Two ligands for a GPCR, proton vs lysolipid1
Introduction
G-protein-coupled receptor (GPCR) is the largest gene family of human genome. GPCR is glaringly obvious by the fact that more than 50% of drugs on the market are either agonists or antagonists on GPCRs[1]. Positive or negative modulation of GPCRs with drugs has been successful tools to treat many diseases such as allergy, gastric ulcer, and hypertension. The common structural feature of all GPCRs is a seven-helical transmembrane region. GPCR activations are evoked by stimuli as diverse as light, Ca2+, odorants, amino acids, nucleotides, proteins, polypeptides, steroids, and fatty acid derivatives.
The completion of the human genome project has identified about 865 GPCR genes[2]. Except sensory receptors, 367 GPCRs have been considered as receptors for endogenous ligands in the human genome[3]. However, identification of novel members of GPCRs by genome sequencing faces orphan receptor problem, that is, ligands are not yet found[1]. About 150 orphan GPCRs are waiting for discovery of their ligands[4]. Pairing orphan GPCRs with their own ligands (endogenous or surrogate) would advance scientific know-ledge and induces discovery of new drugs[5–9]. However, recent double-pairing of OGR1 subfamily GPCRs with two different chemicals, proton and lysolipid, raises several questions.
First, whether both chemicals are the real ligands for OGR1 subfamily. Second, whether modulation of a GPCR by two chemicals could be possible, because classic pharmacological concept is one ligand for one GPCR. Third, one of the chemicals is proton. Although it has been well established for ion channel receptors such as transient receptor potential/vanilloid receptor subtype-1 (TRPV1)[10] and acid-sensing ion channels (ASICs)[11], in GPCR area, not only proton-sensing is a new action mode of GPCR activation, but also it could be generalized in other GPCRs.
OGR1 subfamily and lysolipids
OGR1 subfamily is composed of four members (OGR1, GPR4, G2A, and TDAG8) and has previously been identified as receptors for lysolipids; sphingosylphosphorylcholine (SPC), lysophosphatidylcholine (LPC) and psychosine (galactosylsphingosine)[12–14]. In 2000, Xu et al reported Ovarian cancer G-protein-coupled Receptor 1(OGR1, GPR68) to be a high-affinity receptor for SPC (Kd = 33 nmol/L) and SPC was shown to inhibit cell growth of OGR1-transfected HEK293 as well as various ovarian cancer cell lines[12,15]. In 2001, Zhu et al reported GPR4 to be the second high affinity receptor for SPC and GPR4 was shown to be activated by structurally-related LPC[13]. However, in contrast to OGR1, GPR4 activation stimulated cell growth and cell migration of GPR4-transfected Swiss3T3 cells[13]. TDAG8 (T-cell death-associated gene 8, GPR65) was reported to be activated by psychosine and its activation was shown to result in multinuclear cell formation[14]. G2A (G2 accumulation protein, GPR132), the last member of the subfamily, was characterized to cause cell cycle arrest in the G2/M phase[16]. LPC was initially reported as a ligand of G2A and T cell chemotaxis to LPC was shown to be mediated through G2A[17].
Yan et al reported macrophage chemotaxis to LPC is dependent on G2A function and mutation of the conserved DRY motif of G2A results in loss of function[18]. Wang et al demonstrated that murine G2A was spontaneously internalized and accumulated in endosomal compartments, whereas its surface expression was enhanced and stabilized by LPC treatment[19]. Han et al reported G2A-mediated up-regulation of CXCR4 in human helper T cells[20]. Murakami et al reported G2A-dependent actin stress fiber formation and its inhibition by LPC in G2A-NIH3T3 cells[21]. Also LPC enhances dose-dependently intracellular cAMP accumulation and G2A-induced apoptosis in Hela cells[22]. Ikeno et al reported that secretary phospholipase A2 induce neurite outgrowth in PC12 cells through LPC generation and activation of G2A receptor[23]. Lin and Ye reported that G2A displays a significant level of intrinsic signaling via Gαq, Gαs, and Gα13 pathways[22].
