therefore, targeting AGE, especially breaking established AGE cross-links, was considered to be a novel and promising therapeutic candidate for reversing AGE-related pathologic conditions. In the present study, rats with STZ-induced diabetes of 16 weeks duration exhibited a marked increase in AGE and an abnormal distribution of collagen type in the aorta. However, significant decreases in hemodynamic parameters, such as LV dp/dt, CO, and SAC, were also observed. These results demonstrated that AGE-related changes in structure eventually increased the stiffness of the arterial tree and myocardium, which, in turn, resulted in functional changes. Immunohistochemical assays revealed that C16, a potential AGE breaker, could prevent the increase of AGE accumulation in the aortic media wall of diabetic rats, and could reverse the increase in the collagen type III/I ratio (prior studies have generally shown an association between increased collagen type III and/or the III/I ratio and the accumulation of AGE cross-links[31,32]). Furthermore, both the diastolic function, as indicated by -dp/dtmax, and the contractile function of LV, as indicated by LVSP and +dp/dtmax, were restored significantly by C16. The significant improvements in the hemodynamic parameters could not be attributed to differences in blood pressure, which did not change significantly during treatment as compared with the vehicle-treated diabetic group. Therefore, the improvements reflect intrinsic modifications of the mechanical properties of the arterial wall. The increase in SAC and the decrease in TPR indicates that through treatment with C16 the stiffness of the aorta was reduced to levels comparable to those observed in normal control rats. Moreover, these effects seemed to be related to the duration of the treatment, with stronger effects after 4 weeks than 2 weeks, which indicates that the effects of C16 were exerted through the pathway of structural modification by slowly breaking the established AGE crosslinks. The different approaches used in the present study consistently show that C16, a novel AGE crosslink breaker, exerted beneficial cardiovascular actions and restored diabetes-associated cardiovascular dysfunction in experimental diabetic rats by reducing AGE, and that C16 has similar effects to ALT-711, the well-known AGE breaker.
The presence of AGE crosslinks is thought to contribute to increased insolubility and resistance of collagen to enzymatic and chemical digestion[25], and IgG crosslinked to RBC as a structure of AGE crosslinks is formed earlier than other AGE crosslinks in vivo[14]. Thus, the susceptibility of collagen to digestion by pepsin and the IgG-RBC crosslink content have previously been used to provide 2 indexes of protein crosslinking in vivo[13,20,25]. In the present study, the considerably improved solubility of collagen and decreased content of IgG crosslinked to RBC after treatment with C16 demonstrated that C16 could reduce AGE crosslinks in vivo.
In summary, the novel compound C16 has the ability to break established AGE crosslinks and reduce AGE accumulation in tissues in vivo. Furthermore, C16 can restore diabetes-associated cardiovascular dysfunction in rats. This provides a potential therapeutic approach for diabetes- and aging-related cardiovascular disease.
References
- Brownlee M, Cerami A, Vlassara H. Advanced glycosylation end products in tissue and the biochemical basis of diabetic complications. N Engl J Med 1988;318:1315-21.
- Kass DA. Getting better without AGE: new insights into the diabetic heart. Circ Res 2003;92:704-6.
- Brownlee M. Lilly Lecture 1993. Glycation and diabetic complications. Diabetes 1994;43:836-41.
- Singh R, Barden A, Mori T, Beilin L. Advanced glycation end-products: a review. Diabetologia 2001;44:129-46.
- Brownlee M. The pathological implications of protein glycation. Clin Invest Med 1995;18:275-81.
- Bucala R, Cerami A. Advanced glycosylation: chemistry, biology, and implications for diabetes and aging. Adv Pharmacol 1992;23:1-34.
- Sims TJ, Rasmussen LM, Oxlund H, Bailey AJ. The role of glycation cross-links in diabetic vascular stiffening. Diabetologia 1996;39:946-51.
- Avendano GF, Agarwal RK, Bashey RI, Lyons MM, Soni BJ, Jyothirmayi GN, et al. Effects of glucose intolerance on myocardial function and collagen-linked glycation. Diabetes 1999;48:1443-7.
- Rahbar S, Figarola JL. Novel inhibitors of advanced glycation endproducts. Arch Biochem Biophys 2003;419:63-79.
- Vasan S, Foiles P, Founds H. Therapeutic potential of breakers of advanced glycation end product-protein crosslinks. Arch Biochem Biophys 2003;419:89-96.
- Dukic-Stefanovic S, Schinzel R, Riederer P, Munch G. AGES in brain ageing: AGE-inhibitors as neuroprotective and anti-dementia drugs? Biogerontology 2001;2:19-34.
