Protective effects of iptakalim, a novel ATP-sensitive potassium channel opener, on global cerebral ischemia-evoked insult in gerbils1
Introduction
Stroke is one of the leading causes of death and long-term disability. Many neuroprotective agents targeting specific pathways involved in ischemic neuronal death (eg, excitotoxicity, [Ca2+]i overload and oxygen stress) have been tested in stroke patients[1]. For the last two decades, a great deal of attention has been paid to neuroprotective therapies. Although initial preclinical studies have demonstrated that numerous drugs are effective for treating acute stroke in animal models, subsequent clinical trials have been frustrating, and almost none of the agents have been proven effective. The reason is that such neuroprotective agents are not able to penetrate the blood-brain barrier and/or they are likely to be degraded into nonbioactive fragments in the blood stream, mostly by the actions of proteases[2]. Thus more efforts should be focused on searching for novel targets so as to develop ideal neuroprotective drugs for stroke.
In 1983, Akinori Noma described a novel type of potassium channel in the membrane of cardiac myocytes characterized by a pronounced inhibition of channel activity when the intracellular ATP concentration was increased[3]. Subsequently, such ATP-sensitive potassium (KATP) channels were found in a range of other tissues including vascular smooth muscle, pancreatic β-cells, and neurons, etc. KATP channels belonged to a class of inwardly-rectifying potassium channels activated by a decrease in the ATP/ADP ratio, which could link cell metabolism to its membrane potential[4]. Although KATP channels in many brain cells were closed under normal conditions and did not contribute to resting membrane properties, membrane hyperpolarization occurred when these channels were activated by selective openers or by decreased levels of oxygen or glucose. Previous studies demonstrated that KATP channels regulated neurotransmitter release and served a protective role in reducing the release of excitatory amino acids during brain ischemia and anoxia[5]. Opening of KATP channels hyperpolarized presynaptic terminals, and thus prevented Ca2+ influx and consequently glutamate release. Furthermore, activation of KATP channels hyperpolarized glutamate-sensitive neurons thereby conferring resistance to the depolarization induced by various glutamate receptors[6]. Therefore, the inhibitory modulatory effect of KATP channels on neuronal excitability in the central nervous system (CNS) might play an important intrinsic role in the development of ischemic brain damage. Abundant studies have documented that KATP channel opener (KCO) exerted definite protective effects on ischemic myocardium, but few studies of KCO as a systemic neuroprotective agent have been published until now[7]. Molecular biological and immunohistochemical studies have shown that some ischemia-sensitive brain areas including hippocampus, globus pallidus, substantia nigra, striatum, and cortex were rich in KATP channels[8], suggesting that KATP channels might play a crucial functional significance and might be an ideal target of neuroprotective agents. Theoretically, blood–brain barrier permeable KCO should be expected to play a protective role against ischemic insult. However, there is not a KCO selectively targeting KATP in CNS and exerting significant neuroprotection with less effect on normal blood pressure.
Recently, we designed and synthesized iptakalim (Ipt), a fatty para-amino compound with low molecular weight[9]. Pharmacological, electrophysiological and biochemical studies have confirmed Ipt as a novel KCO[5,7]. The blood–brain barrier permeability of Ipt provided us with an opportunity to evaluate its neuroprotective effects on CNS by systematic administration[9]. Another superiority of Ipt is the minor adverse effect on normal animal blood pressure compared to diazoxide, the classic mitochondrial ATP-sensitive potassium channel opener. In the present study, we investigated the protective effects of Ipt in ischemic brain insult on gerbils using behavioral tests and histological study. We also report that Ipt could alleviate excitotoxicity by enhancing the glutamate uptake of astrocytes and inhibiting glutamate release in vivo and in vitro.
Materials and methods
Materials PC12 cells were purchased from the Shanghai Institute of Biochemistry, Chinese Academy of Sciences (Shanghai, China). Ipt (99.9%) was synthesized by the Beijing Institute of Pharmacology and Toxicology (Beijing, China). Dulbecco’s modified Eagle’s medium (DMEM) was obtained from Gibco RBL (Grand Island, NY, USA). o-Phthalaldehyde (OPA) was provided by Fluka (Switzerland). All other chemicals and reagents were purchased from Sigma (St Louis, MO, USA).
