Effect of protein kinase C alpha, caspase-3, and survivin on apoptosis of oral cancer cells induced by staurosporine1
Introduction
Cell proliferation, differentiation and apoptosis are central features of tissue homeostasis, and inhibition of apoptosis might be involved in the pathogenesis of cancer by prolonging cell life and sustaining growth of malignant tissues[1,2]. Protein kinase C (PKC) is a family of phospholipid-dependent serine/threonine kinases and participates in many cellular responses, including cell apoptosis, which can be inhibited by staurosporine[3–5]. The family contains at least 12 isoforms, and specific roles in cell cycle progression and in apoptosis have been hypothesized for the different PKC isoenzymes. Of these PKC isoforms, PKCα is distributed in almost all tissues and is involved in various signal transductions. Considerable evidence suggests that PKCα plays an important role in the apoptosis of some tumor cells and that the inhibition of PKCα might induce apoptosis[5,6]. In contrast, one recent study reported that elevated expression of PKCα might promote the apoptosis of gastric cancer cells[7]. However, little is known about the protein level and subcellular distribution of PKC α in the apoptosis of oral cancer cells. Elucidating the patterns of PKCα action will lead to a better understanding of the molecular mechanism of the PKCα signal pathway involved in the apoptosis of oral cancer cells.
The activation of caspases, particularly caspase-3, appears to be a central part in most apoptotic pathways and most types of cells[8,9]. Caspases have been shown to be cleaved and possibly activated by PKCs suggesting that they act downstream of PKCs as effectors in the apoptotic machinery[6]. Survivin, a recently characterized inhibitor of apoptosis protein (IAP), is abundantly expressed in oral squamous cell carcinoma (OSCC), but undetectable in normal oral tissues[10,11], suggesting a potential role in oral carcinogenesis. However, no direct molecular interaction has been described so far between PKCα, survivin and caspase-3 in mediating apoptosis of oral cancer cells.
In the present study, we investigated the functional and molecular interactions among PKCα, survivin and caspase-3 in apoptosis induced by staurosporine with the aim of further highlighting their relevance in OSCC biology.
Materials and methods
Reagents Fetal bovine serum (FBS) and Dulbecco’s modified Eagle’s medium (DMEM) were obtained from Gibco Laboratories (Gibro/BRL, Grand Island, NY). MTT, acridine orange (AO), ethidium bromide (EB), propidium iodide (PI) and staurosporine were obtained from Sigma Biotechnologies (Sigma-Aldrich, Inc, Saint Louis, Missouri, USA). Staurosporine was dissolved in ethanol to make a stock solution of 1 mmol/L and diluted to their final concentrations in the culture medium. The final concentration of ethanol never exceeded 0.01%, a concentration at which there is no discernible effect on tongue squamous cell carcinoma (TSCCa) cells in comparison with the control. Primary antibodies including mouse monoclonal antibodies to PKCα (H-7, sc-8393), β-actin (C-2, sc-8432), caspase-3 (E-8, sc-7272) and rabbit polyclonal antibody against survivin (FL-142, sc-1081) were obtained from Santa Cruz Biotechnology (Santa Cruz, California, USA). An enhanced chemiluminescent detection system (ECL kit) from Amersham international Plc (Amersham Biosciences, Buckinghamshire, UK) was used for Western blot analysis. All other reagents were analytical reagents.
Cell culture TSCCa cell line was established by Dr Hui-xi JIN from a patient with OSCC of the tongue[12]. TSCCa cells were cultured in DMEM, supplemented with 10% heat-inactivated FBS, 100 kU/L penicillin, and 0.1 g/L streptomycin. The cells were maintained at 37 °C in a humidified atmosphere of 5% CO2. TSCCa cells were cultured and treated with either 0.01% ethanol (vehicle) or 1–100 nmol/L stauro-sporine. The treated cells were examined by phase contrast microscope (Nikon Instech Co Ltd, Kanagawa, Japan) for evidence of morphological changes induced by staurosporine treatment.
MTT assay Cell viability and activity were detected using MTT dye assay, in which the dye was converted into formazan granules in the presence of reactive oxygen. TSCCa cells were plated at a density of 5×103 cells per well on a 96-well plate. At 24 h after seeding, straurosporine (1–100 nmol/L) was added to the culture medium. At 6 h, 12 h, and 24 h after drug treatment, MTT was added to each well at a concentration of 500 mg/L and incubated for 4 h at 37 °C. After that, media were aspirated and cells were solubilized in 400 μL Me2SO. Cells were incubated for 10 min at 37 °C with gentle shaking. Absorbance was measured at 540 nm using a computerized microplate analyzer.
