In vitro characteristics of poly(lactic-co-glycolic acid) microspheres incorporating gelatin particles loading basic fibroblast growth factor
Introduction
Basic fibroblast growth factor (bFGF) is a heparin binding growth factor that stimulates the proliferation of a wide variety of cells, including mesenchymal, neuroectodermal and endothelial cells. bFGF also exerts a potent angiogenic activity in vivo. But bFGF is easily hydrolyzed by an enzyme, whose half-life is 3 to 10 min in vivo[1], so its availability is very low. Physicochemical and biological properties of bFGF are different from those of conventional drugs. These properties include molecular size, biological half-life, conformational stability, solubility, and dose requirement. The delivery systems that function well for conventional drugs may not be suitable for FGF. Therefore, designing a unique bFGF delivery system is required[2]. Researchers turn attention to biodegradable polymeric systems. The biodegradable polymeric systems may be categorized into hydrophilic polymeric systems, such as gelatin nanoparticles and microspheres[3], and hydrophobic polymeric systems such as poly(lactic-co-glycolic acid) (PLGA) microspheres[4-6,14,25]. Neither the hydrophilic nor the hydrophobic system is ideal for bFGF delivery. Both have advantages and disadvantages. For example, the hydrophilic polymeric systems are biocompatible with the peptide/protein drugs, but have difficulty in achieving sustained drug release[7]. When the systems absorb water and swell, bFGF molecules will release fast. In contrast, the hydrophobic polymeric systems have the capability of sustained release. However, they are incompatible with the water-soluble bFGF. The hydrophobicity of the polymers may induce unfolding of bFGF molecules; therefore, the bFGF may lose their biological activity after being loaded in and then released from the hydrophobic polymeric systems[8,9]. To promote the advantages and overcome the disadvantages of both the hydrophilic and the hydrophobic polymeric systems, published studies have reported that poly(lactic-co-glycolic acid) (PLGA) and some hydrophilic materials were combined in a composite system[2,8,22,23], this system worked well for some drugs in vitro, such as insulin[8], bovine serum albumin[2], isopropyl myristate[25], vapreotide[26], and so on. To create a new biodegradable system for bFGF, a gelatin particle-PLGA micro-sphere composite microsphere was prepared[17–20]. Micro-encapsulated bFGF can be applied to repair an injured peripheral nerve[24], injured spinal cord, or other damaged tissues which need bFGF to be released over a long time.
Materials and methods
Materials Gelatin (bloom strength, 225; pI, 4.7,) was purchased from Sigma Chemical (St Louis, MO, USA), bFGF and PLGA (molar ratio of D, L-lactic to glycolic acid, 75:25) were purchased from Sigma Chemical. CH2Cl2 (AR) and silicon oil (350 cs) were obtained from Kelong Chemical (Chengdu, China).
Preparation of gelatin particles containing bFGF Preparation of gelatin particles was carried out according to the published literature[3]. Aqueous gelatin solution 20 mL (20%) (added 4 mL bFGF 2.0 µg/mL) was added to 350 mL of corn oil (preheated to 37 °C) in a water bath, and then homogenized at a speed of 10 000 rpm for 10 min to form a water-in-oil emulsion by a homogenizer (model BME100Lx, Weiyu, Shanghai, China). The emulsion was then cooled to -20 °C in a refrigerator, while the emulsion was changed to a suspension. The suspension was then separated at a speed of 2000 rpm in a centrifuge at 4 °C for 10 min. The precipitum (gelatin hydrogel particles) was washed four times using acetone to remove the residual corn oil and water. The collected gelatin microspheres were lyophilized overnight, then a white, flowing powder was obtained.
Preparation of gelatin particle-PLGA microsphere composite The PLGA-gelatin composite microsphere was prepared by using a modified phase separation method[8]. PLGA (0.22 g) was dissolved in 5 g CH2Cl2, and then added 0.6 g gelatin microspheres containing bFGF while being stirred at 1000 rpm. The mixture was poured progressively into dimethicone (10 mL) stirred at 800 rpm. Once the droplets of the PLGA solution containing gelatin particles reached the desired size, the emulsion was transferred to a beaker containing 200 mL n-heptane. Finally, the microspheres were collected by filtration, washed with heptane and lyophilized for 24 h (Figure 1).