Global ischemic model Mongolian gerbils (60±5 g, Chinese Academy of Science, Shanghai, China) of both sexes were housed in the animal care facility with a 12-h light, 12-h dark photoperiod and free access to tap water. After acclimation (5–10 d), the animals were anesthetized with sodium amylbarbital (45 mg/kg, ip), the common carotid arteries were visualized via a mid-line neck incision and both arteries were simultaneously occluded for 5 min with non-traumatic micro-aneurysm clips. After occlusion, the clips were removed to allow cerebral reperfusion and the neck incision closed with wound clips throughout the surgery, rectal temperature was maintained at 37±0.5 ºC with a heating blanket with feedback control. Room temperature was regulated to 22–25 ºC by air conditioning[10]. Sham-operated gerbils were killed just after exposing the carotid artery without clamping the vessels. The animals were divided into six groups: sham-operated group, ischemia group, and Ipt groups (0.5, 1.0, 2.0, or 4.0 mg/kg per day, ip). Ipt and saline were administered 40 min prior to carotid artery ligation and subsequently delivered at the same doses (once every day) up to d 7 after surgery.
Behavioral assessment Assessment of locomotor activity was commonly used as behavioral test following global ischemia in the gerbil. On d 1 and d 5 following surgery, all gerbils were placed in an open field maze for 15 min while their locomotor activities were recorded. Locomotor activity included movements across squares and rears (rearing up on haunches), which represented the exploring activities in horizontal and vertical orientation, respectively. On d 2 and d 3, the gerbils were trained in the T-maze (15 pairs of trials/day). On d 4 and d 6, the gerbils were given 15 pairs of trials/day and the percentages of correct choices were determined to measure the working memory of the animals.
Determination of pyramidal cell counts in the hippocampal CA1 region Histological endpoints (counting the remaining number of survival cells or calculating infarct volumes) determined up to 7 d after gerbil global ischemia were customarily used to assess the efficacy of potential therapeutic interventions. For histological endpoints detected, global ischemic gerbils treated with drugs up to d 7 were anesthetized by an overdose of sodium amylbarbital and were then transcardially perfused with 50 mL of saline and 50 mL of 4% buffered polyformaldehyde. The brains were then coronally sectioned at 6 μm. Sections were stained with hematoxylin and eosin, and the cells in the CA1 region of hippocampus were counted.
Measurement of amino acid levels Gerbils were decapitated and the hippocampus was immediately removed and then homogenized in ice-cold homogenizing medium (HClO4 0.4 mol/L). The homogenate was centrifuged (10 000×g, 4 ºC) for 15 min. The supernatant was neutralized with 1.33 volumes of 2.0 mol/L KHCO3. After re-centrifuging at 3000×g at 4 ºC for 5 min, the resulting supernatant was frozen at -80 °C and was used to determine the levels of glutamate by high performance liquid chromatography (HPLC) with fluorescent detection. The HPLC-fluorescent detector system (Shimadzu, Tokyo, Japan) consisted of Shimadzu HPLC, a reverse phase C18 column (Ultrasphere ODS 4.6 mm×250 mm, 5 µm, Japan), fluorescence HPLC monitor RF-530 and a liquid chromatograph LC-6A. The mobile phase consisted of 0.1 mol/L Na2HPO4×12H2O, 0.1 mmol/L edetic acid, methanol 30%, pH 6.04. The emission and excitation wavelengths were set at 425 nm and 338 nm, respectively. The pre-column derivation solution contained OPA (20 mmol/L), β-mercaptoethanol (2 mmol/L), tetraborate (25 mmol/L), 50% methanol, pH 9.6. Samples were mixed with an equivalent volume of derivation solution and were incubated at room temperature (21±1 °C) for 4 min. The derived reaction solutions were used to assay glutamate concentrations at 37 °C with a flow rate of 0.8 mL/min[17].
PC12 cell culture and viability assay PC12 cell were cultured in DMEM supplemented with 10% (v/v) heat-inactivated fetal bovine serum, penicillin 200 kU/L and streptomycin 100 mg/L at 37 °C in atmosphere with 5% CO2. The PC12 cells were then seeded in 96-well plates and grown to 80% confluence in DMEM medium. The cultures were then rinsed with phenol red-free DMEM medium, and 20 µL of MTT solution (dissolved at 5 g/L in PBS) was added to each well. The reaction was stopped after incubation at 37 °C for 4 h by discarding the supernatants, and then 100 µL of dimethylsulfoxide (Me2SO) was added to each well in order to dissolve the resultant dark blue crystal. The absorbance in each well was determined at 570 nm wavelength with an automatic plate reader (TECAN-SUNRISE, F039246A, Austria).
Intracellular calcium measurement The membrane-permeable Ca2+ indicator dye Fluo-3 AM (Molecularprobes, Eugene, OR, USA) was dissolved in Me2SO to produce 1 mmol/L stock solution. For dye loading, cells were incubated with 5 µmol/L Fluo-3 AM diluent for 30 min at 37 °C, followed by 2–3 washes with hydroxyethyl piperazine ethanesulfonic acid solution, with 0.5 mL of this solution for the determination. A Bio-Rad Radiance 2100TM confocal system in conjunction with a Nikon TE300 microscope was used and images were viewed through a 40×magnification lens with a factor N computer zoomed image in a single optical plane. The pixel intensity was used to determine the density value for calcium measures, and ten fields of view were randomly selected for each group.
