Passive sensitization increases histamine-stimulated calcium signaling and NF-κB transcription activity in bronchial epithelial cells1
Introduction
Asthma is best described as a chronic disease that involves airway inflammation and bronchial hyperrespon-siveness, in which the inflammatory mediators are produced mainly by mast cells, macrophages, eosinophils, and lymphocytes. Bronchial epithelial cells directly take part in the pathogenesis of airway inflammation and bronchial hyperresponsiveness[1].
Stimulated by histamine released from mast cells, airway epithelial cells have been shown to produce numerous inflammatory mediators, such as platelet activating factor[2], tumor necrosis factor (TNF)[2], macrophage chemotactic protein (MCP)-1[2], prostaglandins[3], endothelin-1[4], interleukin-1[5], interleukin-6[5], interleukin-8[5], interleukin-16[5]. These mediators may affect the tone of airway smooth muscle, contribute to the local accumulation of inflammatory cells, and act on epithelial cells themselves, thus promoting the chronic airway inflammation. In patients with bronchial asthma, the airway epithelium becomes more sensitive to exogenous or endogenous stimuli, which leads to the increased expression of cytokines and chemoattractants, contributing to exorbitant airway inflammation and bronchial hyperrespon-siveness[1]. However, the mechanism of the hypersensitization of asthmatic airway epithelium is far from being fully understood.
In 1998, Dolmetsch et al and Li et al simultaneously reported that “artificial” calcium oscillations could increase or optimize the gene expression in a frequency-decoding manner[6–8]. Later, Hu et al proved this in receptor mediated calcium oscillations[9]. They found that a decrease in the frequency of [Ca2+]i oscillations during low-level histamine stimulation resulted in a parallel decrease in transcription activity. The regulation of nuclear transcriptional activity by the frequency of cytosolic Ca2+ oscillations may provide cells with a specific mechanism to control the gene expression during agonist stimulation.
These novel findings shed light on the study of diseases related to hypersensitivity. The frequency of calcium oscillation may therefore be used as an indicator of the inner sensitivity of cells. To testify if hypersensitized epithelium in patients with bronchial asthma displays a higher calcium oscillation frequency during agonist stimulation, we measured the alterations of intracellular Ca2+ kinetics caused by different concentrations of histamine in bronchial epithelial cells preincubated with serum either from sensitized or nonsensitized guinea pigs. To further clarify the relevance of the alterations of calcium signaling to asthma, the activity of the calcium dependent transcription factor, nuclear factor of kappaB (NF-κB), also the most important transcription factor involved in airway inflammation, was examined.
Materials and methods
Airway sensitization of guinea pigs and preparation of sensitized serum According to the protocol reported previously, the guinea pigs were sensitized[10]. Briefly, 20 male guinea pigs [400–500 g, conventional animal, certificate N
In the sensitized group, the animals were sensitized by intraperitoneal injection of 1 mL 10% ovalbumin (Sigma) in phosphate buffered saline (PBS) on d 1 and d 8. From d 15, the animals were provoked by exposure to 1% ovalbumin aerosol in PBS generated from supersonic nebulae with a mask for about 30 s until asthma-like attacks occurred for another 2 weeks. The airway responsiveness to histamine was evaluated in the guinea pigs within 24 h after the last provocation, as previously reported[11].
In the nonsensitized group, the animals were treated with PBS under a same procedure.
The sensitized guinea pigs were anaesthetized with 20% urethane with a dose of 6 mL/kg of body weight. Blood was then collected within 24 h after the last provocation through a carotid intubation. The serum was obtained from the supernatant of a centrifugation of blood at 93×g for 10 min and was stored -80 ºC. The guinea pigs were then killed with overdoses (1.2 mL/100 g) of 20% urethane, the lungs were fixed with 10% formaldehyde and followed with an HE staining.
Porcine bronchial epithelial cell culture The method for isolation and culture of porcine bronchial epithelial cells (PBEC) has been described previously[12,13], but was used with modification. Cells were isolated from bronchia of 10- to 12-month-old pigs by casein proprotease and cultured in flasks pre-coated with rat-tail collagen. The medium was DMEM/F-12 (GIBCO, Grand Island, NY, USA) supplemented with 5% fetal bovine serum (GIBCO), 5 µg/mL insulin (Sigma, St Louis, MO, USA), 10 µg/mL transferring (Sigma), 0.5 µg/mL hydrocortisone (Sigma), 10 ng/mL epidermal growth factor (EGF; Sigma), 100 nmol/L retinoic acid (Sigma), 0.5 mg/mL bovine serum albumin (Sigma), and antibiotics (100 IU/mL penicillin and 100 µg/mL streptomycin). After incubation for 24 h, primary cultured cells were propagated in monolayer cultures seeded on glass coverslips pre-coated with rat-tail collagen in culture utensil. The first passages of cells were used in the present study.
