Effects of CpG-ODN on gene expression in formation of foam cells1
Introduction
CpG-oligodeoxynucleotides (CpG-ODN), the synthetic oligodeoxynucleotides containing unmethylated CpG dinucleotides in specific sequence contexts, are novel immune enhancers for systemic and mucosal immunization[1]. Animal studies suggest that CpG-ODN could be used as vaccine adjuvants, or antiallergenic, chemotherapeutic, or immunoprotective agents[2–5]. Although the immunological effects of CpG-ODN have been widely studied[6–9], including the mechanism by which CpG-ODN binds to the cell surface and the way in which its immunostimulatory activity is modulated by extracellular pH[10], its biological activities are not comprehensively understood. To improve our knowledge of the biological activities of CpG-ODN, we used a DNA microarray to profile the gene expression in an immune cell line after CpG-ODN treatment, and found that some genes related to the formation of macrophage foam cells were upregulated. We therefore reasoned that CpG-ODN might affect the lipid metabolism and the formation of foam cells. Foam cells are characteristic pathological cells in atherosclerotic lesion. An excess lipid loading in macrophages results in the formation of foam cells, and this is a key process in the early stages of atherosclerotic lesions[11]. Therefore, it is very important to investigate how CpG-ODN affects the formation of foam cells. In the present study, we investigated the effects of CpG-ODN on the gene expression of the related receptors or enzymes involved in atherosclerosis, and further confirmed the results by using semiquantitative RT-PCR. Furthermore, by histochemical and high performance liquid chromatography (HPLC) analysis, we demonstrated that CpG-ODN could affect the formation of foam cells.
Materials and methods
CpG-ODN ODN-1826 (5'-TCC ATG ACG TTC CTG ACG TT-3') and ODN-2006 (5'-TCG TCG TTT TGT CGT TTT GTC GTT-3') were synthesized by Sangon Biotechnology Co in Shanghai, China.
Cell culture The mouse macrophage line RAW264.7 and the human monocyte line U937 were obtained from the Cell Bank in the Shanghai Institutes of Biochemistry and Cell Biology, Chinese Academy of Sciences, and were cultured in RPMI-1640 medium (Gibco/BRL, Rockville, USA), supplemented with 10% fetal bovine serum, 2 mmol/L glutamine, and 50 mg/mL gentamycin in 5% CO2 at 37 oC. The culture medium was changed every 48 h.
Analysis of gene expression profile of immune cells by using a cDNA microarray The MGEC-80s microarray obtained from Biostar Genechip (Shanghai) was used. RAW264.7 cells were stimulated with ODN-1826 6 μmol/L for 6 h, and total RNA was isolated from cultured cells using TRIzol reagent (Gibco). mRNA was purified using the Oligotex mRNA midi Kit (Qiagen, Germany). The fluorescent cDNA probes were synthesized from total RNA by reverse transcription and then purified, referring to the protocol of Schena et al and Shalon D et al[12,13]. The probes from the control were labeled with Cy3-dUTP; those from the stimulated cells by ODN-1826 were labeled with Cy5-dUTP. The probes were mixed and precipitated with ethanol, and resolved in 20 µL hybridization solution [5×SSC+0.4% sodium dodecylsulfate (SDS)+50% formamide+5× Denhardt’s solution]. Chips were prehybridized with the hybridization solution+3 µL denatured salmon sperm DNA at 42 oC for 6 h. After denaturing at 95 oC for 5 min, the probe mixture was added to the prehybridized chip and covered with glass. Hybridization was performed at 60 oC for 16 h. After hybridization of a sample to the microarray, the sample was removed, and arrays were washed in solutions of 2×SSC + 0.2% SDS, 0.1% SSC + 0.2% SDS, and 0.1×SSC at 60 oC, respectively, for 10 min each, then dried at room temperature. Arrays were scanned with a laser scanner (ScanArray4000, General Scanning Inc, Watertown, Massachusetts) at 2 wavelengths. The acquired images were processed using a modification of GenePix Pro 3.0 software. The intensity of each spot at the 2 wavelengths represented the quantity of Cy3-dUTP and Cy5-dUTP, respectively. Each ratio of Cy3 to Cy5 was computed. The overall intensity was normalized by a coefficient according to the ratios of the 40 housekeeping genes that were also located.