Measurement of extracellular glutamate of PC12 cell The extracellular glutamate levels of PC12 cell exposure to 80 mmol/L KCl were measured by HPLC with fluorescent detection. An aliquot of culture supernatant was deproteinized with 0.4 mol/L perchloric acid and centrifuged at 12 000×g for 10 min at 4 °C. The supernatants were analyzed by HPLC combined with fluorescent detector analysis after pre-column derivation with OPA as shown above.
Primary culture of astrocytes and assay of glutamate uptake Rat primary astrocytes were prepared from the whole brains of postnatal (1–2-day-old) Sprague-Dawley rats and were plated on poly-D-lysine precoated cell culture flasks containing DMEM (10% fetal calf serum, 100 U/mL penicillin, and 100 µg/mL streptomycin). The cells were grown in a humidified atmosphere of 5% CO2/95% air at 37 °C. When the astrocytes reached confluence, the cells were passaged by trypsinization and plated at a density of 1×106 cells/well on 24-well culture plates in a final volume of 1 mL DMEM, and were grown in a humidified atmosphere of 5% CO2/95% air at 37 °C. Two days later, the astrocytes were used for experimentation. Immunocytochemical analyses have shown that this method produces cultures comprising >95% glial fibrillary acid protein-positive astrocytes.
Glutamate uptake measurements were performed as described previously by Pines and Kanner with little modification[11]. The culture medium was removed and HEPES buffered saline containing [3H]glutamate was added to each well (final concentration was 50 µmol/L). Fifteen minutes later, cells were rapidly washed three times with ice-cold NaCl (0.9%) to terminate glutamate uptake. Cells were then harvested and solubilized in 0.2 mL NaOH (0.3 mol/L) followed by immediate centrifugation (5000×g for 5 min at 4 °C). Radioactivity and protein contents of the supernatant were determined by the liquid scintillation counting and Bradford methods, respectively.
Statistical analysis Data were expressed as mean±SD. Significance of difference between control and samples treated with various drugs was determined by one-way ANOVA followed by post hoc least significant difference (LSD) test. Values of P<0.05 were taken as statistically significant.
Results
Protective effects of Ipt on high locomotor activity of gerbils with global cerebral ischemia The open field maze was a valuable functional test that reflected the degree of CA1 pyramidal neuronal injury induced by global cerebral ischemia. Increased locomotor activity in a novel open field correlates with the severity of ischemia-induced cell death in the CA1 pyramidal layer neuron of the hippocampus. On d 1 after operation, square crossed and rears counted of ischemic gerbils were increased by 40% and 31%, respectively. Treatment with Ipt (0.5, 1.0 mg/kg per day, ip) failed to change ischemia-evoked increase of locomotor activity. However, Ipt (2.0, 4.0 mg/kg per day, ip) could decrease the increases of square crossed and rears counted evoked by the global cerebral ischemia. On d 5, square crossed and rears counted of ischemic gerbils were still significantly higher than that of the sham group. But Ipt (0.5–4.0 mg/kg per day, ip) decreased the increase of square crossed and rears counted induced by the global cerebral ischemia (Figure 1).