Passive sensitization of PBEC When PBEC grew to approximately 50%–60% confluence, the culture medium DMEM/F-12 was removed and sensitized or nonsensitized serum was added for substitution. Cells were incubated for another 24 h[14]. The PBEC were therefore divided into 2 groups. In the group S, the cells were preincubated with sensitized serum. In the group N, the cells were preincubated with nonsensitized serum.
Intracellular free Ca2+ measurement The method for intracellular free Ca2+ measurement has been described previously[15]. Briefly, to measure [Ca2+]i, PBEC monolayers on glass coverslips were incubated with sensitized or nonsen-sitized serum containing 2 µmol/L Fura-2 (acetoxymethyl ester form; Calbiochem, La Jolla, Calif, USA) in 37 ºC 5% CO2 atmosphere for 40 min. The coverslips were then gently washed 3 times with indicator-free Hepes buffered saline (HBS) containing: NaCl 140 mmol/L, KCl 4.5 mmol/L, CaCl2 1.5 mmol/L, MgSO4 1.0 mmol/L, D-glucose 10 mmol/L, and HEPES 21 mmol/L, pH 7.40, at room temperature to allow deesterification of the indicator. Glass coverslips were gently transferred to a perfusion chamber mounted on the stage of an inverted epifluorescence microscope (I×70; Olympus, Tokyo, Japan). Monolayers were first exposed to HBS for equilibrium and then histamine was added to a final concentration of 0.1, 1, or 10 µmol/L. Fura-2 fluorescence was recorded on a field of 1–7 connected cells of a subconfluent PBEC monolayer. Fura-2 fluorescence was alternatively excited at 340 nm and 380 nm using a polychrome (Photonics, Munich, Germany) corresponding to the Ca2+-bound and -free forms of the indicator, respectively. Emitted fluorescence through bandpass interference filters (Photonics) with selected wavelength bands at 510 nm was captured by a computer coupled device (CCD, Imago-QE; Photonics) and transferred to Till-vision software (Photonics). Autofluo-rescence from unloaded PBEC was subtracted from Fura-2 fluorescence recordings before the calculation of the ratio of the emitted fluorescence intensity excited at 340 nm and 380 nm, respectively (F340/F380). Because the dissociation constant (Kd) of Fura-2 for Ca2+ in PBEC may be different from that obtained in vitro[16] and because of the general uncertainties of the calibration techniques of [Ca2+]i measurement[17], the absolute amount of [Ca2+]i was not calculated and therefore F340/F380 was used as a relative indicator of [Ca2+]i in this study.
NF-κB activity assay The NF-κB activity assay was carried out according to an ELISA-based method described previously[18,19]. In brief, after histamine stimulation for 30 min, cells were rinsed twice with cold PBS, detached with trypsin and centrifuged for 10 min at 93×g. The pellet was then resuspended in 100 µL lysis buffer (20 mmol/L HEPES pH 7.5, 0.35 mol/L NaCl, 20% glycerol, 1% NP-40, 1 mmol/L MgCl2, 0.5 mmol/L EDTA, 0.1 mmol/L EGTA) containing a protease inhibitor cocktail (92121; Calbiochem, San Diego, CA, USA). After incubating on ice for 10 min, the lysate was centrifuged for 20 min at 17 968×g. The supernatant constituted the total protein extract. After being quantified with BCA reagent (61105; Pierce, Rockford, IL, USA), the cell extract was kept frozen at -80 ºC until NF-κB activity measurement. Cell extracts were incubated in a 96-well plate coated with the oligonucleotide containing the NF-κB consensus-binding site (5'-GGGACTTTCC-3'). Activated transcription factors from extracts specifically bound to the respective immobilized oligonucleotide. NF-κB activity was then detected with the primary antibody to NF-κB p65 and secondary antibody conjugated to horseradish peroxidase. NF-κB activity was finally determined as absorbance values measured with a microplate reader at the wavelength of 450 nm.
Data analysis and statistics All data were expressed as means±SD; t-test was used to evaluate the significance of differences in calcium oscillation amplitude and frequency between different groups. ANOVA in SPSS12.0 was used to evaluate the significance of differences in NF-κB activity between multiple groups, with P<0.05 as the level of significance.
Results
HE staining and airway responsiveness In sensitized guinea pig lungs, there was increased accumulation of lymphocytes, eosinophils, neutrophils both in the interstitial and bronchia. Airway smooth muscle became significantly hypertrophic. In some lungs, the increase in size of the submucosal glands and thickening of basement membrane of bronchial epithelium could be seen, while others displayed epithelial cell sloughing or cilia cell disruption (Figure 1 A, 1B, 1C).