Semiquantitative RT-PCR Total RNA of the U937 cells was extracted, purified using the Tissue/Cell Total RNA Isolation Kit (Watson, Shanghai), and quantified spectro- photometrically. A total of 200 ng RNA was reverse transcribed to cDNA in a volume of 20 µL using oligo(dT) primers and a commercially available kit (Reverse Transcription System, Promega, USA) according to the manufacturer’s instructions. For transcription, the RNA was denatured at 70 oC and then transcribed for 1 h at 42 oC. After inactivation of the avian myeloblastosis virus (AMV), (1 min at 99 oC), the transcript was cooled down to 4 oC. Four microliters of the reaction mixture was subjected to a final PCR reaction in a total volume of 20 µL, containing 0.5 µL of Taq polymerase (Takara Biotechnology, Dalian, China), 2 µL of 10×PCR Buffer (Mg2+ Plus, Takara, Dalian), 2 µL dNTP mix (2.5 mmol/L), 0.5 µL of each of the 5' and 3' primers, and 10.5 µL of H2O. All gene-specific PCR primers were designed based on the DNA sequences in GenBank (National Center for Biotechnology Information, Bethesda, MD, USA). The sequence of the primers and the annealing temperatures are shown in Table 1. The amplification was carried out over 35 cycles of the following: denaturation, 1 min at 94 oC; annealing, 30 s, at temperature see Table 1; elongation, 1 min at 72 oC. PCR products were separated by agarose gel electrophoresis. β-actin was used as a control. The intensities of the gene-specific PCR products were quantified from scanned images using the AlphaEase FC software (Alpha).
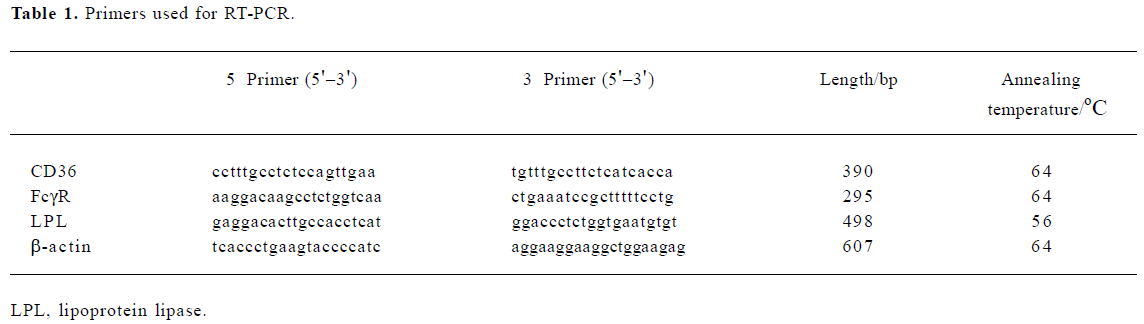
Full table
Preparation of ox-LDL Low-density lipoprotein (LDL) (d=1.019 to 1.063 kg/L; Sigma) was sterilized by filtration through 0.45 µm Millipore membranes, and stored at 4 oC as described previously[14]. After edetic acid was removed by dialysis, LDL were oxidized by incubation in CuSO4 10 µmol/L at 37 oC for 12 h, and then dialyzed in phosphate buffered saline (PBS) containing edetic acid 0.1 mmol/L at 4 oC for 24 h.
In vitro induction of foam cell formation Confluent U937 cells were pretreated in serum-free RPMI-1640 for 24 h and then incubated with ox-LDL 80 mg/L for 48 h. The foam cell model was thus established. In the experiments, U937 cells were cultured with ODN-2006 6 µmol/L and ox-LDL 80 mg/L for 48 h, which were added to the medium simultaneously. The control group was the U937 cells without ODN-2006 treatment. The morphological form of the foam cells was analyzed by histochemical methods.
Histochemical analysis of foam cells The foam cells were collected and fixed with 4% paraformaldehyde for 12 h on the slides as described previously[15]. The slides were rinsed in water and placed in fresh 0.3% oil red O (Amresco, Solon, USA) solution for 20 min. The slides were rinsed in 50% isopropyl alcohol and examined.