AO/EB staining To assess apoptosis, cells were stained with AO/EB. In brief, after TSCCa cells were treated with 100 nmol/L straurosporine for 24 h, nonadherent cells in the medium and trypsinized adherent cells were centrifuged at 200×g for 10 min at 4 °C and all but 50 µL media was removed. The pellet was resuspended with 2 µL for each 0.1 g/L AO and EB. Cells were immediately viewed using a fluorescence microscope (Leica Microsystems AG, Wetzlar, Germany). Morphology was defined according to descriptions from Kern and Kehrer for general apoptotic characteristics[13].
Flow cytometry For flow cytometric assessment of apoptosis and cell cycle phases, TSCCa cells were fixed with ice-cold 75% ethanol following 100 nmol/L staurosporine treatment for 6 h, 12 h or 24 h, and washed twice with phosphate-buffered saline (PBS). Cells were incubated at 37 °C for 30 min in PBS containing 1 g/L RNase, then stained with 100 mg/L PI. Cellular DNA content was measured by Beckman Coulter Epics Altra II cytometer (Beckman Coulter, California, USA).
PKCα subcellular fractionation and Western blot analysis TSCCa cells treated with 100 nmol/L staurosporine for 12 h and 24 h were washed twice with PBS and were then scraped from the culture vessels and collected. Harvested cells were suspended in 0.5 mL of hypotonic buffer [10 mmol/L N-2-hydroxyethylpiperazine-N'-2-ethanesulfonic acid (HEPES, pH 7.4), 1.5 mmol/L MgCl2, 10 mmol/L KCl, 0.2 mmol/L phenyl-methylsulfonyl fluoride (PMSF), 0.5 mmol/L DTT] and homo-genized with a Dounce homogenizer at 4°C. Unlysed cells, nuclei and cell debris were pelleted by centrifugation at 1000×g at 4 °C for 5 min . The supernatant was centrifuged at 100 000×g at 4 °C for 1 h . The 100 000×g supernatant was designated as a cytosol fraction. The 100 000×g pellet was suspended in hypotonic buffer containing 1% Triton X-100 and centrifuged at 10 000×g for 10 min, and the resulting supernatant is referred to as the membrane fraction. Protein samples were mixed with an equal volume of 2×sodium dodecyl sulfate polyacrylamide gel electrophoresis (SDS-PAGE) sample buffer, boiled for 5 min, and then separated using 10% SDS-PAGE gels with 20 µg of protein loaded per lane. After electrophoresis, proteins were transferred to PVDF membranes by semi-dry electrophoretic transfer. The membranes were blocked in 5% dry milk (1 h), rinsed and then incubated with primary antibody of PKCα (1:1000 dilution) overnight at 4 °C. The primary antibody was removed, membranes were washed 4 times, and 0.1 mg/L peroxidase-labeled goat secondary antibody was added for 1 h. Bands were then visualized by ECL kit and exposed to X-ray film. Percentage of membrane or cytosol band was calculated by a Bio-Rad (Richmond, CA) model GS-670 Imaging Densitometer.
Immunocytochemistry TSCCa cells were seeded on 8-chamberred glass slides and treated with 100 nmol/L stauro-sporine for 12 h and 24 h as described above. Cells were fixed for 10 min in 100% methanol at room temperature. Then, cells were washed with PBS, treated with 3% hydrogen peroxide to block endogenous peroxidase activity and incubated with normal serum. After a 2-h incubation with primary antibodies, cells were washed 3 times with PBS and incubated with biotin-labeled anti-mouse or anti-rabbit immuno-globin G for 20 min. After washing 3 times with PBS, cells were stained using the UltraSensitive streptavidin-peroxidase detection system (Maixin Biotechnology, Fuzhou, China). Positive reaction was seen as brown staining. One hundred cells were counted to determine the intensities of protein expression of caspase-3 and survivin by using HPLAS-2000 analysis software (Qianping Biotechnology, Wuhan, China).