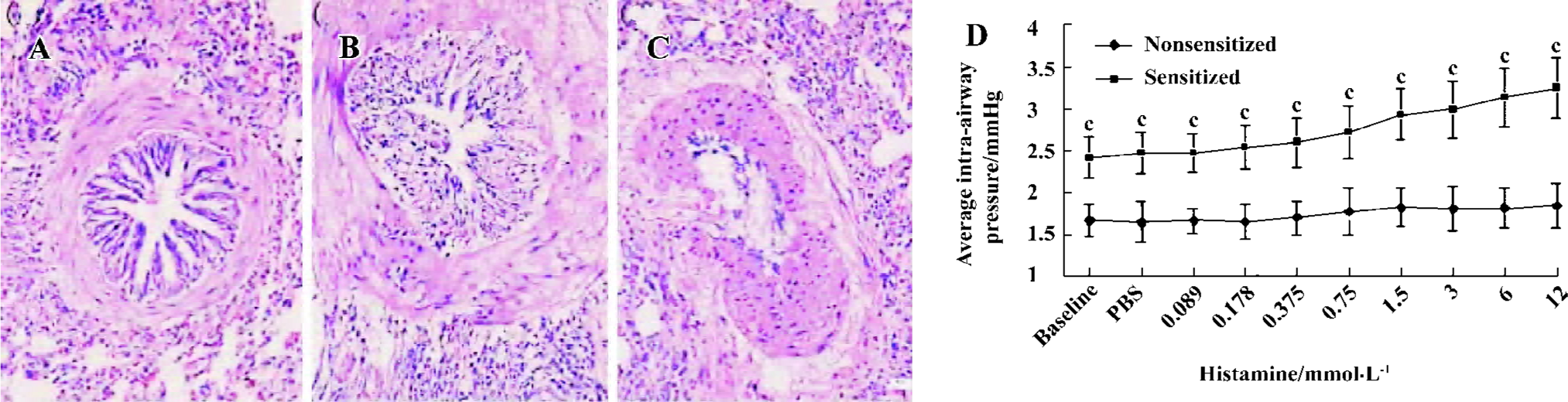
The baseline of the average intra-airway pressure in sensitized guinea pigs was significantly higher than the nonsensitized guinea pigs (2.41±0.24 vs 1.66±0.20 mmHg, P<0.01, n=3). The PC20 (the minimum concentration of histamine required for increasing 20% of the average intra-airway pressure) of nonsensitized guinea pigs did not appear in the concentrations of histamine used. The PC20 of sensitized guinea pigs appeared at 1.5 mmol/L histamine (Figure 1D).
Effect of passive sensitization on 0.1 µmol/L histamine-induced calcium signaling in PBEC In the PBEC of group N, 0.1 µmol/L histamine did not induce any oscillation of [Ca2+]i in all monolayers studied (n=6). In the PBEC of group S, 0.1 µmol/L histamine induced [Ca2+]i oscillations in 4 of 6 monolayers studied (Figure 2).
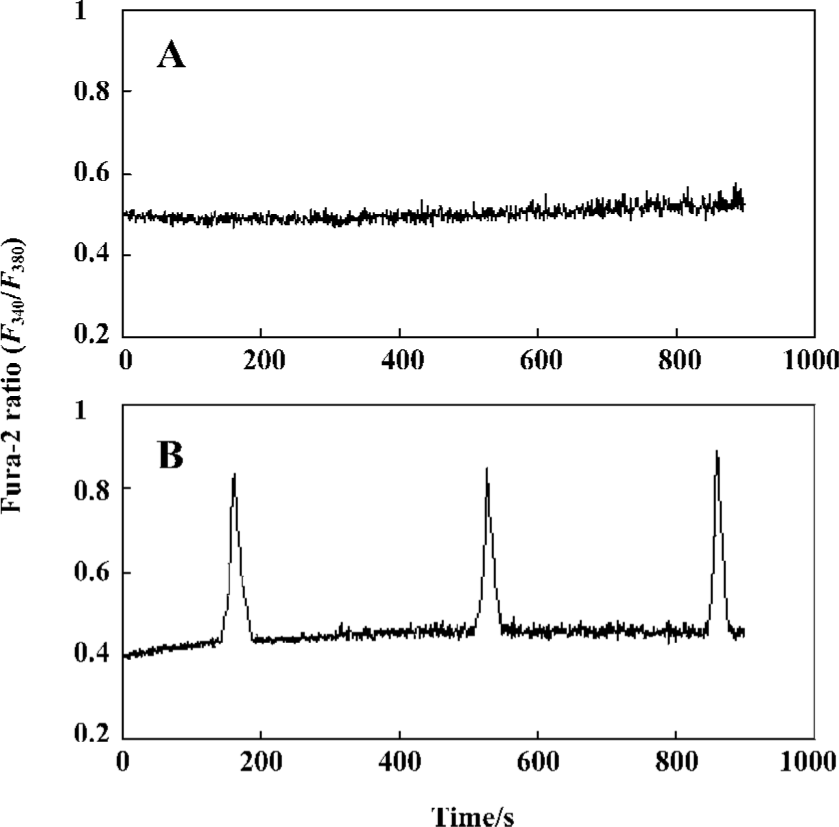
Effect of passive sensitization on 1 µmol/L histamine-stimulated calcium signaling in PBEC During 1 µmol/L histamine stimulation, repetitive [Ca2+]i oscillations were observed in all monolayers studied in the PBEC of group N and group S (n=6 for each). The average [Ca2+]i oscillation amplitude in PBEC of group N and group S was 0.8471±0.0391 and 0.8700±0.0335, respectively. There was no significant increase in [Ca2+]i oscillation amplitude after passive sensitization (P>0.05). The average [Ca2+]i oscillation frequency in PBEC of group N and group S was 0.0069±0.0007 and 0.0100±0.0013, respectively. There was a significant increase in [Ca2+]i oscillation frequency after passive sensitization (P<0.01) (Figure 3).
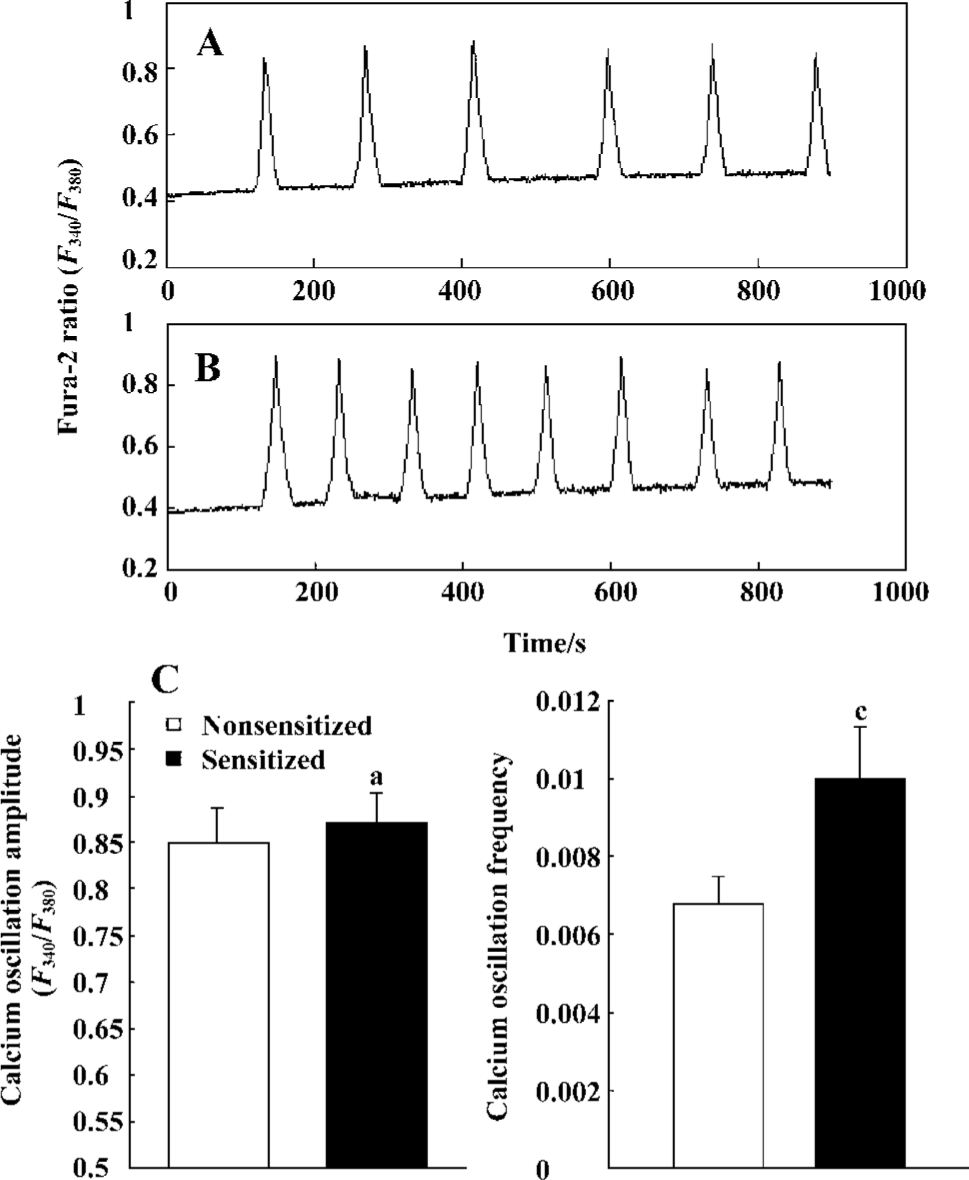
Effect of passive sensitization on 10 µmol/L histamine-stimulated calcium signaling in PBEC When PBEC were stimulated with 10 µmol/L histamine, a transient initial increase (TII) followed by a sustained elevation of [Ca2+]i was observed in monolayers of group N and group S (n=6 for each). There was no significant difference in the amplitude of the TII in PBEC between group N and group S (0.9532±0.0367 vs 0.9831±0.0318, P>0.05, n=6); The average amplitude of following sustained elevation (FSE) in PBEC was significantly increased in group S as compared with that in group N (0.6076±0.0274 vs 0.6559±0.0243, P<0.01, n=6) (Figure 4).