Lum et al showed that inflammatory stress increases GPR4 expression and LPC binding in human microvascular endothelial cells[24]. Recently, Kim et al reported that GPR4 plays a critial role in SPC-induced angiogenesis and SPC trans-activates VEGF receptor 2 in endothelial cells[25].
Maghazachi et al reported that psychosine and glucosylsphingosine induce multinuclear cell formation and apoptosis in TDAG8-expressing natural killer cells[26]. Malone et al reported that activation of TDAG8 by psycho-sine enhanced dexamethasone-induced apoptosis in a TDAG8-dependent manner in lymphomas[27]. Tosa et al reported critical function of TDAG8 in glucocorticoid-induced thymocyte apoptosis[28].
These ligand chemicals have similar lysolipid structures (Figure 1) and their significance in pathological conditions and pharmacological application has been discovered. Especially, G2A deficient mouse developed an autoimmune syndrome similar to systemic lupus erythematosus (SLE)[29], and therapeutic application of LPC for sepsis was proposed in relation with G2A receptor[30].
Overexpression of G2A, GPR4, and TDAG8 in human cancers has been found to play a role in driving or maintaining tumor formation, however, transformation was achieved without addition of lysolipids[31,32]. Bektas et al reported ligand-independent signaling of GPR4 and its inability to respond to SPC and LPC in several assay systems, that is, GTPγS binding, receptor internalization, and arrestin translocation[33]. Additionally, the original paper that reported G2A-LPC pairing was recently retracted by authors, because they could not confirm the LPC-binding experiments[34]. Constitutive activation of GPCR and lipid-independent responses raises a possibility, that is, another activator of GPCR is present in the culture medium or secreted from GPCR-transfected cells. Such a possibility has been suggested and supported with proton by five independent groups[21,35–38].
OGR1 subfamily as proton-sensing GPCRs
Ludwig et al (2003) reported OGR1 and GPR4 to be proton-sensing receptors. At pH 7.8, OGR1 was inactive, but activated fully inositol phosphate (IP) formation at pH 6.8. Ludwig et al predicted several hydrogen-bond interactions occurring between unprotonated histidines by using a computational 3D model of OGR1. Under alkaline conditions these interactions could stabilize the receptor in an inactive state. Exposure to an acidic pH would destabilize the hydrogen bonds, switching the receptor to its active conformation. Indeed, mutation of several histidines (H17, H20, H84, H169, and H269) to phenylalanines reduced proton-sensing ability of OGR1 (Figure 2)[35]. In the same paper, authors observed that a very similar activation of GPR4 by pH change, but GPR4 activates the Gs-adenylyl cyclase-cAMP pathway[35]. However, they were not able to observe any effect of SPC and LPC, previously reported ligands, on OGR1 and GPR4. In 2004, Murakami et al reported that G2A functions as a proton-sensing GPCR[21]. Transient