A novel artemisinin derivative, 3-(12-β-artemisininoxy) phenoxyl succinic acid (SM735), mediates immunosuppressive effects in vitro and in vivo1
Introduction
Artemisinin is extracted from the herb Artemisia annua L, and various forms of the drug are used as anti-malarial agents[1,2]. Artemisinin is a potent anti-malarial agent with low toxicity, and its derivatives have improved efficacy in malaria treatment relative to artemisinin itself. In addition to anti-malarial effects, anti-lymphocytic effects have also been reported for artemisinin derivatives[3,4]. Since the 1980s, the immunosuppressive actions of artemisinin and its derivatives have been studied in China. Artemisinin derivatives have also been tested for the treatment of dermatoses such as photoallergic skin diseases and systemic lupus erythema-tosus, and promising results have been reported[5–7].
Researchers have demonstrated that the anti-malarial activity of artemisinin and its derivatives is associated with their endoperoxides[8,9]. When catalyzed by the ferrous ion in heme, cleavage of peroxy bonds produces free radicals and thus causes damage to malarial DNA and proteins. However, the underlying mechanism of the anti-lymphocytic action of artemisinin and its derivatives is poorly understood.
Despite their anti-lymphocytic mechanisms being poorly understood, we hypothesized that artemisinin derivatives might be promising immunosuppressive agents, because some clinical studies have shown that they are effective in the treatment of immune dysfunction diseases. When considering an optimal molecular structure (higher efficacy and lower toxicity) for artemisinin derivatives for use as immuno-suppressants, we noticed that nonsteroidal anti-inflammatory drugs (NSAIDs) remained the first-line therapy for most people with arthritis and other inflammatory diseases. Compared with other anti-inflammatory or immunosuppressive drugs such as steroids and cyclosporine, the molecular structures of NSAIDs are relatively simple and easy to synthesize. In addition, NSAIDs have few side effects, and a low level of toxicity, which are clinically desirable features. Given the relevance of the structure of NSAIDs to their immunosuppressive activity, we became interested in combining artemisinin and NSAIDs to develop a new class of immunosuppressive agents. Therefore, we synthesized and studied more than 100 different artemisinin derivatives. As a result of this screening program and subsequent medicinal chemistry studies, we obtained some derivatives that have significant immunosuppressive activity. Previous studies indicated that SM735 ([3-(12-β-artemisininoxy)]phenoxyl succinic acid, C25H32O9·1/2H2O, MW: 485.5, Figure 1) is a representative compound, with markedly lower toxicity and higher immunosuppressive activity than artemisinin[10]. Here, we further investigate its immunological characteristics both in vitro and in vivo.
The discovery of cyclosporine (cyclosporin A; CsA) and its successful utilization in organ transplantation was a milestone in clinical transplantation. CsA has produced a new era in transplantation in terms of both efficiency and quality of life for patients. In addition, research into the mechanisms by which CsA acts has been rewarding in that we now have a better understanding of the mechanisms leading to T lymphocyte activation[11,12]. Recently, Noori et al reported that the immunosuppressive activity of artemisinin was even greater than that of CsA, as indicated by both in vivo and in vitro studies[13]. Therefore, in the present study, we demonstrate that SM735 has strong immunosuppressive effects in vitro and in vivo, and explore its possible mechanism of action.
Materials and methods
Reagents Concanavalin A, lipopolysaccharide (Escherichia coli 055:B5), ionomycin, phorbol 12-myristate 13-acetate (PMA), 3-[4,5-dimethylthylthiazol-2-yl]-2,5-diphenyl-tetrazolium bromide (MTT), and 3,3',5,5'-tetramethyl-benzidine were purchased from Sigma (St Louis, MO, USA). Mitomycin-C was purchased from Kyowa Hakko (Tokyo, Japan). RPMI-1640 and fetal bovine serum (FBS) was purchased from Gibco (Life Technology, NY, USA). 1-Fluoro-2,4-dinitrobenzene (DNFB) was purchased from Merck (Hong Kong, China). Mouse enzyme-linked immunosorbent assay (ELISA) kits of interleukin (IL)-2, IL-12p40, interferon (IFN)-γ and IL-6 were purchased from Pharmingen (San Diego, CA, USA).