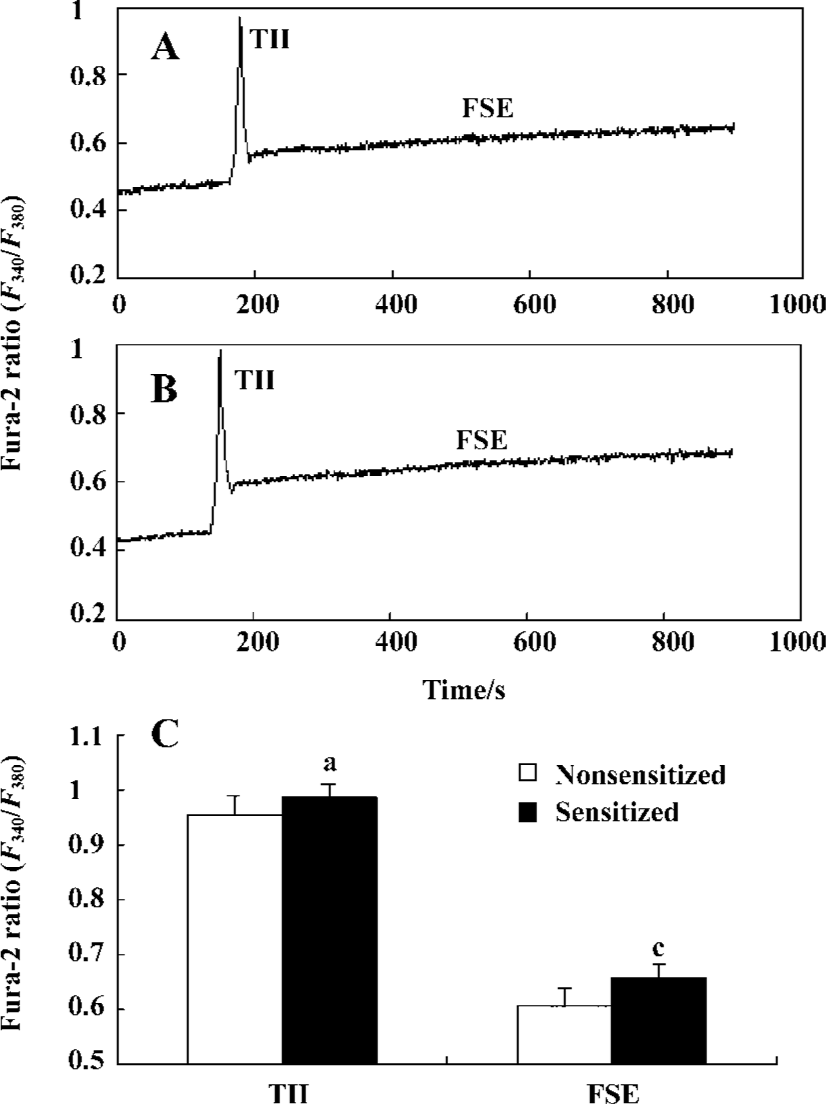
Histamine-stimulated NF-κB activity in sensitized and nonsensitized PBEC There was very low level of basic NF-κB activity in the PBEC of groups N and S (0.183±0.016 vs 0.190±0.004, n=3, P>0.05). Histamine 0.1 µmol/L did not increase NF-κB activity of PBEC in group N (0.183±0.016 vs 0.197±0.013 before and after administration, respectively; n=3, P>0.05). In contrast, Histamine 0.1 µmol/L significantly increased the NF-κB activity of PBEC in group S (0.19±0.004 vs 0.412±0.083 before and after administration, respectively; n=3, P<0.05). Histamine 1 µmol/L significantly increased NF-κB activity of PBEC in group N and group S, and the NF-κB activity in group S was significantly higher than that in group N (1.178±0.095 vs 0.873±0.069, n=3, P<0.05). Histamine10 µmol/L also significantly increased the NF-κB activity of PBEC in group N and group S, and the NF-κB activity in group S was higher than that in group N, but the difference was not significant (1.226±0.086 vs 1.373±0.11, n=3, P>0.05) (Figure 5).