Lipid extraction Lipids were extracted by using the method of Hara and Radin[16] with modifications. The cells were collected from the culture flasks into 0.9% NaCl (2 mL per 75 cm2 flask) and homogenized on ice by sonication for 10 s with a Sonifier 450 sonicator (Branson Ultrasonics, Danbury, USA) set to maximum power. The protein concentration of the cell lysate was determined by the method of Lowry et al[17]. To a volume of cell suspension known to contain 1 mg of protein was added 100 µg of cholesteryl heptadecanoate in chloroform as an internal standard. An equal volume of freshly prepared cold (-20 oC) KOH in ethanol (150 g/L) was then added and the cell lysate was repeatedly vortexed until clear with 6% trichloroacetic acid. An equal volume of 4:1 hexane-isopropanol (v/v) was added and the mixture was vortexed for 5 min followed by centrifugation at 800×g and 15 oC for a further 5 min. The extraction procedure was repeated twice (a total of three extraction procedures). The combined organic phase was transferred to clean tapered glass tubes and thoroughly dried in a vacuum freeze dryer at 65 oC. The tubes were allowed to cool to room temperature, 100 µL of the mixture of isopropyl alcohol-n-heptane-acetonitrile at 35:12:52 (v:v:v) was added, and the sample was solubilized by placing it in an ultrasound water bath for 5 min at room temperature. After centrifugation at 800×g for 5 min, 20 µL of the sample was introduced into the HPLC device.
Determination of cholesterol and cholesteryl ester content of U937 cells by HPLC The cholesterol and cholesteryl ester content of cells were analyzed by HPLC as described previously[18]. HPLC was performed using a Waters device (Milford, USA) equipped with a model 1525 binary pump, a model 717 plus autosampler, a model 2487 dual λ absorbance detector, and a 4.6 mm×100 mm Gen-Pak FAX column (Waters). Waters’ Breeze software was used to control the HPLC system. Cholesterol and cholesteryl esters were eluted isocratically at a flow rate of 0.5 mL/min and at a temperature of 4 oC using an eluent consisting of isopropanol-n-heptane-acetonitrile at the ratio of 35:12:52 (v:v:v) and detected by ultraviolet absorption at 206 nm.
Statistics Every experiment was repeated at least 3 times. All values are expressed as mean±SD. Differences were considered statistically significant when P<0.05 as determined by the paired t-test.
Results
Gene expression profile in immune cells By using the cDNA microarray, 119 genes related to cell cycle, immune modulation, and signal transduction were found to be differentially expressed, of which 3 genes related to the formation of macrophage foam cells had increased expression after ODN-1826 6 μmol/L stimulation (Table 2).
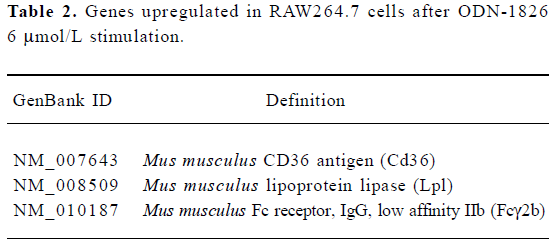
Full table
Confirmation of foam cell-related gene expression by RT-PCR According to the 3 genes in Table 2, we designed primers and performed semiquantitative RT-PCR. The expression of these genes in the ODN-2006 treated group was markedly increased, compared with the control group (Figure 1).
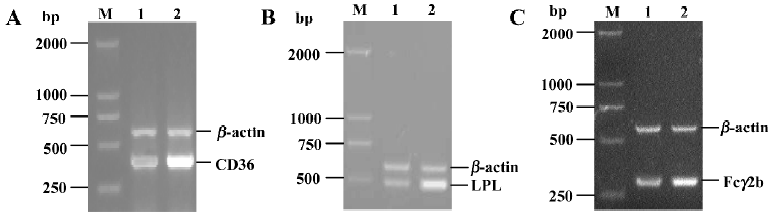
Effect of CpG-ODN on lipid deposition in cells Lipid drops inside the foam cells were stained with oil red O, and some of the red pellets were found in the plasma of the U937 cells after being incubated with ox-LDL, indicating that the foam cell model was formed. After ODN-2006 6 μmol/L was added to the medium, lipid deposition in U937 foam cells was enhanced (Figure 2).