Cyclosporine (Sandimmun) was purchased from Novartis Pharma AG, Switzerland. Cyclophosphamide was purchased from Hualian Pharma (Shanghai, China). SM735 [3-(12-β-artemisininoxy)phenoxyl succinic acid] was synthesized by us. Before use, SM735 was dissolved in pure dimethyl sulfoxide (Me2SO; 100 g/L) as a stock solution, and stored at 4 oC. The stock solution was diluted to the needed concentrations with RPMI-1640 supplemented with 10% FBS. The final concentration of Me2SO in the culture medium was less than 0.01%, which had no influence on the assays[10,14]. For in vivo experiments, both SM735 and the reference drugs were dissolved in 0.5% Tween-80, 0.33% Me2SO in saline.
Animals and housing conditions Inbred 7−9-week-old BALB/c, C57BL/6 mice were obtained from the Shanghai Experimental Animal Center of the Chinese Academy of Sciences (Certificate N
Preparation of splenocytes Splenocytes were prepared aseptically from inbred 7−9-week-old BALB/c, C57BL/6 mice, and cultured in RPMI-1640 supplemented with 10% endotoxin-free, heat-inactivated FBS, 100 kU/L penicillin, 100 mg/L streptomycin, 10 mmol/L N-2-hydroxyethylpiperazine-N'-2-ethanesulfonic acid (HEPES), and 50 µmol/L 2-mercapto-ethanol (2-ME).
Lymphocyte proliferation and cytotoxicity assay Lymphocyte proliferation was carried out as described elsewhere[15]. Briefly, 7–8-week-old male BALB/c mice were killed and splenocytes were prepared aseptically to a single-cell suspension. Splenic lymphocytes were stimulated with ConA (5 mg/L) or lipopolysaccharide (LPS 10 mg/L), plus the required concentrations of drugs. The cell cultures were then incubated for 48 h at 37 oC in a humidified 5% CO2 incubator. Cells were pulsed with 0.5 µCi/well [3H]-thymidine 8 h prior to the end of the culture. After 48 h, cells were harvested onto a glass fiber filter using a HARVESTER96® (TOMTEC, USA) 96-well cell harvester and incorporated radioactivity was counted with a Beta Scintillation counter (1450 MicroBeta Trilux, PerkinElmer Life Sciences, Boston, MA, USA).
Cytotoxicity was assessed by using the MTT assay. Briefly, 15 µL of 5 g/L MTT was pulsed 4 h prior to the end of the culture (in a total volume of 160 µL), and then 80 µL solvent [10% sodium dodecylsulfate (SDS), 50% N,N-dimethyl formamide, pH 7.2] was added to dissolve the precipitate. The solution was incubated for another 7 h and OD570 nm was read by using a microplate reader (Bio-Rad, Model 550, Tokyo, Japan).
Mixed lymphocyte reaction proliferation assay The mixed lymphocyte reaction (MLR) was carried out as previously described, with some modifications[16]. Briefly, spleen cells from 7–9-week-old BALB/c mice were prepared in a 1×1010 cells/L suspension, which was inactivated for 2 h with 50 mg/L of mitomycin C. Cells were washed and co-cultured with freshly prepared splenocytes from C57/BL6 mice (the ratio of stimulator to responder was 1.0) in a final concentration of 1.5×109 cells/L for 96 h. The desired concentrations of each compound were added in cultures for immunological activity assays. [3H]Thymidine (0.5 µCi/well) was pulsed 24 h prior to the end of the culture and then cells were harvested onto a glass fiber filter for measurement of incorporated radioactivity.
Cytokine production and analysis Splenocytes (5×106) were prepared from 7–9-week-old BALB/c mice, added to 1 mL of RPMI-1640 media, and then were incubated with 5 mg/L of ConA, 10 mg/L of LPS, or 10 µg/L of PMA plus 1 µmol/L of ionomycin, for 24 h of culture in 48-well microculture plates. The supernatants were harvested and stored at –80 oC before the assay was carried out. Cytokine levels in supernatants were measured by ELISA, according to the manufacturer’s instructions (Pharmingen, San Diego, CA, USA). 3,3',5,5'-Tetramethylbenzidine was used to develop the color reaction. The absorbance was read at 450 nm by a microplate reader (Bio-Rad, model 550, Tokyo, Japan). Cytokine concentrations were calculated based on a standard curve created using standard murine cytokines.