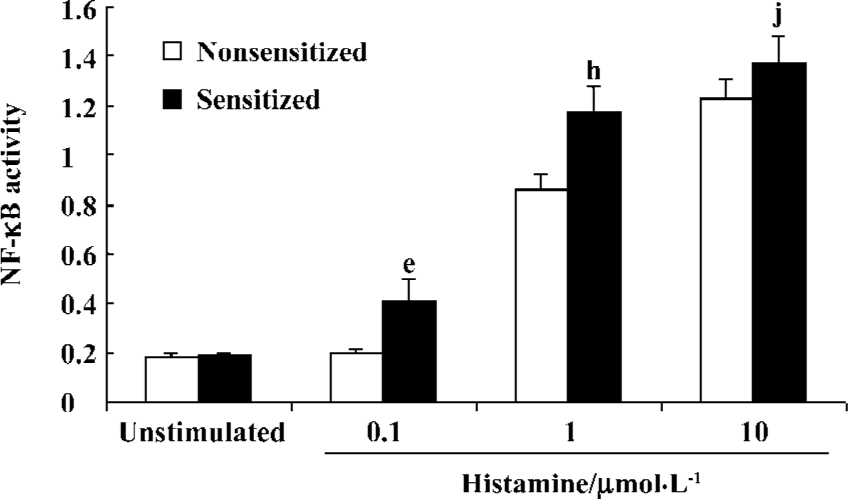
Discussion
Lung HE staining and airway responsiveness indicated the existence of airway inflammation and hyperrespon-siveness in ovalbumin sensitized guinea pigs. This demonstrated that the asthma model employed in the present study was feasible.
Passive sensitization provides a useful model for the understanding of asthma at a cellular level. This technique involves the incubation of bronchial epithelial or smooth muscle cells in culture with serum from patients with allergic asthma or, alternatively, serum from nonallergic nonasthmatic patients[20].
The results in this study showed that histamine could induce calcium oscillations in PBEC. Histamine 0.1 µmol/L could induce [Ca2+]i oscillations of PBEC in group S, but not in group N. Histamine 1 µmol/L could induce [Ca2+]i oscillations of PBEC in both group S and group N. The [Ca2+]i oscillation frequency was significantly higher in PBEC of group S than in group N. These results demonstrated that passive sensitization might increase histamine-induced calcium oscillation frequency in PBEC. PBEC becomes more sensitive to histamine after sensitization. The data presented here provide direct evidence of the existence of hypersensitivity in calcium signaling in passive sensitized PBEC. The subsequent calcium-regulated NF-κB activity was in accordance to the calcium oscillation frequency in 0.1 µmol/L and 1 µmol/L histamine-stimulated PBEC.
The allergic serum contains a high concentration of total IgE[21,22] and cytokines such as IL-1β[23], TNF-α[24,25], and so on. Hakonarson and Grunstein also reported that passive sensitization of human bronchus could markedly increase the expression of IL-1β as well as the mRNA for the low-affinity IgE and these cytokines may increase the reactive oxygen species (ROS) production in PBEC. ROS generation in cells has been observed after stimulation by many cytokines, including IL-1β[26] and TNF-α[27–30]. It has been proved that ROS could increase the sensitivity of endoplasmic reticulum (ER) Ca2+ stores to inositol 1,4,5-trisphosphate (Ins-1,4,5-P3)[31], which may lead to an increased calcium oscillation frequency[32,33].
The finding that passive sensitization may increase histamine-stimulated calcium oscillation frequency in PBEC has important significance in the understanding of asthma pathophysiology. As gene transcription was regulated by calcium oscillation frequency[6–9], the increased histamine-induced calcium oscillation frequency led to a parallel elevation of NF-κB activity in 0.1 µmol/L and 1 µmol/L histamine-stimulated PBEC. It is known now that NF-κB is the most important transcription factor involved in the pathogenesis of asthma[34,35] and that it can drive the expression of cytokines such as IL-1β, TNF-α, and so on, in airway epithelium. This leads to the formation of positive feedback and ultimately contributes to exorbitant inflammation in asthma. The finding that sensitized cells prone to generate calcium oscillations or displaying higher calcium oscillation frequency during agonist stimulation might also be observed in other sensitized target cells involved in allergic diseases such as allergic rhinitis, eczema, and so on, and that they might also be a universal feature of allergic diseases with hypersensitivity in target cells. Intervention concerning the calcium oscillation frequency might be a kind of strategy to control allergic diseases in the future.