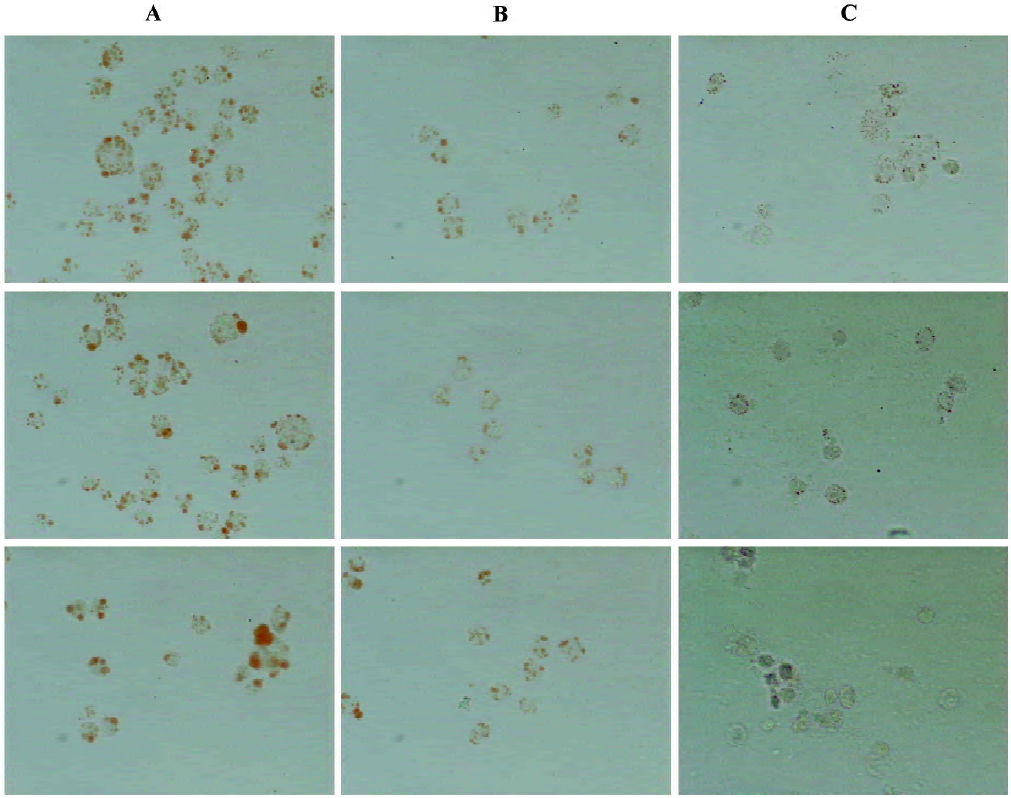
Effect of CpG-ODN on cholesterol and cholesteryl ester in foam cells After incubation with ODN-2006 6 μmol/L and ox-LDL 80 mg/L for 48 h, the level of cholesteryl ester in foam cells increased markedly compared with the controls (Figure 3). This suggests that CpG-ODN can also increase lipid accumulation (based on the assay of the concentrations of cholesteryl ester and the ratio of cholesteryl ester to total cholesterol; Table 3). Cholesterol concentration has a strong linear relationship with its peak area within 0.05–1.0 mg/L (r2 in each case was >0.998).
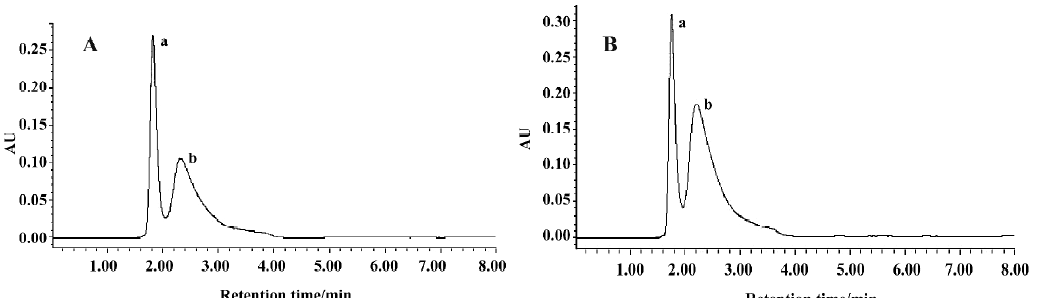

Full table
Discussion
Previous reports have suggested that CpG-ODN is mainly involved in immunomodulation[19,20]. Nevertheless, in the study presented here, we investigated immune cells treated with CpG-ODN using cDNA microarray technology and, surprisingly, found that the expression of 3 genes (CD36 [NM_007643], LPL [NM_008509], and Fcγ2b [NM_010187]) increased markedly. These results were further confirmed by semiquantitative RT-PCR. CD36, LPL, and Fcγ2b are thought to be closely related to lipid metabolism and the formation of macrophage foam cells, therefore CpG-ODN is able to modulate gene expression in foam cells, and increase lipid accumulation in foam cells. Our findings suggest that CpG-ODN has a novel biological activity.