Statistical analysis Data were expressed as mean±SD. Statistical significance was assessed with one-way ANOVA followed by Duncan’s multiple-range test. P<0.05 was considered statistically significant.
Results
Effect of staurosporine on cell viability in TSCCa cells In the present study, the effect of staurosporine on TSCCa cell viability was evaluated using a MTT assay. Stauro-sporine exerted a marked dose- and time-dependent inhibitory effect on the viability of TSCCa cells. The effect was observed at 12 h after treatment, and increased with time, so that after 24 h with 100 nmol/L staurosporine cell viability was reduced to only 24% of the control (Figure 1).
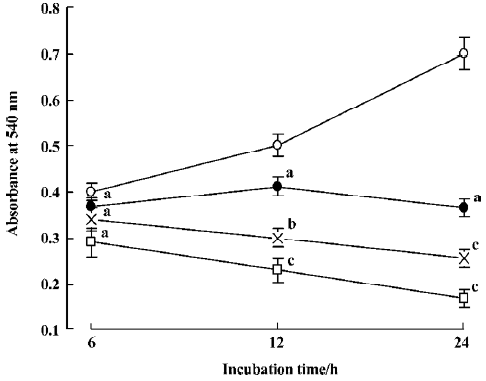
Morphological changes in TSCCa cells induced by staurosporine To address the ability of staurosporine to induce cell death, we first investigated the effect of stauro-sporine on cell morphological changes using a phase contrast microscope. Numerous TSCCa cells exhibited a flat appearance in the absence of staurosporine (Figure 2A). After incubation with 100 nmol/L staurosporine, TSCCa cells presented remarkable morphological changes. TSCCa displayed a long spindle shape 6 h after stimulation (Figure 2B). Then the number of TSCCa cells decreased, with an increased, large amount of intracellular vesicles (Figure 2C). After 24 h of staurosporine stimulation, we observed that treatment resulted in cells exhibiting evidence of apoptosis, including cell detachment, loss of cell processes and membrane shrin-kage, as evidenced by curling up of cells (Figure 2D).
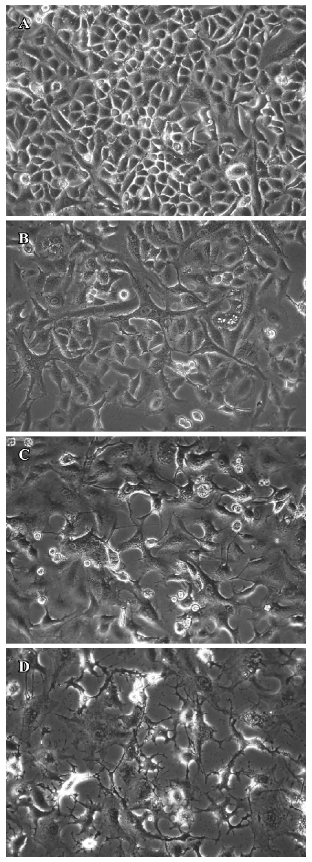
Morphological changes of TSCCa cells were also investigated by AO/EB staining using fluorescence microscopy. Live cells stain only with AO, which shows up green inside the cell. Apoptotic cells induce fragmentation of the yellow organelle. Necrotic cells stain with EB, and are detected by their red color. The images in Figure 3 show that with a dose of 100 nmol/L staurosporine treatment for 24 h, there were increasing amounts of swollen and distorted cells coupled with organelle disintegration and a rise in necrotic cells.
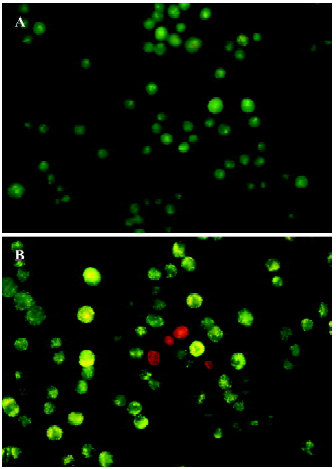
DNA content analysis of TSCCa cells treated with staurosporine To determine the effects of staurosporine on cell cycle progression and apoptosis, TSCCa cells were stained with PI and subjected to flow cytometric analysis. A representative cell cycle profile of control cells is shown in Figure 4A. Treatment with 100 nmol/L staurosporine for various periods of time resulted in changed DNA content profiles. Staurosporine treatment for 6 h and 12 h caused an accumulation of the cells in G2/M phase of approximately 26.6% and 34.0%, respectively; and that of the control was approximately 9.9% (Table 1). This indicates that the appearance of a G2/M arrest of staurosporine in TSCCa cells is time-dependent. With the time prolonged, the G2/M arrest caused by staurosporine released and cells returned to G0/G1 phase and S phase. After 24 h of staurosporine treat-ment, the percentage of cells in G2/M decreased to 19.8%; however, this was still higher than that of the control (Figure 4).