Histamine 10 µmol/L could increase the [Ca2+]i to a higher plateau in passive sensitized PBEC than in nonsensitized PBEC. These results demonstrated that passive sensitized PBEC developed an increasing tendency to form calcium overload during a higher concentration of agonist stimulation. As calcium overload may mediate injury, sensitized PBEC become more susceptible to stimuli. This may contribute to the epithelial cell sloughing, cilia cell disruption, which was frequently observed in late stage asthmatic lung. However, there was no significant difference in the NF-κB activity of PBEC between the two groups, which might be due to the fact that the frequency-decoding manner of calcium-regulated gene expression was more effective than the amplitude-decoding manner.
Acknowledgements
We thank Ms Li-hong LONG, Ms Zhong-lin ZHU, and Mr Zhou-huan WU in Department of Pharmacology of Tongji Medical College for their technical assistance.
References
- Fireman P. Understanding asthma pathophysiology. Allergy Asthma Proc 2003;24:79-83.
- Holtzman MJ, Ferdman B, Bohrer A, Turk J. Synthesis of the 1-o-Hexadecyl molecular species of platelet-activating factor by airway epithelial and vascular endothelial cells. Biochem Biophys Res Commun 1991;177:357-64.
- Churchill L, Chilton FH, Resau JH, Bascom R, Hubbard WC, Proud D. Cyclooxygenase metabolism of endogenous arachidonic acid by cultured human tracheal epithelial cells. Am Rev Respir Dis 1989;140:449-59.
- Ackerman V, Carpi S, Bellini A, Vassalli G, Marini M, Mattoli S. Constitutive expression of endothelin in bronchial epithelial cells of patients with symptomatic and asymptomatic asthma and modulation by histamine and interleukin-1. J Allergy Clin Immunol 1995;96:618-27.
- Arima M, Plitt J, Stellato C, Bickel C, Motojima S, Makino S, et al. Expression of interleukin-16 human epithelial cells. Inhibition by dexamethasone. Am J Respir Cell Mol Biol 1999;21:684-92.
- Dolmetsch RE, Xu K, Lewis RS. Calcium oscillations increase the efficiency and specificity of gene expression. Nature 1998;6679:933-6.
- Li W, Llopis J, Whitney M, Zlokarnik G, Tsien RY. Cell-permeant caged InsP3 ester shows that Ca2+ spike frequency can optimize gene expression. Nature 1998;392:936-41.
- Meldolesi J. Oscillation, activation, expression. Nature 1998;392:863-6.
- Hu Q, Deshpande S, Irani K, Ziegelstein RC. [Ca2+]i oscillation frequency regulates agonist-stimulated NF-kappaB transcriptional activity. J Biol Chem 1999;274:33995-8.
- Ouyang NT, Liu ZH, Zhong NS. Distribution of endothelin-1 in asthmatic guinea pigs. Zhonghua Jie He He Hu Xi Za Zhi 1995;18:151. Chinese..
- Wang CZ, Guo XJ, Wang SC. A guinea pig model to determine airway responsiveness in asthma. J 3rd Military Med Univ 1994; 16: 277–8. Chinese.
- Wu R, Yankaskas J, Cheng E, Knowles MR, Boucher R. Growth and differentiation of human nasal epithelial cells in culture. Serum-free, hormone-supplemented medium and proteoglycan synthesis. Am Rev Respir Dis 1985;132:311-20.
- Chen F, Wu R, Wang X. Effects of cigarette smoke extract on the expression of β-catenin and tyrosine phosphorylation in airway epithelial cell. Zhong Hua Yi Xue Za Zhi 2001;81:406. Chinese..
- Hakonarson H, Maskeri N, Carter C, Grunstein MM. Regulation of TH1- and TH2-type cytokine expression and action in atopic asthmatic sensitized airway smooth muscle. J Clin Invest 1999;103:1077-87.
- Jin S, Chen JG, Zhu LP, Liu SY, Wang DX, Hu Q. Subacute mild hypoxia increases histamine-stimulated calcium oscillation frequency in pulmonary artery endothelial cells. Prog Biochem Biophy 2005;32:551-6.
- Konishi M, Olson A, Hollingworth S, Baylor SM. Myoplasmic binding of Fura-2 investigated by steady-state fluorescence and absorbance measurements. Biophys J 1988;54:1089-104.