DNFB-induced delayed-type hypersensitivity response Female BALB/c mice were randomized into 6 groups and sensitized with 20 µL of 0.5% DNFB dissolved in acetone-olive oil (4:1) on each hind foot on d 0 and d 1. Me2SO vehicle, CsA and SM735 were administered to each group (n=10) by intraperitoneal injection on 4 consecutive days (d 7–d 10). On d 9 mice were challenged with 10 µL of 0.2% DNFB on both sides of the right ear, using the method described by Phanuphak et al, with some modifications[17]. The extent of ear swelling was expressed as the difference between the weight of punches taken from the left and right ears by using an 8-mm punch 48 h after the second challenge.
Quantitative hemolysis of sheep red blood cells Female BALB/c mice were immunized by ip injection with 0.2 mL of 16.7% sheep red blood cells (SRBC) on d 0. Me2SO vehicle, 25 mg/kg of cyclophosphamide and SM735 were administered to each group (n=6) by ip injection on 4 consecutive days (d 1–d 4). On d 5, mice were killed, and mixed suspensions of 2×109 spleen cells/L were made. A total of 1 mL of cell suspension was incubated with 1 mL of 0.5% SRBC and 1 mL of 1:10 dilution of guinea pig complement for 0.5 h at 37 oC. The suspension was then centrifuged (3 min at 3000×g) and the extent of hemolysis in the supernatant was determined at 520 nm, according to the method used by Simpson and Gozzo with some modifications[18].
Statistical analysis Three independent experiments were performed with similar results. We counted the 50% cytotoxic concentration (CC50) and the 50% inhibitory concentration (IC50) values using the Origin software package (Microcal Software). Student’s t-test and one-way analysis of variance (ANOVA) with Newman-Keuls multiple comparisons on post-tests were used to analyze data and compare groups. P<0.05 was considered significant.
Results
Cytotoxicity of SM735 for murine splenocytes To determine the safe dose range of SM735, we first examined the cytotoxic effect of the compound. As Figure 2A shows, in a 48-h culture, SM735 had a typical S-shaped concentration-toxicity relationship for murine splenocytes. SM735 at a dose of 25 µmol/L showed no cytotoxicity (inhibitive rate <10%, P>0.05). The 50% cytotoxic concentration (CC50) value of SM735 was 53.1±7.8 µmol/L for a 48-h culture.
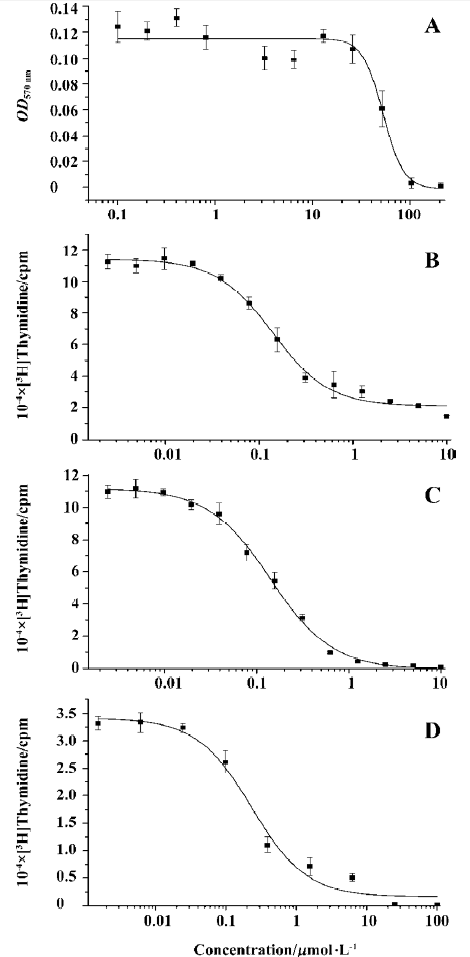
SM735 inhibits mitogen-induced murine splenocyte proliferation Concentration-dependent suppression of murine splenocyte proliferation in response to ConA and LPS was observed when SM735 was added to cell cultures. Figure 2B, 2C shows that proliferation induced by ConA or LPS was significantly inhibited by SM735 exposure. Both concentration-dependencies fitted typical S-shaped curves. Comparisons with a vehicle control showed that this effect was statistically significant (P<0.05) when concentrations of SM735 were above 0.04 µmol/L in response to ConA and above 0.02 µmol/L in response to LPS. In response to ConA, the IC50 value of SM735 inhibition of lymphocyte proliferation was 0.33±0.06 µmol/L, and in response to LPS, the IC50 value was 0.27±0.02 µmol/L.