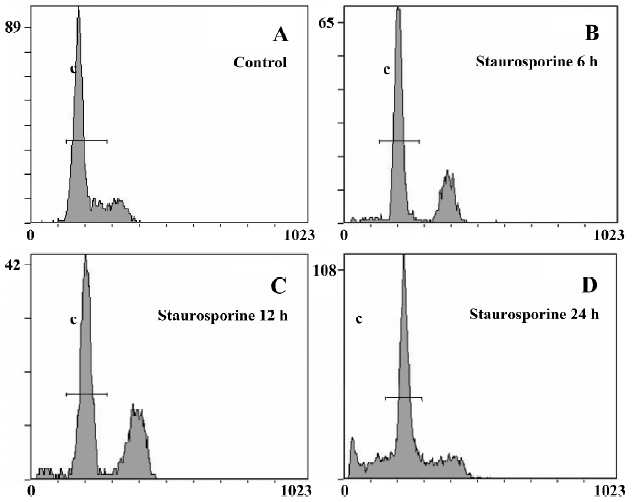
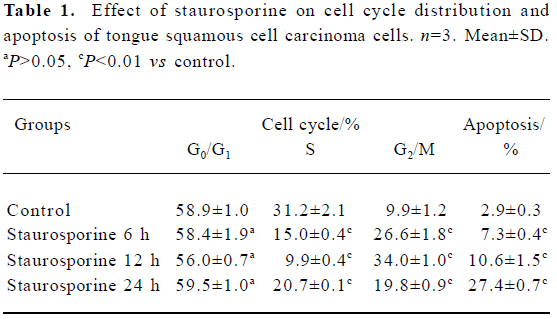
Full table
The percentage of cells or cell fragments with DNA content less than 2 N (hypodiploid or apoptotic shift) increased in a time-dependent manner, from 2.9% in control cultures to approximately 27.4% at 100 nmol/L staurosporine exposure for 24 h (Table 1, Figure 4).
Changes in PKC α content and subcellular distribution in TSCCa cells Because the translocation of PKC α from cytosol to the membrane is a hallmark of PKC α activation, Western blot experiments were performed to detect the protein level of PKC α in cytosol and membrane of TSCCa cells treated with 100 nmol/L staurosporine for different periods of time. We found that PKC α is expressed in both cytosol and membrane in ethanol-treated control TSCCa cells. After treatment with staurosporine for various periods of time, PKC α content in cytosol and membrane decreased in a time-dependent manner (Figure 5). In addition, the percentage of PKC α content in membrane versus cytosol decreased quickly in staurosporine treated cells, from 0.45 in ethanol-treated control cultures to 0.18 at staurosporine exposure for 24 h (Table 2).
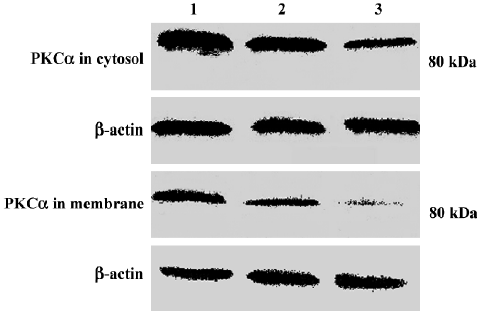
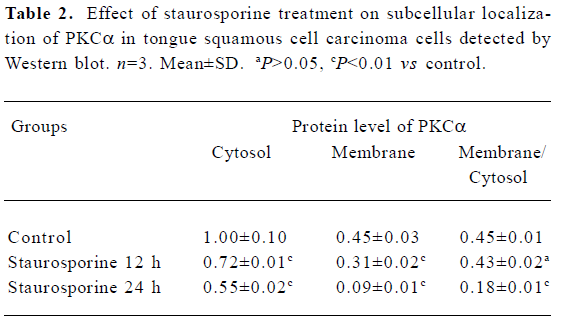
Full table
Effect of staurosporine on the activation of caspase-3 and the protein level of survivin To further characterize the mechanisms that control apoptosis in TSCCa cells, protein levels of caspase-3 and survivin in TSCCa cells treated with 100 nmol/L staurosporine for 12 h and 24 h was measured by immunocytochemical staining. In untreated TSCCa cells, there was a faint brown cytoplasmic staining for caspase-3 (Figure 6Aa). After incubation with staurosporine for 12 h and 24 h, cytoplasmic caspase-3 staining was substantially increased in a time-dependent manner (Figure 6B). TSCCa cells with fusiform shape, numerous intracellular vesicles and shrinking cells showed deep brown cytoplasmic granules of caspase-3 staining (Figure 6Ab).