Preparation of PLGA microsphere containing bFGF PLGA microsphere containing bFGF without gelatin particles was also prepared for the purpose of comparison. bFGF (10.0 µg) was dissolved in 2 mL phosphate-buffered saline (PBS; pH 7.6). This aqueous solution was emulsified in the PLGA solution using a homogenizer to form a water-in-oil emulsion. The emulsion was then cooled to -100 °C in a refrigerator and the solid emulsion was lyophilized over night. The PLGA microsphere containing bFGF was then obtained.
Morphology of microparticles A light microscope (CKX41,Olympus,Tokyo Japan) was used to examine the microscopic characteristics of gelatin particles and the gelatin particle-PLGA microsphere composites.
Size and size distribution of microparticles A laser diffraction particle size distribution analyzer (LA-300, Horiba, Osaka Japan) was used to determine the size and size distribution of the microparticles.
Determination of bFGF loading efficiency bFGF loaded in the gelatin particle-PLGA microsphere composite was determined directly by recovering bFGF from the composite[9–13]. The experiments were conducted in triplicate. Dried composite (20 mg) was transferred to a separate funnel and methylene chloride (10 mL) was added into the composite sample. After the composite was dissolved, phosphate buffer (5 mL) was added to the separate funnel, and the mixture composite was dissolved. Phosphate buffer (5 mL) was added to the separate funnel, and the mixture was agitated in a shaker for 12 h. After the aqueous phase and the organic phase had separated completely, the aqueous layer was collected for the determination of bFGF. A capillary electrophoresis apparatus (P/ACE5000, Beckman California USA) was employed to determine bFGF concentration in the aqueous extractant using Tris-borax buffer (pH8.4) as a mobile phase. A UV detector (200 nm) was used to detect the bFGF. The bFGF loading efficiency was calculated by the formula:
Where Mactual is the actual amount of bFGF in each composite determined by the above experiment and Mtheoretical is the theoretical amount of bFGF in each composite calculated from the quantity added in the fabrication process.
bFGF release in vitro In vitro bFGF release studies were performed in triplicate. The gelatin particle-PLGA (poly lactic-co-glycolic acid) microsphere composite (100 mg) was dispersed in 10 mL PBS. The dispersion was incubated at 37 °C in a shaking water bath at a shaking speed of 40 rpm. At designed time point, 1 mL of the supernatant was taken and 1 mL of fresh PBS was added to it. It was then analyzed using the same capillary electrophoresis apparatus described for the determination of the released bFGF and its integrity. The cumulative amount of the bFGF released from the composite is defined by the following equation:
Where Mt is the amount of bFGF released at time t, and Mactual is the actual amount of bFGF loaded in the composite defined above.
Results
Microscopic characteristics of microparticles Figure 2 shows typical gelatin particles with spherical shape and uniform size. Figure 3 shows the PLGA-gelatin composite microspheres with spherical shape and uniform size, which are bigger than gelatin particles.
Size and size distribution of microparticles Size and size distribution are important properties of the composite microspheres of microparticulate drug delivery systems. The reason is that the size of the microparticles has a great effect on drug release characteristics[14]. From Figure 4, we can see that the composites possess a normal or Gaussian size distri-bution. The average diameter of the gelatin particles and gelatin particle-PLGA microsphere composites are approximately 8 µm and 10 µm, respectively. The stir rate of the emulsion apparatus in the process of preparing the gelatin particles is a critical factor in determining the size of the composites[15]. In our experiments, with a stir rate of approximately 3 000 rpm, the average diameters of the collected gelatin particles and the composites could reach a size of 20 μm and 25 µm, respectively. However, when the stir rate increased to 10 000 rpm, the average diameters of the collected gelatin particles and the composites were approximately 8 μm and 10 μm, respectively. In addition, concentration of gelatin is also an important factor. The diameter of the composites was also affected by the concentration of PLGA organic solution. Increased concentration of PLGA would lead to increase in diameter of the composites.