SM735 inhibits splenocyte proliferation in MLR Mitomycin C-inactivated splenocytes from BALB/c mice (H-2d) were applied as allogeneic stimuli to proliferating splenocytes of C57BL/6 mice (H-2b). As shown in Figure 2D, SM735 strongly suppressed T cell proliferation in MLR in a dose-dependent manner, with an IC50 value of 0.86±0.18 µmol/L for a 96-h co-culture. When concentrations of SM735 were above 0.08 µmol/L, comparisons with the vehicle control showed that this effect was statistically significant (P<0.05). The IC50 values were comparable to those when ConA-stimulated T cell proliferation was suppressed.
Effects of SM735 on cytokine production We used ConA alone, LPS alone, or PMA plus ionomycin as stimuli to promote cytokine secretion in mouse splenocytes. Then the effects of SM735 on the production of proinflammatory cytokine IL-6, and Th1-type cytokines IL-2, IL-12, and IFN-γ, were examined. The results are summarized in Table 1. The production of IL-12, IFN-γ, and IL-6 was significantly decreased in a concentration-dependent manner when the splenocyte cultures were exposed to SM735, upon stimulation with LPS or PMA plus ionomycin. We also detected a strong inhibitive effect of SM735 on IFN-γ production induced by ConA. Unexpectedly but interestingly, we did not observe a significant effect of SM735 on IL-2 production.
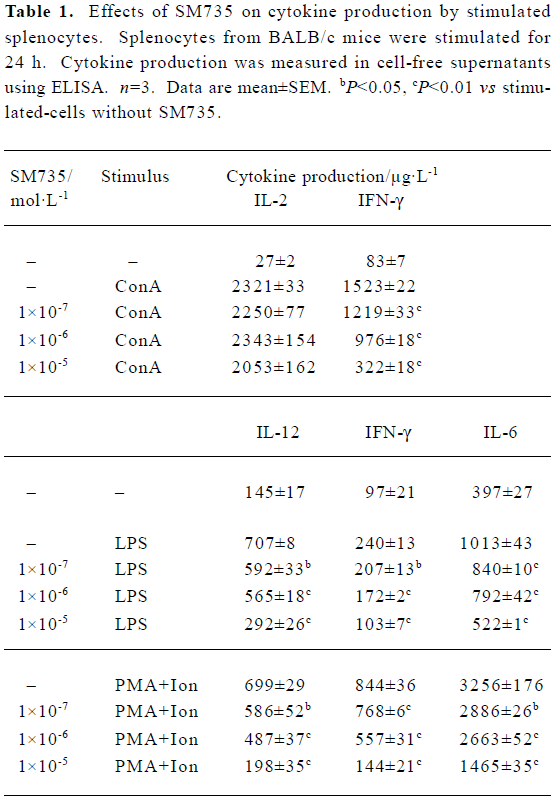
Full table
SM735 suppresses DNFB-induced delayed-type hypersensitivity reaction Figure 3 illustrates the dose-dependent inhibitory effect of SM735 on DNFB-induced DTH ear swelling. When SM735 was administered for 4 consecutive days, at doses of 7.5, 15, and 30 mg/kg, SM735 significantly suppressed ear swelling by 19.6%, 35.5%, and 55.4%, respec-tively. The inhibition was comparable to that of CsA, which exerted 55.2% suppression at a dose of 50 mg/kg.