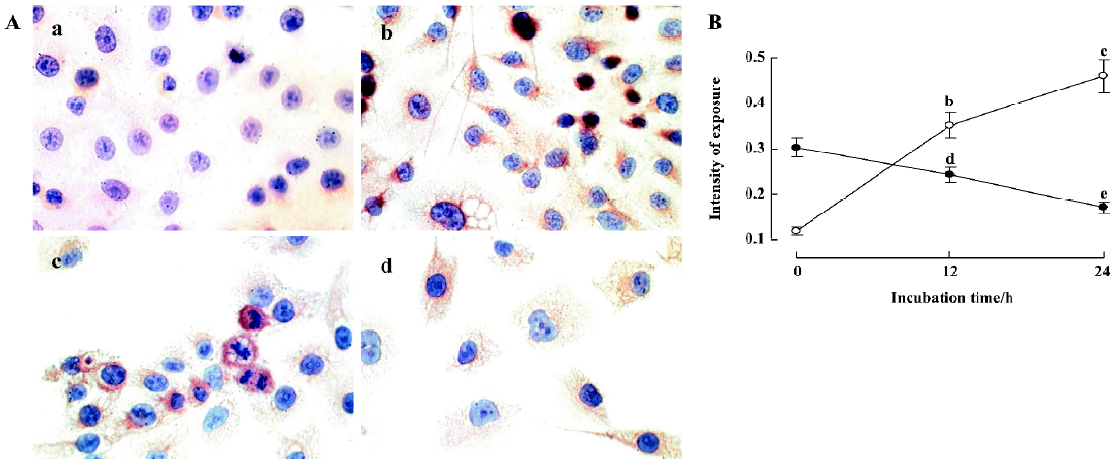
Furthermore, a strong cytoplasmic staining of survivin was observed in control cells, especially in nuclear division cells (Figure 6Ac). After incubation with staurosporine for 24 h, survivin expression was diminished (Figure 6Ad, 6B).
Discussion
The molecular mechanisms associated with apoptosis have been widely explored, but are not yet precisely understood[14]. Staurosporine has been shown to induce apoptosis in a wide variety of cell types, such as rat cardiomyocytes, human dermal papilla fibroblasts and luteinized granulose cells. Therefore, staurosporine-induced apoptosis has been recognized as a useful model for investigating the mechanism of apoptosis in mammalian cells[15]. To assess whether staurosporine induces apoptosis in oral cancer cells, 3 specific methods were used in the present study to evaluate apoptosis. The presented data demonstrate that apoptotic changes induced by staurosporine were confirmed by morphological changes observed under phase contrast micro-scopy, AO/EB staining and flow cytometric analyses of cellular DNA content. A significant proportion of cells at G2/M phase were also observed after 6 h treatment with stauro-sporine, and appeared at peak after 12 h of treatment. With the time prolonged, the G2/M arrest caused by staurosporine released and cells returned to G0/G1 phase and S phase after 24 h of treatment, whereas a significant proportion of cells appeared at sub-G0/G1 apoptotic peak. Another report showed that staurosporine induced apoptosis in Chang liver cells by a mitochondria-caspase-dependent pathway, which can be suppressed by z-VAD-fmk, a general inhibitor of caspases; whereas the arrest of cells in G2/M phase of cell cycle was not modified by z-VAD-fmk, suggesting that apoptosis and G2/M arrest caused by staurosporine might be controlled in different independent pathways[16]. Although cells with typical apoptotic morphology were observed after the treatment, we cannot exclude the possibility that some necrosis occurr-ed with the high staurosporine dose. However, our findings were consistent with other reports showing that stauro-sporine at 100 nmol/L concentration can trigger apoptosis regardless of the cell cycle status[15].