Peptide loading efficiency There are several methods in preparing the gelatin particle-PLGA microspheres in previously published studies[2,10,21]. Load efficiency is an important factor for drug manufacture. To take the loading efficiency into consideration, the phase separation method is recommended for the preparation of PLGA microspheres and encapsulation of water-soluble substances. Every step of the phase separation method is conducted in an organic system. There is no aqueous phase involved in this method. The mean bFGF loading efficiency of the gelatin particle-PLGA microsphere composite was approximately 80.50%. Loading efficiency of PLGA microsphere containing bFGF was up to 96.4% higher than the gelatin particle-PLGA microsphere.
bFGF release The bFGF release curve of the composite presented two phases: fast release phase (30%) and sequential slow release phase. The fast release phase might be caused by some gelatin particles sitting on or imbedding in the PLGA microsphere surface, or imperfection of the composite surface. The mechanism of slow release phase is relatively complicated. It may involve the following aspects[2]: (1) PLGA degradation; (2) diffusion of bFGF molecules through a tortuous water-filled path in the PLGA microsphere matrix; (3) diffusion of bFGF molecules through the swollen gelatin nanoparticles; (4) water permeation through the PLGA matrix and absorption by the gelatin nanoparticles; (5) gelatin molecules cross-linking internally. In comparison with the gelatin particle-PLGA microsphere composite, the PLGA microspheres containing bFGF only released bFGF very fast. In approximately 10 d, about 80% of bFGF has been released. This burst release retards the application of PLGA micro-spheres to peptide drug delivery[16]. However the new gelatin particle-PLGA microsphere composite released the peptide slowly, only 50% of the loaded peptide molecules were released In 18 d. After 42 d, bFGF could still be released from the composite, and only approximately 65% has been released. But the released rate became lower than before (Figure 5). Retardation of the burst release or the slow release characteristic of the composite makes this new biodegradable system a good carrier candidate for prolonged peptide/protein drug delivery.
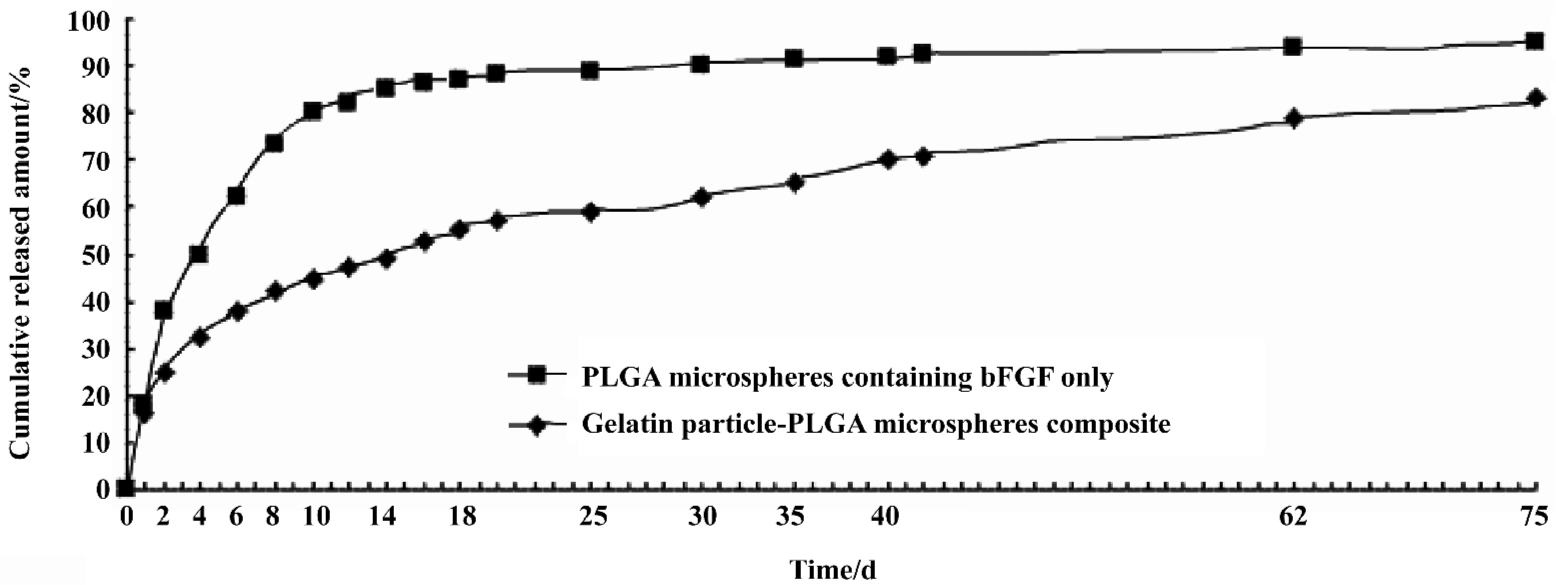
Discussion
Peptide drugs are easily denatured or easily lose their biological activity. The denaturation of peptide drugs may include the following aspects[3]: aggregation, degradation, and/or unfolding. All of these denaturation aspects can differentiate the peptide molecules from their native counterpart conformationally or structurally. The conformational or structural change may be detected instrumentally. Capillary electrophoresis has many potential uses, such as analyzing purity and structure conformation of a substance. The separation mechanism of capillary electrophoresis is based on the difference in the