transfection of G2A caused significant activation of the zif 268 promoter and IP accumulation at pH 7.6 and lowering extracellular pH augmented the activation only in G2A-expressing PC12h cells[21]. Site-directed mutation of His-174, which is predicted to be located at the extracellular part of the transmembrane helix IV (Figure 2), reduced partially G2A-dependent signaling at lower pH. They found that LPC and SPC did not cause IP formation at pH 7.6, but LPC inhibited IP formation at pH 6.8 in a dose-dependent manner, suggesting that LPC acts as an antagonist not an agonist. Wang et al reported that TDAG8 is also a proton-sensing GPCR stimulating cAMP accumulation[36]. They found that psychosine and SPC are antagonistic on pH-dependent responses in the cells transfected with TDAG8. Psychosine-sensitive and pH-dependent cAMP accumulation was also observed in mouse thymocytes, where TDAG8 is endogenously expressed[36]. Radu et al conducted experiments with all 4 members of OGR1 subfamily to test proton-sensibility and confirmed previous reports on OGR1, GPR4, and TDAG8[38]. However, G2A was insensitive or less sensitive to extracellular pH change in their experimental conditions[38]. They suggested that lack of many histidine residues, defined to be involved in pH-sensing of OGR1, could be a cause for insensitiveness of G2A to acidic pH (Figure 2)[38]. Also they suggested that the constitutive activation of G2A in neutral pH might be resulted from maintaining active conformation of G2A via positively charged amino acids in human and mouse G2A instead of conserved histidines (Figure 2)[38]. Ishii et al reported that TDAG8 is a proton-sensing GPCR, however, they were not able to observe any inhibitory effect of psychosine on pH-dependent TDAG8 activation[37]. Therefore, a series of publications propose that extracellular proton could be an activator of the OGR1 subfamily of GPCRs. More than two independent groups reported proton-sensing properties of OGR1, GPR4, and TDAG8 (Table 1). In the case of G2A, constitutive activation at pH 7.4 has been observed in many transfected cells by many research groups, however, pH-dependent activation was supported only by one group[21] and was not fully reproduced by another group[38]. Dependence of pH sensing on the histidine residues on the extracellular domains of GPCRs has been tested on OGR1, TDAG8, and G2A[21,35–37]. However, site-directed mutagenesis study of the histidines on GPR4 has not been experimentally reported.
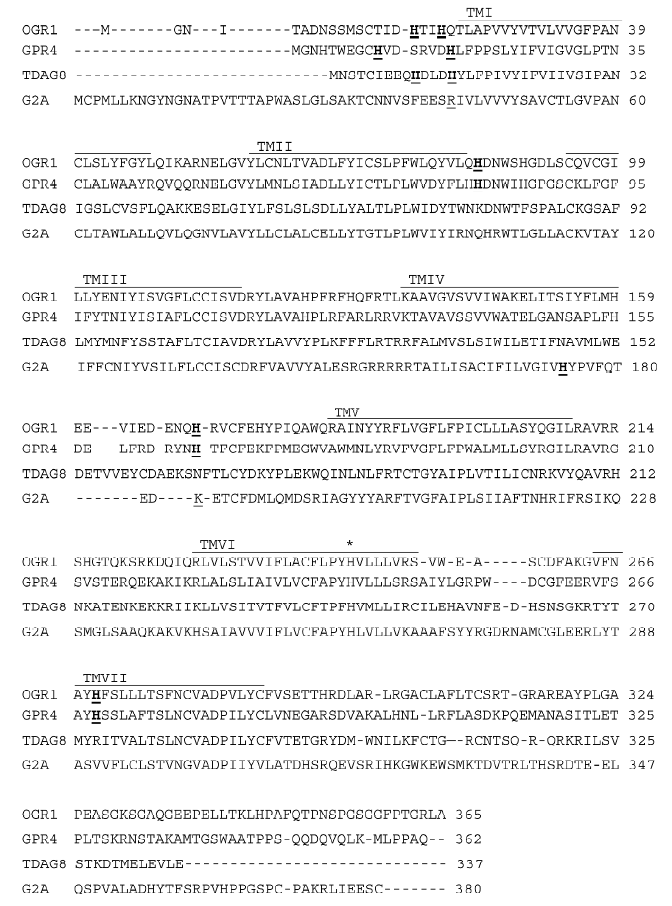
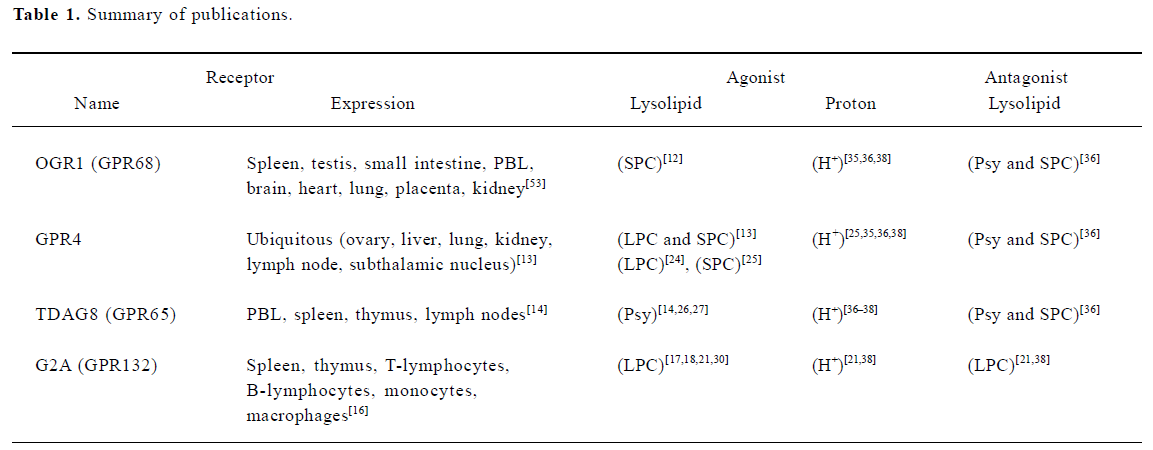
Full table
In summary, there are four opinions in the published reports, that is agonism of lysolipid, antagonism of lysolipid, agonism of proton, and no confirmation of lysolipid action on OGR1 subfamily GPCRs. Table 1 shows list of publications supporting each opinion except negative observation or constitutive activation.
Two ligands for a GPCR, proton vs lysolipid