Atherosclerosis, and the resulting coronary heart disease and cerebral stroke, is the most common cause of death in industrialized nations. Mammalian cells have evolved complex feedback mechanisms to ensure a sufficient supply of cholesterol and to prevent its excessive accumulation. During the process of atherosclerosis, these homeostatic mechanisms fail in macrophages. Uncontrolled cholesterol deposition is promoted by scavenger functions of the macrophages and the adaptive mechanisms elicited are not sufficient to process the lipid load. Consequently, a lipid-laden “foam cell” is formed. The active stages of atherosclerotic lesions are characterized by the extensive infiltration of blood-derived monocyte/macrophages through the endothelium into the arterial intima. The monocytes migrate into the arterial intima where they internalize ox-LDL through scavenger receptors and become macrophage-derived foam cells results in the formation of fatty streaks, which are believed to represent the earliest type of atherosclerotic plaque. CpG-ODN can upregulate the related gene expression in foam cells, which implies that CpG-ODN might function as a facilitative atherosclerotic factor. This suggests that the clinical application of CpG-ODN might be risky in cases of atherosclerosis.
Macrophage-derived foam cells in atherosclerotic lesions are generally thought to play a major role in the pathology of the disease. The uptake of ox-LDL by macrophages is a key event implicated in the initiation and development of atherosclerotic lesions. Two macrophage surface receptors, CD36 (a class B scavenger receptor) and the macrophage scavenger receptor (a class A scavenger receptor), have been identified as the major receptors that bind and internalize ox-LDL. CD36 is an 88 kDa transmembrane glycoprotein that is expressed on monocytes, platelets, and microvascular endothelium. The expression of CD36 in monocytes/macrophages in tissue culture is dependent both on their differentiation state and exposure to soluble mediators (cytokines and growth factors). Modified lipoproteins such as LDL immune complexes can also enter the macrophage through the Fcγ receptor, which is a classic inhibitory receptor that is widely expressed on B cells, macrophages, neutrophils, and mast cells. Once LDL immune complexes combine with the Fcγ receptor, it may enhance LDL oxidation and expression of the scavenger receptor[21]. Lipoprotein lipase (LPL) is the enzyme primarily responsible for the hydrolysis of core triglycerides in circulating chylomicrons and very low density lipoproteins (VLDL) to generate cholesterol-enriched remnants[22]. LPL is synthesized by many cell types, including vascular smooth muscle cells (VSMC) and macrophages, and associates with the vascular endothelium. Increased LPL activity in the arterial wall correlates with increased areas of lipid deposition and increased atherosclerotic lesion formation[23]. The activity of LPL in promoting atherosclerosis may depend on its dual role as an enzyme and as a bridging protein that increases the binding of lipoproteins to the cell surface[22].
Our data suggest that CpG-ODN upregulates the expression of CD36, Fcγ2b, and LPL in foam cells, which indicates that CpG-ODN might have some influence on the formation of foam cells and related gene expression.
References
- McCluskie MJ, Davis HL. CpG DNA is a potent enhancer of systemic and mucosal immune responses against hepatitis B surface antigen with intranasal administration to mice. J Immunol 1998;161:4463-6.
- Sommer F, Wilken H, Faller G, Lohoff M. Systemic Th1 immunization of mice against Helicobacter pylori infection with CpG oligodeoxynucleotides as adjuvants does not protect from infection but enhances gastritis. Infect Immun 2004;72:1029-35.
- Weigel BJ, Rodeberg DA, Krieg AM, Blazar BR. CpG oligo- deoxynucleotides potentiate the antitumor effects of chemotherapy or tumor resection in an orthotopic murine model of rhabdomyosarcoma. Clin Cancer Res 2003;9:3105-14.