charge-to-mass ratio of individual analytes. The difference in the charge-to-mass ratio results in the different electrophoretic mobility of individual analytes. The migration rate of an analyte is influenced not only by its electric charge but also by the bulk flow of the mobile phase. This flow is known as electro-osmotic flow, which is a result of the movement of a counter-anion at the wall toward the cathode. For the fused silica capillary, the electro-osmotic flow is strong and has great influence on the migration rate of the analyte.
The capillary electrophoresis system used in this experiment has the anode on the injection side. In the mobile phase of pH 8.4, the bFGF is negatively charged. Under the applied high voltage field, the negatively charged bFGF is pulled toward the anode. Hence, the negatively charged species with higher charge-to-mass ratio will present a longer retention time, while those with lower charge-to-mass ratio will display a shorter retention time. During preparation and storage of the gelatin particle-PLGA microsphere composite, harmful conditions that bFGF molecules encountered may cause peptide denaturation, such as aggregation, degrada-tion, and/or unfolding. These harmful conditions may include contact with organic solvents, exposure to the hydrophobic and acidic microenvironment of the PLGA matrix, and mechanical agitation. Protein denaturation changes the mass-to-surface charge ratio, which results in distinctly different capillary electrophoretic peaks from their parent product. We can see that the bFGF released from PLGA microspheres containing bFGF only presents deformative peaks at the retention time of 8.32 and 9.51 min (Figure 6). These peaks are an indication of formation of a new mass-to-surface charge ratio or formation of a new molecular weight species, which probably means that the bFGF has been denatured to some extent. The peak at 8.32 min indicates an aggregated species because they possess a lower charge-to-mass ratio, which results in greater electrophoretic mobility, whereas the peak at 9.51 min may be a result of a degraded species, because they have a higher charge to mass ratio, which results in lower electrophoretic mobility. In contrast, bFGF released from the gelatin particle-PLGA microsphere composite displays a normal bFGF peak, which is the same as that of the native bFGF. The percentage of integrity change is calculated from the peak area of the shoulder peaks divided by the area of the total peaks. We can see that more bFGF molecules lose their integrity as the release time increases (Table 1). In comparison, the bFGF molecules protected by the gelatin particles do not show any deformative peaks, even after 25 d of release. Therefore, it may be concluded that the gelatin particles in the PLGA microspheres showed some potential to protect bFGF from integrity loss or denaturation during the microsphere preparation, storage and release.