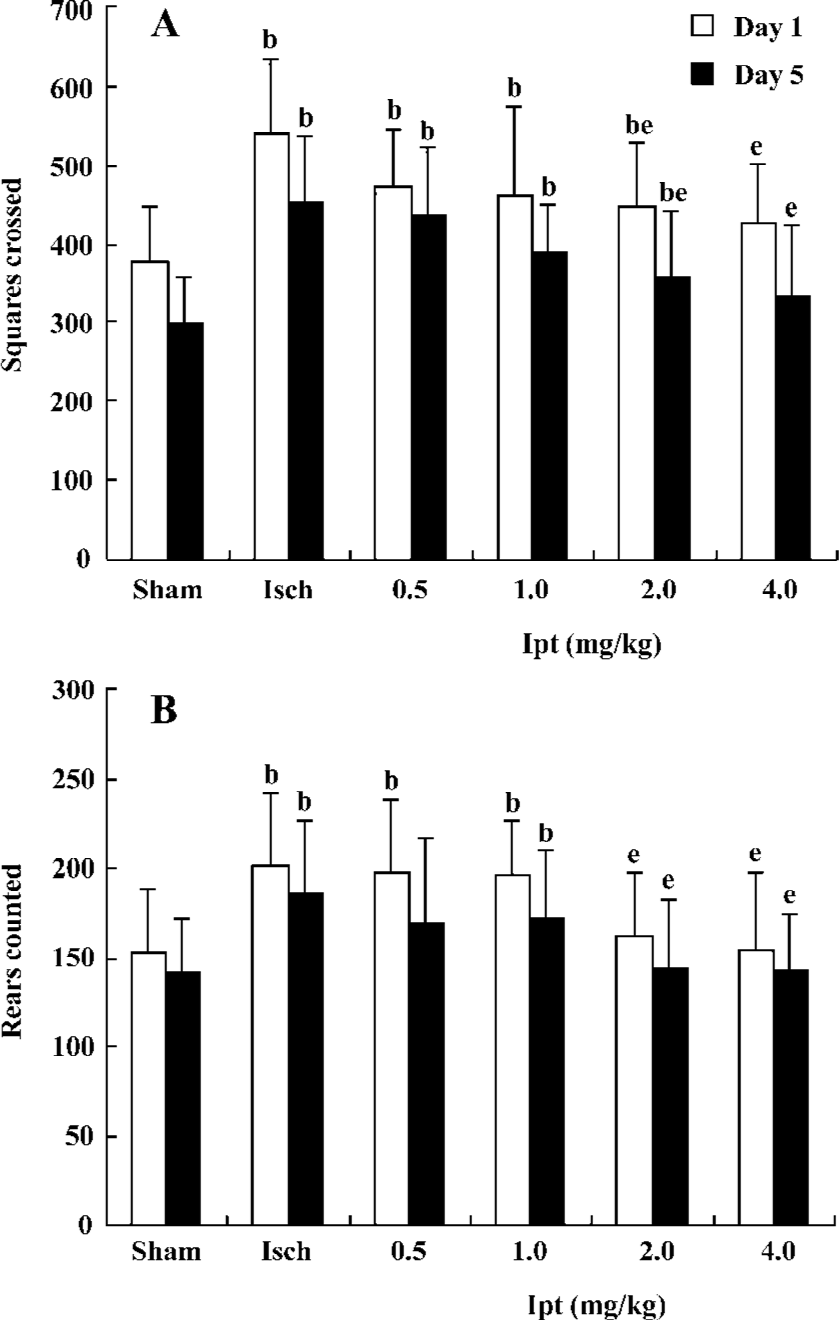
Ipt improved working memory of gerbils with global cerebral ischemia The T-maze task provided a valuable method to assess the function of working memory. On d 2 and d 3, all groups were trained in the T-maze (15 pairs of trials/day). On d 4 and d 6, gerbils were given 15 pairs of trial/day and the % correct to measure working memory of gerbils was calculated. The gerbils with global cerebral ischemia exhibited significant working memory impairments, which could not be improved by Ipt (0.5, 1.0 mg/kg, ip). However, Ipt (2.0, 4.0 mg/kg, ip) could increase the % correct of ischemic gerbils so as to obtain the food reward (Figure 2).
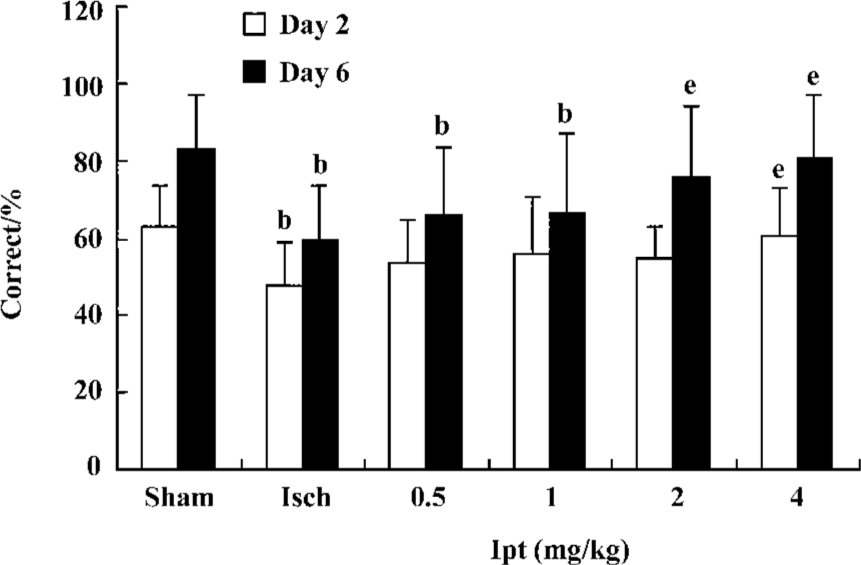
Protective effects of Ipt on the CA1 neurons of gerbils with global cerebral ischemia CA1 neurons are highly susceptible to global ischemic injury and their laminar distribution and large size made them relatively easy to quantify. The results from HE staining showed extensive losses (overall counted averaged ~15% of sham group) of hippocampus CA1 zone pyramidal neurons in gerbils with global cerebral ischemia for 5 min. Ipt (0.5–4.0 mg/kg, ip) could increase the remaining number of healthy neurons in hippocampus CA1 zone in a dose-dependent manner (Figure 3).