As for lysolipids as ligands, Ludwig et al could not confirm such activation of GPCRs with lysolipids[35], and three research groups observed antagonistic effects of LPC, SPC and psychosine on the GPCRs in acidic conditions rather than agonism (Table 1)[21,36–38]. In summary, lysolipids have been suggested as ligands for the OGR1 subfamily GPCRs, however, all four members of the GPCRs have also been proposed as proton-sensing GPCRs. OGR1 subfamily has been considered as a contentious GPCR subgroup, because pairing it with lysolipids has been controversial in the scientific society[4,21,33,35,36]. Additionally, the original G2A paper was retracted[34] and GPR4 paper would be retracted (Y XU, FASEB conference, 2005). However, there are growing numbers of reports supporting actions of lysolipids on OGR1 subfamily GPCRs (Table 1). As proposed by Kim et al it can be dependent on cell types. Kim et al recently confirmed pH-dependent cAMP production in GPR4-transfected HEK293 cells but not in GPR4-transfected HUVEC or HMEC-1 cells[25]. Cell-type specific functions remind us RAMPS (receptor activity-modulating proteins) which are essential proteins for expression and function of GPCRs such as CGRP (calcitonin gene-related peptide) and adrenomedullin[39]. If there are RAMP-like proteins specific for OGR1 subfamily, the complicated results may be solved. Wang et al recently observed spontaneous internalization of murine G2A and reported that LPC induces surface redistribution and stabilization of murine G2A[19]. Such an action of LPC may support spontaneous activation of G2A and explain agonistic and antagonistic effects of LPC. If spontaneous activity of G2A was presumed as control level in neutral pH, LPC-induced action might be interpreted as agonism. However, if spontaneous activation or proton-activated effect was thought as agonism, LPC-induced action might be considered as antagonism. Further investigation on cell-type specificity and receptor distribution in the cells may clarify action mode of OGR1 subfamily GPCRs by both chemicals, proton and lysolipids.
Therefore, it is not easy to say which chemical is the real ligand, although both chemicals could be called as modulators of the GPCRs. Finding of antagonistic effects of lysolipids on the receptors at acidic conditions may advance our understanding and might reconcile the controversy in the future. If both chemicals could activate the same GPCRs in certain conditions, another issue might be two ligands for a GPCR. Dual actions may be a rare example in the GPCR area. However, considering that TRPV1 could be activated by capsaicin, proton, heat and lipids, two chemicals for a GPCR could not be a surprising action mode in biological sciences[10]. Investigation of physiological roles and pathological implications of the GPCRs in the future may lighten importance of discovery of proton-sensing GPCRs, because acidosis is related with many diseases such as cancer[40], asthma[41], atherosclerosis[42], arthritis[43], and osteopenia[35,44].