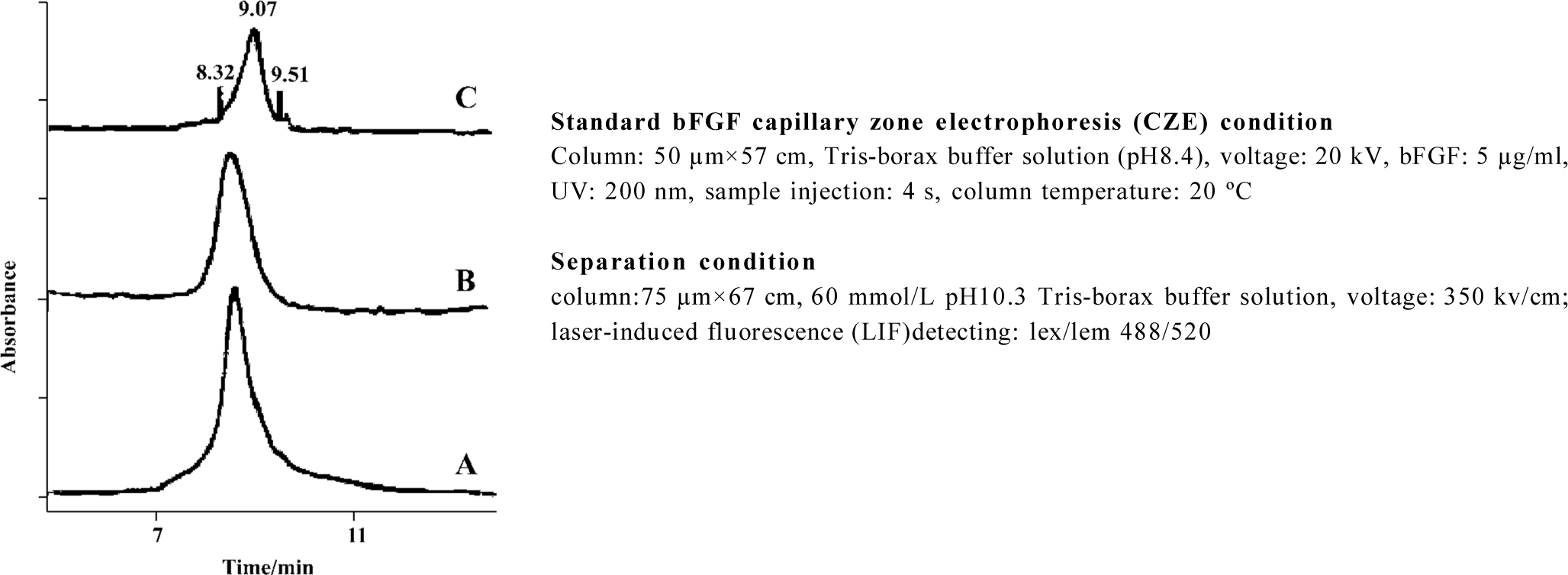
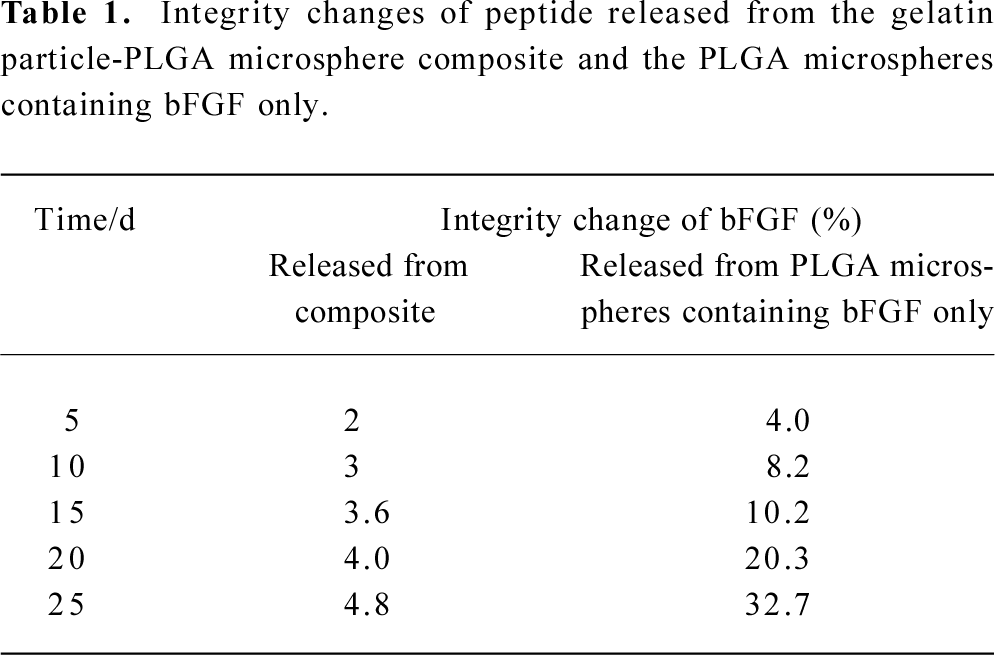
Full table
In conclusion, we take a modified approach to develop a biodegradable system for the prolonged or controlled release of peptide/protein drugs, especially for bFGF. This new system is a combination of a hydrophilic polymer (gelatin) and a hydrophobic polymer (PLGA). The gelatin is formulated as particles, while the PLGA is formulated as micro-spheres. The particles are then encapsulated in the micro-spheres. The experimental results show that the bFGF-loaded gelatin particles were successfully encapsulated in the PLGA microspheres. The encapsulation was conducted by a phase separation method. This encapsulation practice creates a new composite system. The average diameter of the gelatin particle-PLGA microsphere composites is between 5 and 18 μm. The bFGF loading efficiency is approximately 80.50% for the composite. Release experiments show that only 70% of bFGF is released from the gelatin particle-PLGA micro-sphere composite in 60 d, which indicates that this new system possesses sustained release characteristics for peptide drugs. This new system also demonstrates the capability of preventing peptide drugs from integrity loss and denaturation.
References