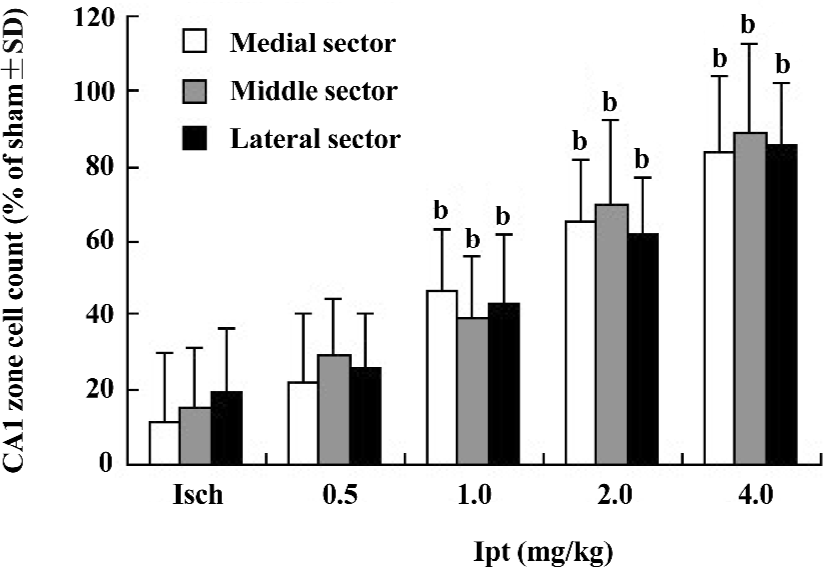
Effects of Ipt on the contents of amino acids in the hippocampus of gerbils with global cerebral ischemia After the ligation of bilateral carotid artery for 5 min and reperfusion for 60 min, the contents of glutamate from hippocampus in gerbils increased by 40%. Pretreatment with Ipt (1.0, 2.0, 4.0 mg/kg, ip) 40 min prior to carotid artery ligation could reverse ischemia-evoked increases of glutamate in hippocampus of gerbils (Figure 4).
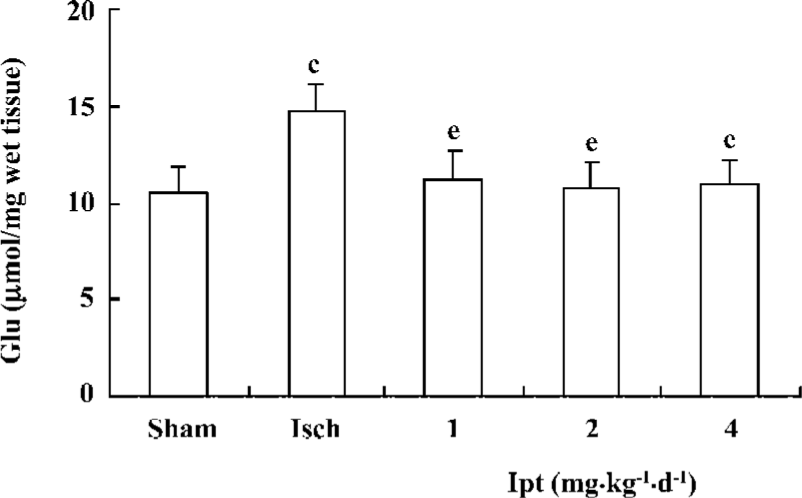
Protective effect of Ipt on glutamate-induced cytotoxicity After pre-treatment of PC12 cells with 10 µmol/L Ipt for 15 min, cells were co-exposed to 10 µmol/L Ipt and 10 mmol/L glutamate for 24 h, after which the cell viability was measured. The viability of Ipt treated cells was 95%, which was significantly higher than that of glutamate treated cells (79%). Application of the classic KATP channels opener pinacidil showed similar protective effects. Pre-incubation of PC12 cells with glibenclamide, a KATP channels blocker, for 15 min, could abolish the protective effects of Ipt and pinacidil (Figure 5).