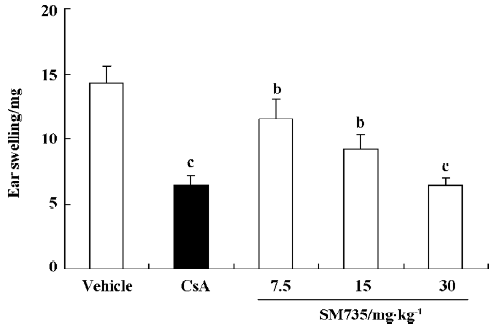
SM735 suppresses anti-SRBC specific immunoglobulin production Quantitative hemolysis of SRBC (QHS) is a model of primary antibody production in response to antigenic stimulation. Because CsA specifically blocks T cell activation by suppressing IL-2 production, with little effect on B cells, we chose cyclophosphamide (CTX) as a reference drug in the present study[19]. As Figure 4 shows, administration of 15 and 30 mg/kg SM735 for 4 consecutive days significantly suppressed QHS in a dose-dependent manner. The inhibitive rates were 15.3% (P<0.05) and 25.4% (P<0.01), respectively, slightly less potent than the 31.8% inhibition effected by CTX (P<0.01).
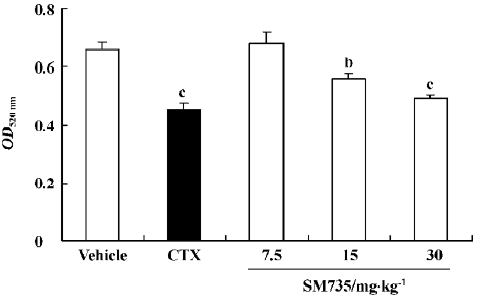
Discussion
Patients who receive organ grafts are principally treated with immunosuppressive agents. In addition, there are a number of other autoimmune chronic inflammatory disorders that might be able to be treated with immunosuppressive drugs. In the 1970s and 1980s, the discovery and clinical use of CsA and tacrolimus (FK506) enabled the successful transplantation of major organs in human patients. However, it seems now that neither are able to be tolerated in the long term[20,21]. These drugs cause systemic immunosuppression that greatly increases the risks of tumors arising and lethal fungal infections occurring. Therefore, new immunosuppressants are required that possess better therapeutic effects or can be combined with currently used drugs to reduce long-term tolerance and side effects.
Previous studies have indicated that artemisinin possesses anti-lymphocytic activities. There are numerous reports of clinical cases in which autoimmune diseases have been treated with artemisinin and artesunate; therapeutic effects were achieved in these cases, thus encouraging us to study artemisinin and its derivatives. To improve the efficacy of the drug, we optimized artemisinin by linking it with various NSAID structures. Here, we report that a newly synthesized artemisinin derivative, SM735, possesses potent immunosuppressive activity both in vitro and in vivo.
Lymphocyte proliferation is a crucial event in the activation cascade of both cellular and humoral immune responses. Treatment with SM735 can dose-dependently reduce both the T and B cell proliferation promoted by mitogens. Because it has a relatively high CC50 value, SM735 has a favorably large safety range.
A mixed lymphocyte reaction is induced by allogeneic stimuli, and T lymphocytes are the main responders, indicating an immune response very similar to that which occurs in post-transplantation graft-versus-host diseases[22,23]. MLR is often used clinically for tissue typing to identify the compatibility of donor organs and recipients. Furthermore, the suppression of MLR by immunosuppressants also helps improve the success of transplantation[24,25]. Here we show that SM735 also potently inhibited MLR, with an IC50 value comparable to that for the inhibition of T cell proliferation. At concentrations above 10 µmol/L, SM735 almost completely abrogated MLR. This suggests that SM735 would probably act as an immunosuppressant in physiological conditions.