Proton as an agonist
Another issue remains; could proton be a ligand? To be an agonist, it should bind to GPCR specifically and reversibly[45]. Furthermore, it should dose-dependently activate GPCR. In five publications, proton has been shown to fulfill all the above criteria, except specificity[21,35–38] . Basal activity of many other GPCRs has been observed in neutral pH without apparent presence of endogenous ligands as like δ-opioid receptor[46,47]. Such basal activity of GPCR has further been confirmed by inverse agonists, which reduce the basal activity in a dose-dependent manner[46,47]. Many GPCRs have histidine residues in extracellular loops and outer segments of GPCR helixes. Thus, if change of extracellular pH could change basal activities of many GPCRs, although the magnitude of change varies, this means lack of specificity. If many GPCRs are activated or inactivated by change of pH without presence of any agonists, proton can not be an agonist, because it lacks specificity, even though OGR1 subfamily activation with proton was the greatest response among GPCRs activated with proton. Indeed, modulation of GPCRs by extracellular pH has been reported in P2Y4 ATP receptor and calcium-sensing receptor[48,49].
Four research groups have used the term “proton-sensing GPCR” for OGR1 subfamily, however, the term for proton, the counterpart, is omitted or the term “ligand” has been used for proton. However, it is inadequate, because of the above-mentioned reason, lack of specificity. GPCR modulator may be the good term for proton, because it changes activity of GPCRs, but it can not be an agonist. So far, the smallest particle ever reported to be a GPCR activator is photon. In this case, photon energy in light activates rhodopsin GPCR by isomerisation of 11-cis-retinal to trans conformation within the rhodopsin helixes[50,51]. Now, proton might become the second small molecule activating GPCRs. Although lysolipids as the ligand of OGR1 subfamily still remain controversial, proton as a ligand for the GPCRs also need to be considered with caution, because an endogenous ligand might be waiting to be discovered, suggesting possible presence of another chemical to activate OGR1 subfamily in neutral conditions (Figure 3). It may not be a surprise if there is another chemical activating OGR1 subfamily in neutral pH such as prostaglandins and capsaicin modulating TRPV1[52].
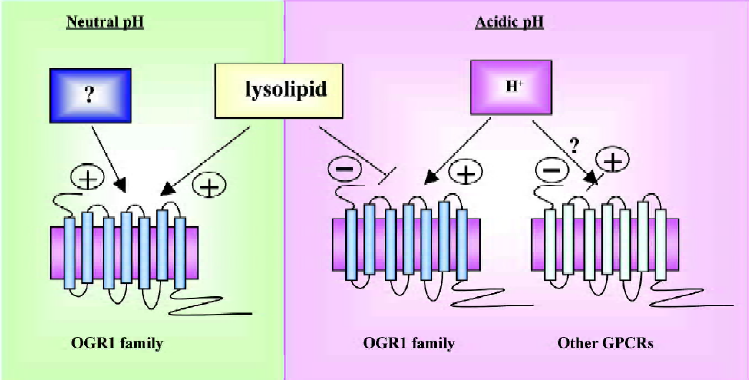
In summary, proton activates OGR1 subfamily GPCRs and lysolipids modulate activity of OGR1 GPCRs positively or negatively. However, we need to consider other two possibilities. One is that modulation by proton could be generalized in other GPCRs even though the magnitude varies (Figure 3). Second, there could be another chemical activating OGR1 subfamily in neutral pH (Figure 3).
Acknowledgement
The author thanks Prof Fumikazu OKAJIMA (Gunma University) for his critical comments.
References
- Im DS. Orphan G protein-coupled receptors and beyond. Jpn J Pharmacol 2002;90:101-6.