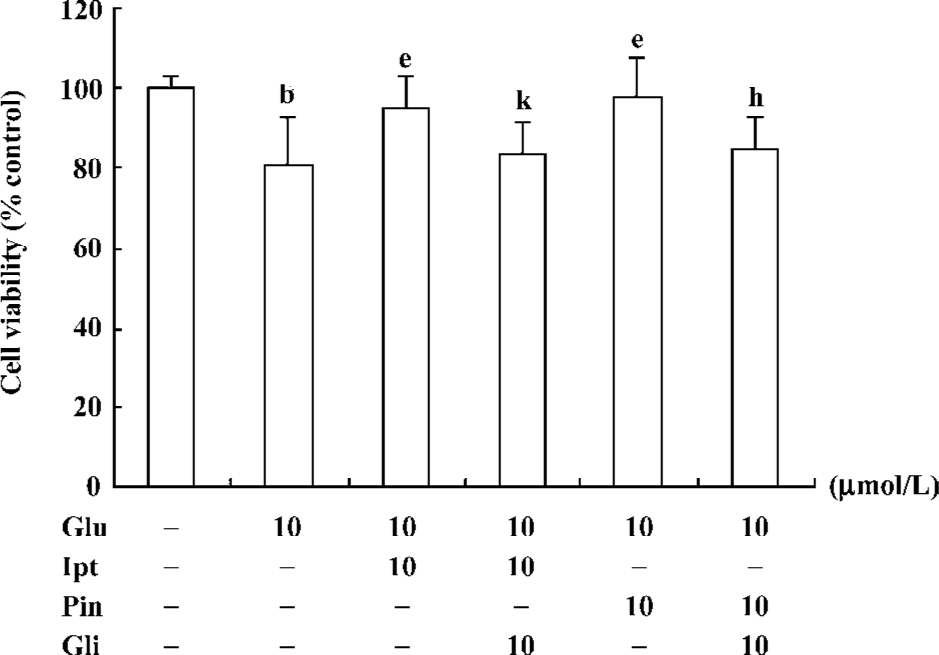
Effects of Ipt on the elevation of intracellular calcium induced by glutamate Fluorometric Ca2+ measurements demonstrated that cells pre-treated with 10 µmol/L Ipt or pinacidil had lower Ca2+ in PC 12 cells compared to that treated with 10 mmol/L glutamate for 24 h (Figure 6). Likewise, the reduction of intracellular Ca2+ overload was attenuated by glibenclamide.
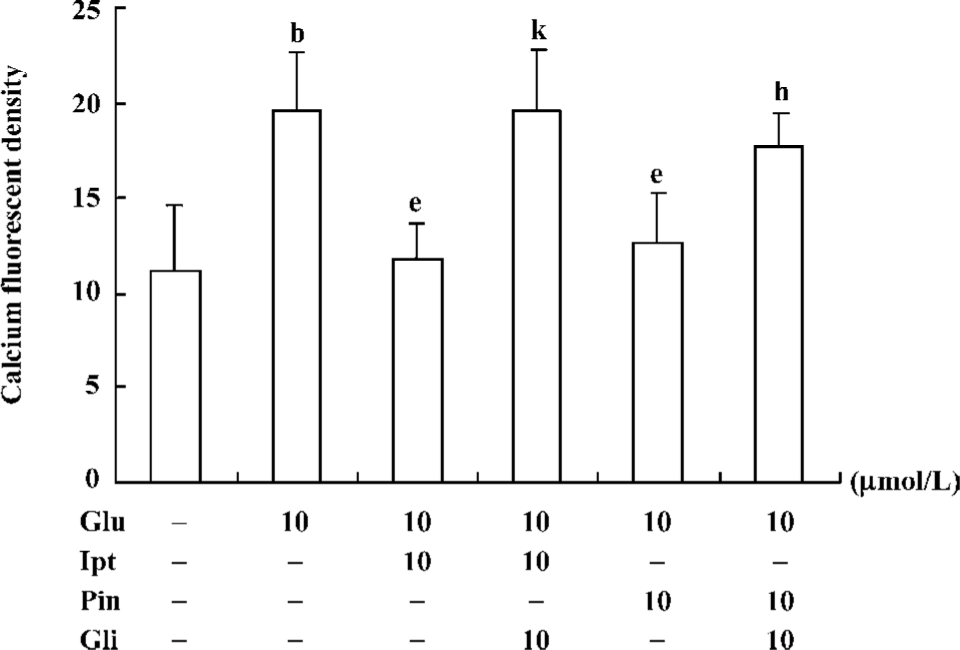
Effects of Ipt on extracellular glutamate levels of PC12 cell incubated with high extracellular K+ PC12 cell were pre-incubated with different concentrations of Ipt before high extracellular K+ treatments. As shown in Figure 7, pre-treatment with different concentrations of Ipt for 15 min reduced extracellular glutamate levels of PC12 cell induced by high extracellular K+ in a concentration-dependent manner. Glibenclamide reversed the inhibitory effects of Ipt (Figure 7).