Cytokines are important modulators and effectors in the immune system. In particular, multiple proinflammatory cytokines have been proved to be closely associated with many autoimmune diseases. It is critical to silence proinflam-matory cytokines to maintain successful immunosuppression[26–28]. In the present study, we used mitogen ConA, bacterial LPS, and PMA plus ionomycin, which agitated the whole cell population by activating protein kinase C, to promote cytokine production in splenocytes. The results showed that SM735 significantly inhibited IL-12, IFN-γ and IL-6 production with LPS or PMA plus ionomycin stimulation. When using ConA as a stimulant, we also observed a dose-dependent inhibition of IFN-γ production. Unexpectedly, the IL-2 level was not obviously altered. Although the two cornerstone immunosuppressants, CsA and FK506, are putatively regarded as IL-2 inhibitors, it seems that SM735 acts totally differently, on some event(s) downstream of IL-2-mediated naive T cell activation. IL-2 is widely considered to be a key cytokine in T-cell-dependent immune responses. However, the main non-redundant activity of this cytokine centers on the regulation of T cell tolerance, and recent studies have indicated that a failure in the production of CD4+CD25+ regulatory T cells is the underlying cause of autoimmunity in the absence of IL-2[29]. Thus, the fact that SM735 does not inhibit IL-2 indicates that further research is necessary on the induction of long-term immune tolerance by SM735.
To examine the immunoregulatory effects of SM735 in vivo, we used a mouse DTH model and the QHS model. Ear swelling in DTH is primarily the result of antigen-specific CD4+ T cell activation[30]. Administration of SM735 for 4 consecutive days significantly suppressed ear swelling, indicating that SM735 is capable of inhibiting the T-cell-dependent immune response in vivo. In our experiments, the effect of SM735 was comparable to that of cyclosporine at a curative dose. The QHS model reflects the antibody-producing capacity of plasma cells in response to SRBC[18,19]. The suppressive effect of SM735 in QHS indicated that it also suppressed antibody-secreting B cells in vivo.
In conclusion, our results demonstrate that SM735, a newly synthesized artemisinin derivative, has strong immunosuppressive effects in vitro and in vivo. Because artemi-sinin and its derivatives potentially have low toxicity and few side effects[4,31], our study suggests the possibility of developing SM735 and other artemisinin derivatives as novel safe immunosuppressants, probably with a different mechanism from that of CsA or FK506. However, further studies are necessary to elucidate the details of the mechanism.
References
- Luo XD, Shen CC. The chemistry, pharmacology, and clinical applications of qinghaosu (artemisinin) and its derivatives. Med Res Rev 1987;7:29-52.
- Klayman DL. Qinghaosu (artemisinin): an antimalarial drug from China. Science 1985;228:1049-55.
- Li Y, Wu YL. An over four millennium story behind qinghaosu (artemisinin): a fantastic antimalarial drug from a traditional Chinese herb. Curr Med Chem 2003;10:2197-230.
- Tawfik AF, Bishop SJ, Ayalp A, el-Feraly FS. Effects of artemisinin, dihydroartemisinin and arteether on immune responses of normal mice. Int J Immunopharmacol 1990;12:385-9.
- Yu Q, Jin H. Artesunate in treatment of dermatosis. Bengbu Yixueyuan Xuebao 1997;22:309-10. Chinese..
- Yu Q, Gao Y. Systemic lupus erythematosus treated with artesunate. Chin J Dermatol 1997;30:51-2. Chinese..
- Zhang J, Zhong J, Shi Z, Dai X. Effects of lingdan and artesunate on T cell populations in human SLE. Chin Interg Tradit West Med 2002;7:489. Chinese..
- Meshnick SR, Taylor TE, Kamchonwongpaisan S. Artemisinin and the antimalarial endoperoxides: from herbal remedy to targeted chemotherapy. Microbiol Rev 1996;60:301-15.
- Yang YZ, Little B, Meshnick SR. Alkylation of proteins by artemisinin. Effects of heme, pH, and drug structure. Biochem Pharmacol 1994;48:569-73.
- Yang ZS, Zhou WL, Sui Y, Wang JX, Wu JM, Zhou Y, et al. Synthesis and immunosuppressive activity of new artemisinin derivatives. Part I. [12(β or α)-dihydroartemisininoxy] phen(ox)yl aliphatic acids/esters. J Med Chem 2005;48:4608-17.