To explore more precisely the mechanisms of stauro-sporine-induced TSCCa cells apoptosis, we investigated whether the PKCα signal pathway was involved, as it has been shown that perturbation of PKCα activity can induce or repress apoptosis[5–7]. We determined, using Western blot analysis, that cultured TSCCa cells expressed PKC α both in cytosol and membrane under 10% serum concentration. When TSCCa cells were treated with staurosporine, content of PKC α in cytosol and membrane decreased dramatically, especially in membrane. One important factor in determining specific functions of PKC isoforms is their intracellular localization. PKC isoforms show different patterns of subcellular localization, which can vary for the various isoforms according to tissue and cell type. PKC α typically reside in the cytosol in an inactive state. After cell stimulation, they are often translocated to other compartments. Our results suggest that inhibition of PKC α translocated from cytosol to membrane might mediate staurosporine-induced TSCCa cells apoptosis. It is not clear, however, how the complex isoform-specific subcellular distribution and stimulus-induced redistribution can be achieved. As staurosporine was a wide inhibitor of PKC, we could not exclude the possibility that other PKC isoenzymes could also mediate staurosporine-induced TSCCa cells’ apoptosis.
Caspase-3 has been reported to play a key role in apoptosis[8,9]. Caspase-3 normally exists in the cytosolic fraction of cells as an inactive precursor, activating proteolytically when cells are signaled to undergo apoptosis. Multiple apoptotic signals, including serum withdrawal and treatment with a variety of pharmacological agents, activate caspase-3. The role of caspase-3 in apoptosis of oral cancer cells has not yet been reported. Lewis et al report that PKC inhibition induces DNA fragmentation in the colon cancer cell line, COLO 205 cells, which is blocked by cysteine protease inhibition but not mediated through caspase-3[17]. However, we have examined the caspase-3 content changes in TSCCa cells after staurosporine exposure for various periods of time, and have shown that staurosporine activates caspase-3 in a time-dependent manner. These results suggest that caspase-3 was activated by staurosporine in TSCCa cells, and might mediate staurosporine-induced TSCCa cells apoptosis.
Survivin is associated with the microtubules of the mitotic spindle. Disruption of the survivin-microtubule interaction leads to loss of survivin function and increased proapoptotic caspase-3 activity[18]. In the present study, the majority of ethanol-treated control TSCCa cells over-expressed survivin, but this was not enough to protect TSCCa cells from staurosporine-induced apoptosis. Further-more, a dose-related decrease of survivin content in TSCCa cells was associated with the induction of apoptosis by staurosporine; these results suggest that survivin in TSCCa cells could not protect TSCCa cells from the lethal effects of staurosporine.
In conclusion, the present study demonstrates that staurosporine induces apoptosis in TSCCa cells. Our results document, for the first time, the potential roles of the inhibition of PKC α in TSCCa cells’ apoptosis. This property of PKC α appears to be linked to the cleavage of survivin and activation of caspase-3. Previous studies have reported that PKC activation could cause stimulation of the transcription factor NF-κB, which in turn induced expression of the IAP[19–22]. As discussed in the present study, the IAPs are known to block caspase-3 activity and apoptosis. Therefore, it is possible that PKC α inhibition-mediated staurosporine causes reduction of survivin and results in the activation of the caspase-3 signaling pathway. Further study is needed to understand the role of other caspases in staurosporine-induced TSCCa cells apoptosis, which might be a potential target in the treatment of oral cancer diseases.
References
- Meier P, Finch A, Evan G. Apoptosis in development. Nature 2000;407:796-801.
- Thompson CB. Apoptosis in the pathogenesis and treatment of disease. Science 1995;267:1456-62.
- Newton AC. Protein kinase C: structure, function, and regulation. J Biol Chem 1995;270:28495-8.
- Newton AC. Protein kinase C: structural and spatial regulation by phosphorylation, cofactors, and macromolecular interactions. Chem Rev 2001;101:2353-64.
- Chae HJ, Kang JS, Byun JO, Han KS, Kim DU, Oh SM, et al. Molecular mechanism of staurosporine-induced apoptosis in osteoblasts. Pharmacol Res 2000;42:373-81.