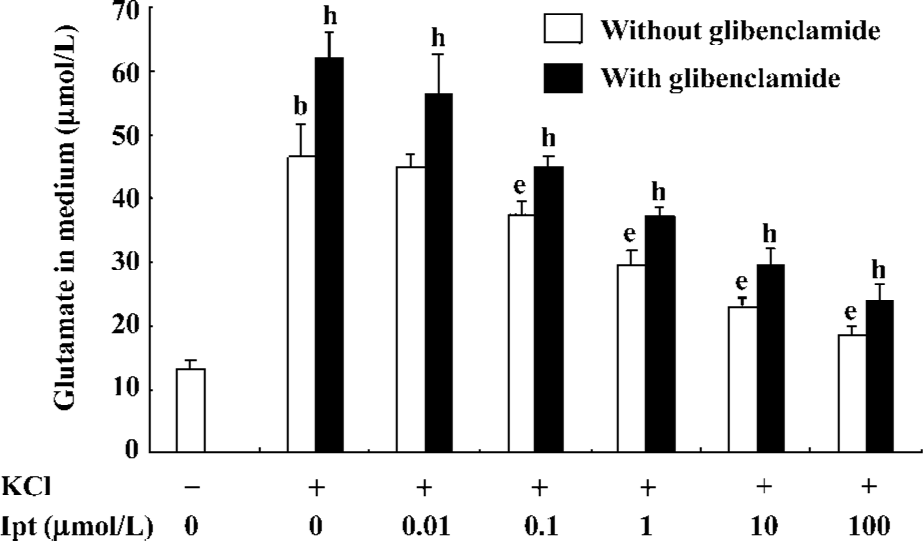
Effects of Ipt on astrocytic glutamate uptake Primary cultured astrocytes were incubated with different concentrations of Ipt for 24 h. Astrocytic glutamate uptake was determined by measuring intracellular concentrations of [3H]D,L-glutamate using isotope techniques. Ipt (10, 100 µmol/L) could enhance astrocytic glutamate uptake (Figure 8).
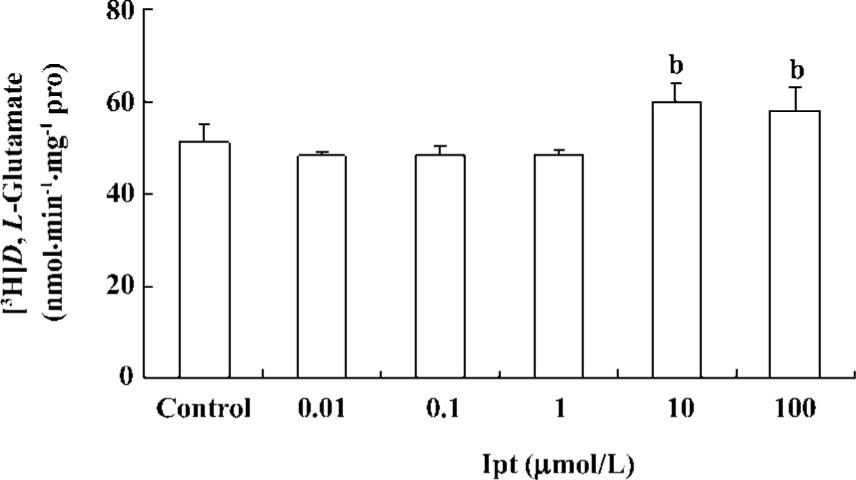
Discussion
Our studies indicated that Ipt (0.5–4.0 mg/kg, ip) could reduce the high locomotor activity evoked by ischemia and improve global cerebral ischemia-induced working memory impairments. Ipt did not affect the locomotor activity and working memory of normal gerbils in the preliminary experiments. Histological study revealed that Ipt (0.5–4.0 mg/kg per day, ip) could increase the survival neuron in hippocampus CA1 zone in a dose-dependent manner. Moreover, Ipt (1.0, 2.0, 4.0 mg/kg, ip) 40 min prior to carotid artery ligation could reverse ischemia-evoked increases of glutamate in the hippocampus of gerbils. According to previous studies, hippocampal glutamate levels were thought to represent the extracellular released glutamate[12]. Ipt could protect PC12 cells against glutamate induced cytotoxicity by reducing the [Ca2+]i and extracellular glutamate levels of PC12 cells and enhancing the glutamate uptake activity of primary cultured astrocytes.