- Jorgensen JB, Johansen LH, Steiro K, Johansen A. CpG DNA induces protective antiviral immune responses in Atlantic salmon ( L). J Virol 2003; 77: 11 471–9.
- Sur S, Wild JS, Choudhury BK, Sur N, Alam R, Klinman DM. Long term prevention of allergic lung inflammation in a mouse model of asthma by CpG oligodeoxynucleotides. J Immunol 1999;162:6284-93.
- Yi AK, Klinman DM, Martin TL, Matson S, Krieg AM. Rapid immune activation by CpG motifs in bacterial DNA. Systemic induction of IL-6 transcription through an antioxidant-sensitive pathway. J Immunol 1996;157:5394-402.
- Ballas ZK, Rasmussen WL, Krieg AM. Induction of NK activity in murine and human cells by CpG motifs in oligodeoxynucleotides and bacterial DNA. J Immunol 1996;157:1840-5.
- Verthelyi D, Ishii KJ, Gursel M, Takeshita F, Klinman DM. Human peripheral blood cells differentially recognize and respond to two distinct CpG motifs. J Immunol 2001;166:2372-7.
- Sparwasser T, Koch ES, Vabulas RM, Heeg K, Lipford GB, Ellwart JW, et al. Bacterial DNA and immunostimulatory CpG oligonucleotides trigger maturation and activation of murine dendritic cells. Eur J Immunol 1998;28:2045-54.
- Hu ZL, Sun SH, Zhou FJ. The binding of CpG-oligodeo- xynucleotides to cell-surface and its immunostimulatory activity are modulated by extracellular acidic pH. Vaccine 2003;21:485-90.
- Vainio S, Ikonen E. Macrophage cholesterol transport: a critical player in foam cell formation. Ann Med 2003;35:146-55.
- Schena M, Salon D, Heller R. Parallel human genome analysis: microarray-based expression monitoring of 1000 genes. Proc Natl Acad Sci USA 1996; 93: 10 614–9.
- Shalon D, Smith SJ, Brown PO. A DNA microarray system for analyzing complex DNA samples using two-color fluorescent probe hybridization. Genome Res 1996;6:639-45.
- Kuzuya M, Naito M, Funaki C, Hayashi T, Asai K, Kuzuya F. Lipid peroxide and transition metals are required for the toxicity of oxidized low density lipoprotein to cultured endothelial cells. Biochim Biophys Acta 1991;1096:155-61.
- Yang PY, Rui YC, Li K, Huang XH, Jiang JM, Yu L. Expression of intercellular adhesion molecule-1 in U937 foam cells and inhibitory effect of imperatorin. Acta Pharmacol Sin 2002;23:327-30.
- Hara A, Radin NS. Lipid extraction of tissues with a low-toxicity solvent. Anal Biochem 1978;90:420-6.
- Lowry OH, Rosebrough NJ, Farr AL, Randall RJ. Protein measurement with the Folin phenol reagent. J Biol Chem 1951;193:265-75.
- Wang Z, Liu YL, Jiang ZS. A new method to measure the level of L-arginine of aortic wall in rabbits. Chin J Arterioscler 1996;4:72-4.
- Klinman DM, Yi AK, Beaucage SL, Conover J, Krieg AM. CpG motifs present in bacteria DNA rapidly induce lymphocytes to secrete interleukin 6, interleukin 12, and interferon gamma. Proc Natl Acad Sci USA 1996;93:2879-83.
- Horner AA, Raz E. Immunostimulatory sequence oligodeox- ynucleotide-based vaccination and immunomodulation: two unique but complementary strategies for the treatment of allergic diseases. J Allergy Clin Immunol 2002;110:706-12.
- Kiener PA, Rankin BM, Davis PM, Yocum SA, Warr GA, Grove RI. Immune complexes of LDL induce atherogenic responses in human monocytic cells. Arterioscler Thromb Vasc Biol 1995;15:990-9.
- Goldberg IJ. Lipoprotein lipase and lipolysis: central roles in lipoprotein metabolism and atherogenesis. J Lipid Res 1996;37:693-707.
- Stein Y, Stein O. Lipoprotein lipase and atherosclerosis. Atherosclerosis 2003;170:1-9.