- Perez DM. From plants to man: the GPCR “tree of life”. Mol Pharmacol 2005;67:1383-4.
- Vassilatis DK, Hohmann JG, Zeng H, Li F, Ranchalis JE, Mortrud MT, et al. The G protein-coupled receptor repertoires of human and mouse. Proc Natl Acad Sci USA 2003;100:4903-8.
- Kostenis E. A glance at G-protein-coupled receptors for lipid mediators: a growing receptor family with remarkably diverse ligands. Pharmacol Ther 2004;102:243-57.
- Lynch KR, O’Neill GP, Liu Q, Im DS, Sawyer N, Metters KM, et al. Characterization of the human cysteinyl leukotriene CysLT1 receptor. Nature 1999;399:789-93.
- Yokomizo T, Izumi T, Chang K, Takuwa Y, Shimizu T. A G-protein-coupled receptor for leukotriene B4 that mediates chemotaxis. Nature 1997;387:620-4.
- Sakurai T, Amemiya A, Ishii M, Matsuzaki I, Chemelli RM, Tanaka H, et al. Orexins and orexin receptors: a family of hypothalamic neuropeptides and G protein-coupled receptors that regulate feeding behavior. Cell 1998;92:573-85.
- Hecht JH, Weiner JA, Post SR, Chun J. Ventricular zone gene-1 (vzg-1) encodes a lysophosphatidic acid receptor expressed in neurogenic regions of the developing cerebral cortex. J Cell Biol 1996;135:1071-83.
- Lee MJ, Van Brocklyn JR, Thangada S, Liu CH, Hand AR, Menzeleev R, et al. Sphingosine-1-phosphate as a ligand for the G protein-coupled receptor EDG-1. Science 1998;279:1552-5.
- Ahern GP, Brooks IM, Miyares RL, Wang XB. Extracellular cations sensitize and gate capsaicin receptor TRPV1 modulating pain signaling. J Neurosci 2005;25:5109-16.
- Voilley N. Acid-sensing ion channels (ASICs): new targets for the analgesic effects of non-steroid anti-inflammatory drugs (NSAIDs). Curr Drug Targets Inflamm Allergy 2004;3:71-9.
- Xu Y, Zhu K, Hong G, Wu W, Baudhuin LM, Xiao Y, et al. Sphingosylphosphorylcholine is a ligand for ovarian cancer G-protein-coupled receptor 1. Nat Cell Biol 2000;2:261-7.
- Zhu K, Baudhuin LM, Hong G, Williams FS, Cristina KL, Kabarowski JH, et al. Sphingosylphosphorylcholine and lysophosphatidylcholine are ligands for the G protein-coupled receptor GPR4. J Biol Chem 2001;276:41325-35.
- Im DS, Heise CE, Nguyen T, O’Dowd BF, Lynch KR. Identification of a molecular target of psychosine and its role in globoid cell formation. J Cell Biol 2001;153:429-34.
- Xu Y, Fang XJ, Casey G, Mills GB. Lysophospholipids activate ovarian and breast cancer cells. Biochem J 1995;309:933-40.
- Weng Z, Fluckiger AC, Nisitani S, Wahl MI, Le LQ, Hunter CA, et al. A DNA damage and stress inducible G protein-coupled receptor blocks cells in G2/M. Proc Natl Acad Sci USA 1998;95:12334-9.
- Radu CG, Yang LV, Riedinger M, Au M, Witte ON. T cell chemotaxis to lysophosphatidylcholine through the G2A receptor. Proc Natl Acad Sci USA 2004;101:245-50.
- Yang LV, Radu CG, Wang L, Riedinger M, Witte ON. Gi-independent macrophage chemotaxis to lysophosphatidylcholine via the immunoregulatory GPCR G2A. Blood 2005;105:1127-34.