- Adayev T, Ray I, Sondhi R, Sobocki T, Banerjee P. The G protein-coupled 5-HT1A receptor causes suppression of caspase-3 through MAPK and protein kinase Cα. Biochim Biophys Acta 2003;1640:85-96.
- Zhang B, Wu Q, Ye XF, Liu S, Lin XF, Chen MC. Roles of PLC-γ2 and PKCα in TPA-induced apoptosis of gastric cancer cells. World J Gastroenterol 2003;9:2413-8.
- Nicholson DW, Ali A, Thornberry NA, Vaillancourt JP, Ding CK, Gallant M, et al. Identification and inhibition of the ICE/CED-3 protease necessary for mammalian apoptosis. Nature 1995;376:37-43.
- Khan SM, Dauffenbach LM, Yeh J. Mitochondria and caspases in induced apoptosis in human luteinized granulosa cells. Biochem Biophys Res Commun 2000;269:542-5.
- Lo Muzio L, Pannone G, Staibano S, Mignogna MD, Rubini C, Mariggio MA, et al. Survivin expression in oral squamous cell carcinoma. Br J Cancer 2003;89:2244-8.
- Tanaka C, Uzawa K, Shibahara T, Yokoe H, Noma H, Tanzawa H. Expression of an inhibitor of apoptosis, survivin, in oral carcinogenesis. J Dent Res 2003;82:607-11.
- Shang ZJ, Li JR, Li ZB. Effect of exogenous nitric oxide on oral squamous cell carcinoma: an in vitro study. J Oral Maxillofac Surg 2002;60:905-10.
- Kern JC, Kehrer JP. Acrolein-induced cell death: a caspase-influenced decision between apoptosis and oncosis/necrosis. Chem Biol Interact 2002;139:79-95.
- Yang LC, Yang SH, Tai KW, Chou MY, Yang JJ. MEK inhibition enhances bleomycin A5-induced apoptosis in an oral cancer cell line: signaling mechanisms and therapeutic opportunities. J Oral Pathol Med 2004;33:37-45.
- Zhang BF, Peng FF, Zhang W, Shen H, Wu SB, Wu DC. Involvement of cyclin dependent kinase 5 and its activator p35 in staurosporine-induced apoptosis of cortical neurons. Acta Pharmacol Sin 2004;25:1105-11.
- Giuliano M, Bellavia G, Lauricella M, D’Anneo A, Vassallo B, Vento R, et al. Staurosporine-induced apoptosis in Chang liver cells is associated with down-regulation of Bcl-2 and Bcl-XL. Int J Mol Med 2004;13:565-71.
- Lewis AE, Wong BCY, Langman MJS, Eggo MC. Protein kinase C inhibition induces DNA fragmentation in COLO 205 cells which is blocked by cysteine protease inhibition but not mediated through caspase-3. Exp Cell Res 2003;289:1-10.
- Shin S, Sung BJ, Cho YS, Kim HJ, Ha NC, Hwang JI, et al. An antiapoptotic protein human survivin is a direct inhibitor of caspase-3 and -7. Biochemistry 2001;40:1117-23.
- Joshi SG, Francis CW, Silverman DJ, Sahni SK. NF-kappaB activation suppresses host cell apoptosis during Rickettsia rickettsii infection via regulatory effects on intracellular localization or levels of apoptogenic and anti-apoptotic proteins. FEMS Microbiol Lett 2004;234:333-41.
- Zou T, Rao JN, Guo X, Liu L, Zhang HM, Strauch ED, et al. NF-kappaB-mediated IAP expression induces resistance of intestinal epithelial cells to apoptosis after polyamine depletion. Am J Physiol Cell Physiol 2004;286:C1009-18.
- Luschen S, Scherer G, Ussat S, Ungefroren H, Adam-Klages S. Inhibition of p38 mitogen-activated protein kinase reduces TNF-induced activation of NF-kappaB, elicits caspase activity, and enhances cytotoxicity. Exp Cell Res 2004;293:196-206.
- Yang LQ, Fang DC, Wang RQ, Yang SM. Effect of NF-kappaB, survivin, bcl-2 and caspase 3 on apoptosis of gastric cancer cells induced by tumor necrosis factor related apoptosis inducing ligand. World J Gastroenterol 2004;10:22-5.