- Li YM, Steffes M, Donnelly T, Liu C, Fuh H, Basgen J, et al. Prevention of cardiovascular and renal pathology of aging by the advanced glycation inhibitor aminoguanidine. Proc Natl Acad Sci USA 1996;93:3902-7.
- Brownlee M, Vlassara H, Kooney A, Ulrich P, Cerami A. Aminoguanidine prevents diabetes-induced arterial wall protein cross-linking. Science 1986;232:1629-32.
- Wolffenbuttel BH, Boulanger CM, Crijns FR, Huijberts MS, Poitevin P, Swennen GN, et al. Breakers of advanced glycation end products restore large artery properties in experimental diabetes. Proc Natl Acad Sci USA 1998;95:4630-4.
- Asif M, Egan J, Vasan S, Jyothirmayi GN, Masurekar MR, Lopez S, et al. An advanced glycation endproduct cross-link breaker can reverse age-related increases in myocardial stiffness. Proc Natl Acad Sci USA 2000;97:2809-13.
- Vaitkevicius PV, Lane M, Spurgeon H, Ingram DK, Roth GS, Egan JJ, et al. A cross-link breaker has sustained effects on arterial and ventricular properties in older rhesus monkeys. Proc Natl Acad Sci USA 2001;98:1171-5.
- Liu J, Masurekar MR, Vatner DE, et al. Glycation end-product cross-link breaker reduces collagen and improves cardiac function in aging diabetic heart. Am J Physiol Heart Circ Physiol 2003;285:H2587-91.
- Susic D, Varagic J, Ahn J, Frohlich ED. Cardiovascular and renal effects of a collagen cross-link breaker (ALT 711) in adult and aged spontaneously hypertensive rats. Am J Hypertens 2004;17:328-33.
- Kass DA, Shapiro EP, Kawaguchi M, Capriotti AR, Scuteri A, deGroof RC, et al. Improved arterial compliance by a novel advanced glycation end-product crosslink breaker. Circulation 2001;104:1464-70.
- Vasan S, Zhang X, Zhang X, Kapurniotu A, Bernhagen J, Teichberg S, et al. An agent cleaving glucose-derived protein crosslinks in vitro and in vivo. Nature 1996;382:275-8.
- Li S, Cui H, Wang LL, inventors. New substituted penta azacyclo salt kind compound and its use in treating protein ageing related disease. CN patent 1534027. 2004 Oct 6.
- Yamamoto K, Masuyama T, Sakata Y, Nishikawa N, Mano T, Yoshida J, et al. Myocardial stiffness is determined by ventricular fibrosis, but not by compensatory or excessive hypertrophy in hypertensive heart. Cardiovasc Res 2002;55:76-82.
- Levy BI, Duriez M, Phillipe M, Poitevin P, Michel JB. Effect of chronic dihydropyridine (isradipine) on the large arterial walls of spontaneously hypertensive rats. Circulation 1994;90:3024-33.
- Yin FC, Guzman PA, Brin KP, et al. Effect of nitroprusside on hydraulic vascular loads on the right and left ventricle of patients with heart failure. Circulation 1983;67:1330-9.
- Kochakian M, Manjula BN, Egan JJ. Chronic dosing with aminoguanidine and novel advanced glycosylation end product-formation inhibitors ameliorates cross-linking of tail tendon collagen in STZ-induced diabetic rats. Diabetes 1996;45:1694-700.
- Stegemann H, Stalder K. Determination of hydroxyproline. Clin Chim Acta 1967;18:267-73.
- Junqueira LC, Cossermelli W, Brentani R. Differential staining of collagens type I, II and III by Sirius Red and polarization microscopy. Arch Histol Jpn 1978;41:267-74.
- Whittaker P, Kloner RA, Boughner DR, Pickering JG. Quantitative assessment of myocardial collagen with picrosirius red staining and circularly polarized light. Basic Res Cardiol 1994;89:397-410.
- Brownlee M, Cerami A, Vlassara H. Advanced products of nonenzymatic glycosylation and the pathogenesis of diabetic vascular disease. Diabetes Metab Rev 1988;4:437-51.
- Cooper ME. Importance of advanced glycation end products in diabetes-associated cardiovascular and renal disease. Am J Hypertens 2004;17:31S-8S.
- Shimizu M, Umeda K, Sugihara N, Yoshio H, Ino H, Takeda R, et al. Collagen remodelling in myocardia of patients with diabetes. J Clin Pathol 1993;46:32-6.
- Bruel A, Oxlund H. Changes in biomechanical properties, composition of collagen and elastin, and advanced glycation end products of the rat aorta in relation to age. Atherosclerosis 1996;127:155-65.