- Duan H, Shen B, He Q, Pei FX, Chen J. Preparation of bFGF-PLGA sustained release microspheres and studies on their release characteristics and biologic activity in vitro. Chin Pharm J 2004;139:196-8. Chinese..
- Li JK, Wang N, Wu XS. A novel biodegradable system based on gelatin nanoparticles and poly (lactic-co-glycolic acid) micro-spheres for protein and peptide drug delivery. J Pharm Sci 1997;86:891-5.
- Tabata Y, Ikada Y. Synthesis of gelatin microspheres containing interferon. Pharm Res 1989;6:422-7.
- Ruiz JM, Benoit JP. In vivo peptide release from poly (DL-lactic acid-co-glycolic acid) copolymer 50/50 microspheres. J Controll Release 1991;16:177-86.
- Heya T, Okada H, Ogawa Y, Toguchi H. Factors influencing the profiles of TRH release from copoly (d,l-lactic/glycolic acid) microspheres. Int J Pharm 1991;72:199-205.
- Cohen S, Yoshioka T, Lucarelli M, Hwang LH, Langer R. Controlled delivery systems for proteins based on poly (lactic/glycolic acid) microspheres. Pharm Res 1991;8:713-20.
- Li JK, Wang N, Wu XS. Gelatin nano-encapsulation of protein/peptide drugs using an e-mulsifier-free emulsion method. J Microencapsul 2006. in press.
- Wang N, Wu XS. A novel approach to stabilization of protein drugs in poly(lactic-co-glycolic acid) microspheres using agarose hydrogel. Int J Pharm 1998;166:1-14.