- Wang L, Radu CG, Yang LV, Bentolila LA, Riedinger M, Witte ON. Lysophosphatidylcholine-induced Surface Redistribution Regulates Signaling of the Murine G Protein-coupled Receptor G2A. Mol Biol Cell 2005;16:2234-47.
- Han KH, Hong KH, Ko J, Rhee KS, Hong MK, Kim JJ, et al. Lysophosphatidylcholine up-regulates CXCR4 chemokine receptor expression in human CD4 T cells. J Leukoc Biol 2004;76:195-202.
- Murakami N, Yokomizo T, Okuno T, Shimizu T. G2A is a proton-sensing G-protein coupled receptor antagonized by lysophosphatidylcholine. J Biol Chem 2004;279:42484-91.
- Lin P, Ye RD. The lysophospholipid receptor G2A activates a specific combination of G proteins and promotes apoptosis. J Biol Chem 2003;278:14379-86.
- Ikeno Y, Konno N, Cheon SH, Bolchi A, Ottonello S, Kitamoto K, et al. Secretory phospholipase A2 induces neurite outgrowth in PC12 cells through lysophosphatidylcholine generation and activation of G2A receptor. J Biol Chem 2005;280:28044-52.
- Lum H, Qiao J, Walter RJ, Huang F, Subbaiah PV, Kim KS, et al. Inflammatory stress increases receptor for lysophosphatidylcholine in human microvascular endothelial cells. Am J Physiol Heart Circ Physiol 2003;285:H1786-9.
- Kim KS, Ren J, Jiang Y, Ebrahem Q, Tipps R, Cristina K, et al. GPR4 plays a critical role in endothelial cell function and mediates the effects of sphingosylphosphorylcholine. FASEB J 2005;19:819-21.
- Maghazachi AA, Knudsen E, Jin Y, Jenstad M, Chaudhry FA. D-galactosyl-beta1-1'-sphingosine and D-glucosyl-beta1-1'-sphingosine induce human natural killer cell apoptosis. Biochem Biophys Res Commun 2004;320:810-5.
- Malone MH, Wang Z, Distelhorst CW. The glucocorticoid-induced gene tdag8 encodes a pro-apoptotic G protein-coupled receptor whose activation promotes glucocorticoid-induced apoptosis. J Biol Chem 2004;279:52850-9.
- Tosa N, Murakami M, Jia WY, Yokoyama M, Masunaga T, Iwabuchi C, et al. Critical function of T cell death-associated gene 8 in glucocorticoid-induced thymocyte apoptosis. Int Immunol 2003;15:741-9.
- Le LQ, Kabarowski JH, Weng Z, Satterthwaite AB, Harvill ET, Jensen ER, et al. Mice lacking the orphan G protein-coupled receptor G2A develop a late-onset autoimmune syndrome. Immunity 2001;14:561-71.
- Yan JJ, Jung JS, Lee JE, Lee J, Huh SO, Kim HS, et al. Therapeutic effects of lysophosphatidylcholine in experimental sepsis. Nat Med 2004;10:161-7.
- Zohn IE, Klinger M, Karp X, Kirk H, Symons M, Chrzanowska-Wodnicka M, et al. G2A is an oncogenic G protein-coupled receptor. Oncogene 2000;19:3866-77.
- Sin WC, Zhang Y, Zhong W, Adhikarakunnathu S, Powers S, Hoey T, et al. G protein-coupled receptors GPR4 and TDAG8 are oncogenic and overexpressed in human cancers. Oncogene 2004;23:6299-303.
- Bektas M, Barak LS, Jolly PS, Liu H, Lynch KR, Lacana E, et al. The G protein-coupled receptor GPR4 suppresses ERK activation in a ligand-independent manner. Biochemistry 2003;42:12181-91.
- Kabarowski JH, Zhu K, Le LQ, Witte ON, Xu Y. Lysophos-phatidylcholine as a ligand for the immunoregulatory receptor G2A. Science 2001;293:702-5. Retraction in: Witte ON, Kabarowski JH, Xu Y, Le LQ, Zhu K. Retraction. Science 2005; 307: 206.