- Paltauf-Doburzynska J, Frieden M, Spitaler M, Graier WF. Histamine-induced Ca2+ oscillations in a human endothelial cell line depend on transmembrane ion flux, ryanodine receptors and endoplasmic reticulum Ca2+-ATPase. J Physiol 2000;524:701-13.
- Renard P, Ernest I, Houbion A, Art M, Calvez HL, Raes M, et al. Development of a sensitive multi-well colorimetric assay for active NF-κB. Nucleic Acids Res 2001;29:e21.
- Jin S, Lu DQ, Ye SQ, Ye H, Zhu LP, Feng ZH, et al. A simplified probe preparation for ELISA-based NF-κB activity assay. J Biochem Biophys Methods 2005;65:20-9.
- Black JL, Johnson PR. What determines asthma phenotype? Is it the interaction between allergy and the smooth muscle? Am J Respir Crit Care Med 2000;161:S207-10.
- Black JL, Marthan R, Armour CL, Johnson PR. Sensitization alters contractile responses and calcium influx in human airway smooth muscle. J Allergy Clin Immunol 1989;84:440-7.
- Li C, Hu BC, Long ZJ. Effects of the decoction of Yumian Anti-asthma Formula on general serum IgE contents of asthmatic guinea pigs. Jiangsu Yao Xue Yu Lin Chuang Za Zhi 2001;9:14-6. Chinese..
- Hakonarson H, Herrick DJ, Serrano PG, Grunstein MM. Autocrine role of interleukin 1β in altered responsiveness of atopic asthmatic sensitized airway smooth muscle. J Clin Invest 1997;99:117-24.
- Hirata N, Kohrogi H, Iwagoe H, Goto E, Hamamoto J, Fujii K, et al. Allergen exposure induces the expression of endothelial adhesion molecules in passively sensitized human bronchus: time course and the role of cytokines. Am J Respir Cell Mol Biol 1998;18:12-20.
- Hakonarson H, Grunstein MM. Autologously up-regulated Fc receptor expression and action in airway smooth muscle mediates its altered responsiveness in the atopic asthmatic sensitized state. Proc Natl Acad Sci USA 1998;95:5257-62.
- Matsubara T, Ziff M. Increased superoxide anion release from human endothelial cells in response to cytokines. J Immunol 1986;137:3295-8.
- Toborek M, Blanc EM, Kaiser S, Mattson MP, Hennig B. Linoleic acid potentiates TNF-mediated oxidative stress, disruption of calcium homeostasis, and apoptosis of cultured vascular endothelial cells. J Lipid Res 1997;38:2155-67.
- Murphy HS, Shayman JA, Till GO, Mahrougui M, Owens CB, Ryan US, et al. Superoxide responses of endothelial cells to C5a and TNF-alpha: divergent signal transduction pathways. Am J Physiol 1992;263:L51-9.
- Deshpande SS, Angkeow P, Huang J, Ozaki M, Irani K. Rac1 inhibits TNF-α-induced endothelial cell apoptosis: dual regulation by reactive oxygen species. FASEB J 2000;14:1705-14.
- Thabut G, El-Benna J, Samb A, Corda S, Megret J, Leseche G, et al. Tumor necrosis factor-alpha increases airway smooth muscle oxidants production through a NADPH oxidase-like system to enhance myosin light chain phosphorylation and contractility. J Biol Chem 2002;277:22814-21.
- Hu Q, Zheng G, Zweier JL, Deshpande S, Irani K, Ziegelstein RC. NADPH oxidase activation increases the sensitivity of intracellular Ca2+ stores to inositol 1,4,5-trisphosphate in human endothelial cells. J Biol Chem 2000;275:15749-57.
- Hu Q, Yu ZX, Ferrans VJ, Takeda K, Irani K, Ziegelstein RC. Critical role of NADPH oxidase-derived reactive oxygen species in generating Ca2+ oscillations in human aortic endothelial cells stimulated by histamine. J Biol Chem 2002;277:32546-51.
- Lounsbury KM, Hu Q, Ziegelstein RC. Calcium signaling and oxidant stress in the vasculature. Free Radic Biol Med 2000;28:1362-9.
- Hart LA, Krishnan VL, Adcock IM, Barnes PJ, Chung KF. Activation and localization of transcription factor, nuclear factor-kappaB, in asthma. Am J Respir Crit Care Med 1998;158:1585-92.
- Poynter ME, Irvin CG, Janssen-Heininger YM. Rapid activation of nuclear factor-kappaB in airway epithelium in a murine model of allergic airway inflammation. Am J Pathol 2002;160:1325-34.