- Zhou GH. Luo GAn, Zhou KY, Gu ZL. On-line capillary electrophores is-mass spectrometry for determina ion of peptide mixture and protein mixture. Chem J Chin Univ 1998;l:3353-8. Chinese..
- Liang H, Wang ZG, Gu JL, Fu RN. A peptides migration model in capillary zone electrophoresis. J Beijing Inst Technol 1996;5:35-9. Chinese..
- Guo WY, Bai XZ, Zhou L, Zhao Y, Yang J, Yu DM. Capillary zone electrophoresis & protein and peptide’s determination. Jinzhou Med Coll Trans 1995;16:56-8. Chinese..
- Cui B, Cheng YH, Zhong QP. Application of capillary electrophoresis in separation and detection of polypeptide. Food Machin 2004;20:45-7. Chinese..
- Liu H, Feng N, Yuan XB, Yao KD. Preparation and release study of anticancer drug loaded PLA-gelatin nanospheres. Chin J Biomedl Engin 2003;22:178-82. Chinese..
- Wu XS. Preparation, characterization, and drug delivery applications of microspheres based on biodegradable lactic/glycolic acid polymer. In: Wise DL, Trantolo DJ, Altobelli DE, Yaszemski MJ, Gresser JD, Schwartz ER, editors. Encyclopedic Handbook of Biomaterial and Bioengineering. New York: Marcel Dekker; 1995. p1151–250
- Wang HT, Palmer H, Linhardt RJ, Glanagan DR, Schmitt E. Degradation of poly(ester) micro-spheres. Biomaterials 1990;11:679-85.
- Feng XP, Tian QY, Qu HY, Yang Y. The study of bFGF’s activity and stability. Biotechnology 2003;13:32. Chinese..
- Shi M, Yang YY, Chaw CS, Goh SH, Moochhala SM, Ng S, et al. Double walled POE/PLGA microspheres: en-capsulation of water-soluble and water-insoluble proteins and their release pro-perties. J Control Release 2003;89:167-77.
- Lam XM, Duenas ET, Daugherty AL, Levin N, Cleland JL. Sustained release of recombinant human insulin-like growth factor-I for treatment of diabetes. J Control Release 2000;67:281-92.
- Diaz RV, Llabrés M, Évora C. One-month sustained release microspheres of 125I-bovine calcitonin: in vitro-in vivo studies. J Control Release 1999;59:55-62.
- Yoo HS, Lee KH, Oh JE, Park TG. In vitro and in vivo anti-tumor activities of nanoparticles based on doxorubicin-PLGA conjugates. J Control Release 2000;68:419-31.
- Erden N, Celebi N. Factors influencing release of salbutamol sulphate from poly (lactide-co-glycolide) microspheres prepared by water-in-oil-in-water emulsion technique. Int J Pharm 1996;137:57-66.
- Chen GP, Ushida T, Tateishi T. Poly (DL-lactic-co-glycolic acid) sponge hybridized with collagen microsponges and deposited apatite particulates. Poly(DL-lactic-co-glycolic acid) sponge hybridized with collagen microsponges and deposited apatite particulates. J Biomed Mater Res 2001;57:8-14.
- De Gregorio G, Garc A, Lapez-Cabarcos E, Castro RM. Study of poly(lactic-co-glycolic acid) interactions with collagen. Eur Polymer J 2005;41:2416-21.
- Witschi C, Doelker E. Influence of the microencapsulation method and peptide loading on poly (lactic acid) and poly (lactic-co-glycolic acid) degradation during in vitro testing. J Control Release 1998;51:327-41.
- Wang YM, Sato H, Horikoshi I. In vitro and in vivo evaluation of taxol release from poly (lactic-co-glycolic acid) microspheres containing iso-propyl myristate and degradation of the microspheres. J Control Release 1997;49:157-66.
- Rothen-Weinhold A, Besseghir K, De Zelicourt Y, Gurny R. Development and evaluation in vivo of a long-term delivery system for vapreotide, a somatostatin analogue. J Control Release 1998;52:205-21.