- Ludwig MG, Vanek M, Guerini D, Gasser JA, Jones CE, Junker U, et al. Proton-sensing G-protein-coupled receptors. Nature 2003;425:93-8.
- Wang JQ, Kon J, Mogi C, Tobo M, Damirin A, Sato K, et al. TDAG8 is a proton-sensing and psychosine-sensitive G-protein-coupled receptor. J Biol Chem 2004;279:45626-33.
- Ishii S, Kihara Y, Shimizu T. Identification of T cell death-associated gene 8 (TDAG8) as a novel acid sensing G-protein-coupled receptor. J Biol Chem 2005;280:9083-7.
- Radu CG, Nijagal A, McLaughlin J, Wang L, Witte ON. Differential proton sensitivity of related G protein-coupled receptors T cell death-associated gene 8 and G2A expressed in immune cells. Proc Natl Acad Sci U S A 2005;102:1632-7.
- Conner AC, Simms J, Hay DL, Mahmoud K, Howitt SG, Wheatley M, et al. Heterodimers and family-B GPCRs: RAMPs, CGRP and adrenomedullin. Biochem Soc Trans 2004;32:843-6.
- Wike-Hooley JL, Haveman J, Reinhold HS. The relevance of tumour pH to the treatment of malignant disease. Radiother Oncol 1984;2:343-66.
- Hunt JF, Fang K, Malik R, Snyder A, Malhotra N, Platts-Mills TA, et al. Endogenous airway acidification. Implications for asthma pathophysiology. Am J Respir Crit Care Med 2000;161:694-9.
- Naghavi M, John R, Naguib S, Siadaty MS, Grasu R, Kurian KC, et al. pH Heterogeneity of human and rabbit atherosclerotic plaques; a new insight into detection of vulnerable plaque. Atherosclerosis 2002;164:27-35.
- Christensen BN, Kochukov MY, McNearney TA, Taglialatela G, Westlund KN. A. Proton-Sensing G-Protein Coupled Receptor Mobilizes Calcium in Human Synovial Cells. Am J Physiol Cell Physiol 2005;289:C601-8.
- Krieger NS, Frick KK, Bushinsky DA. Mechanism of acid-induced bone resorption. Curr Opin Nephrol Hypertens 2004;13:423-36.
- Kenakin TP. Drug-receptor theory. In: Kenakin TP. Pharmacological analysis of drug-receptor interaction. New York: Raven Press; 1987. p 1–30.
- Strange PG. Mechanisms of inverse agonism at G-protein-coupled receptors. Trends Pharmacol Sci 2002;23:89-95.
- Tryoen-Toth P, Decaillot FM, Filliol D, Befort K, Lazarus LH, Schiller PW, et al. Inverse agonism and neutral antagonism at wild-type and constitutively active mutant delta opioid receptors. J Pharmacol Exp Ther 2005;313:410-21.
- Wildman SS, Unwin RJ, King BF. Extended pharmacological profiles of rat P2Y2 and rat P2Y4 receptors and their sensitivity to extracellular H+ and Zn2+ ions. Br J Pharmacol 2003;140:1177-86.
- Quinn SJ, Bai M, Brown EM. pH Sensing by the calcium-sensing receptor. J Biol Chem 2004;279:37241-9.
- Lagnado L, Baylor D. Signal flow in visual transduction. Neuron 1992;8:995-1002.
- Palczewski K, Kumasaka T, Hori T, Behnke CA, Motoshima H, Fox BA, et al. Crystal structure of rhodopsin: A G protein-coupled receptor. Science 2000;289:739-45.
- Nagy I, Santha P, Jancso G, Urban L. The role of the vanilloid (capsaicin) receptor (TRPV1) in physiology and pathology. Eur J Pharmacol. 2004;500:351-69.
- Xu Y, Casey G. Identification of human OGR1, a novel G protein-coupled receptor that maps to chromosome 14. Genomics 1996;35:397-402.