- Allison AC. Immunosuppressive drugs: the first 50 years and a glance forward. Immunopharmacology 2000;47:63-83.
- Liu J. FK506 and cyclosporin, molecular probes for studying intracellular signal transduction. Immunol Today 1993;14:290-5.
- Noori S, Naderi GA, Hassan ZM, Bathaie SZ, Hashemi SM. Immunosuppressive activity of a molecule isolated from Artemisia annua on DTH responses compared with cyclosporin A. Int Immunopharmacol 2004;4:1301-6.
- Rowland TL, McHugh SM, Deighton J, Dearman RJ, Ewan PW, Kimber I. Differential regulation by thalidomide and dexamethasone of cytokine expression in human peripheral blood mononuclear cells. Immunopharmacology 1998;40:11-20.
- Feng YH, Zhou WL, Wu QL, Li XY, Zhao WM, Zou JP. Low dose of resveratrol enhanced immune response of mice. Acta Pharmacol Sin 2002;23:893-7.
- Wu QL, Fu YF, Zhou WL, Wang TX, Feng YH, Liu J, et al. Inhibition of S-adenosyl-L-homocysteine hydrolase induces immunosuppression. J Pharmacol Exp Ther 2005;313:705-11.
- Phanuphak P, Moorhead JW, Claman HN. Tolerance and contact sensitivity to DNFB in mice. I. In vivo detection by ear swelling and correlation with in vitro cell stimulation. J Immunol 1974;112:115-23.
- Simpson MA, Gozzo JJ. Spectrophotometric determination of lymphocyte mediated sheep red blood cell hemolysis in vitro. J Immunol Methods 1978;21:159-65.
- Bin-Hafeez B, Ahmad I, Haque R, Raisuddin S. Protective effect of Cassia occidentalis L on cyclophosphamide-induced suppression of humoral immunity in mice. J Ethnopharmacol 2001;75:13-8.
- Toungouz M, Donckier V, Goldman M. Tolerance induction in clinical transplantation: the pending questions. Transplantation 2003;75 Suppl 9:58S-60S.
- Offermann G. Immunosuppression for long-term maintenance of renal allograft function. Drugs 2004;64:1325-38.
- Tourkova IL, Yurkovetsky ZR, Shurin MR, Shurin GV. Mechanisms of dendritic cell-induced T cell proliferation in the primary MLR assay. Immunol Lett 2001;78:75-82.
- Amrolia PJ, Muccioli-Casadei G, Yvon E, Huls H, Sili U, Wieder ED, et al. Selective depletion of donor allo-reactive T-cells without loss of anti-viral or anti-leukemic responses. Blood 2003;102:2292-9.
- Tiercy JM, Villard J, Roosnek E. Selection of unrelated bone marrow donors by serology, molecular typing and cellular assays. Transpl Immunol 2002;10:215-21.
- Goes N, Chandraker A. Human leukocyte antigen matching in renal transplantation: an update. Curr Opin Nephrol Hypertens 2000;9:683-7.
- Firestein GS. The T cell cometh: interplay between adaptive immunity and cytokine networks in rheumatoid arthritis. J Clin Invest 2004;114:471-4.
- Matei I, Matei L. Cytokine patterns and pathogenicity in autoimmune diseases. Rom J Intern Med 2002;40:27-41.
- Semenkov VF, Artemjeva OV, Shishina RN, Golubeva VL, Serova LD. Influence of clinical immunosuppression on proinflammatory cytokines and acute-phase proteins. Transplant Proc 1998;30:4195.
- Malek TR, Bayer AL. Tolerance, not immunity, crucially depends on IL-2. Nat Rev Immunol 2004;4:665-74.
- Frew AJ, Varney VA, Gaga M, Kay AB. Immunohistology of human allergic late-phase skin reactions. Skin Pharmacol 1991;4 Suppl 1:71-8.
- Yamachika E, Habte T, Oda D. Artemisinin: an alternative treatment for oral squamous cell carcinoma. Anticancer Res 2004;24:2153-60.