Brief periods of global brain ischemia in rodents caused delayed cell death in hippocampal CA1 pyramidal neurons days after reperfusion. A similar phenomenon occurred after ischemic injury in humans[13]. The cause of such delayed neuronal damage in the CA1 neurons has not been fully understood, although many mechanisms have been proposed. Among them, glutamate excitotoxicity has played a critical role[14].
Glutamate, the major excitory neurotransmitter in the CNS, is the main cause of excitotoxicity in CNS pathology[15]. As glutamate neurotoxicity was considered to be initiated by excessive Ca2+ influx resulting from the overactivation of the NMDA receptor, it was generally accepted that one of the most important pathophysiological factors underlying glutamate-induced cellular damage was the failure of intracellular Ca2+ homeostasis[16]. Excessive activation of the NMDA receptor could induce a massive influx of extracellular calcium, which further activated a series of calcium-dependent enzymes involved in the catabolism of proteins and eventually resulted in neuronal apoptosis[17]. Therapeutic strategies for cerebral ischemia have been developed to inhibit the downstream occurrence of glutamate receptor overactivation-induced pathophysiological reactions. However, more attention should been given to reducing the glutamate levels in the synaptic cleft. Two main processes are involved in maintaining physiological glutamate concentrations at the synaptic cleft: one regulates glutamate release from the presynaptic membrane, and the other regulates glutamate uptake, which is mainly mediated by high affinity glutamate transporters located in astrocytes[6,18].
Ipt has been demonstrated as a novel KCO and a promising neuroprotectant with previous results showing that Ipt protected substantia nigra dopaminergic neurons against a variety of neurotoxins (eg, MPP+, 6-OHDA or rotenone) in vitro and in vivo[6,19–21]. In this study, we assessed the protective effect of Ipt on global cerebral ischemia through systematic administration. To explore the protective mechanism regarding excitotoxicity, we found that Ipt could reverse global cerebral ischemia induced increase of glutamate levels in the hippocampus of gerbils, which may be an important neuronal mechanism of its neuroprotective role. Furthermore, Ipt inhibited glutamate release from PC12 cells evoked by high extracellular K+ and reduced intracellular Ca2+ of PC12 cells, which could be abolished by KATP channel blocker glibenclamide, suggesting it was the consequence of, at least partly, activation of KATP channels. Meanwhile, Ipt exhibited potent neuroprotective capacities in PC12 cells exposed to glutamate. Several lines of evidence indicated that the activation of KATP channels inhibited neurotransmitter releases such as acetylcholine from rat striatal slices, catecholamide from PC12 cell[5] and glutamate release from embryonic chick telencephalon neuron by causing hyperpolarization[22]. Combined with the results of the present study, we hypothesized that the inhibitory regulation of glutamate release was involved in the neuroprotection of Ipt on ischemia-evoked neuronal death.
KATP channels exist both in astrocytes in adult rats and newborn rat primary astrocytes[18,23,24]. In the present study we revealed that Ipt could enhance the glutamate uptake of primary cultured astrocytes. Our previous in vitro analysis and in vivo microdialysis experiments indicated that Ipt could reduce extracellular glutamate levels[18,21,25]. Therefore, we hypothesized that Ipt alleviated glutamate excitotoxicity in two ways: one was to inhibit glutamate release and the other was to enhance glutamate uptake of astrocytes. How did Ipt regulate the activity of astrocytic glutamate uptake? The activity of Na+-dependent glutamate transporters was governed by both thermodynamic and kinetic factors, such as Na+/K+ gradients[26]. As the driving forces were all supplied by ATP hydrolysis, opening of KATP channels resulted in the preservation of intracellular ATP production, hence, subsequently elevated activity of glutamate transporter. Moreover, the activation of KATP channels lead to the almost immediate activation of protein kinase C[27]. Activators of protein kinase C, such as phorbol esters, rapidly stimulate glutamate transporter activity[28]. So it is proposed that Ipt may enhance glutamate uptake mediated by glutamate transporters by the opening of KATP channels, subsequently augmenting ATP production and PKC-mediated phosphorylation.
In conclusion, Ipt, a novel and blood-brain barrier permeable KCO, could effectively protect brain neurons against glutamate neurotoxicity in in vitro and in vivo animal ischemic models. The mechanisms underlying the neuroprotection of Ipt may involve the opening of KATP, diminishing glutamatergic synaptic transmission by inhibiting presynaptic glutamate release, alleviating postsynaptic ionotropic glutamate receptor such as NMDA receptor overdepolarization-induced events, enhancing glutamate uptake of astrocytes, and reducing intracellular Ca2+ stores. Accordingly, Ipt is not only a useful pharmacological tool for systemic investigation on KATP channels in CNS but also a promising therapeutic agent with few side-effects for stroke.
References
- Labiche LA, Grotta JC. Clinical trials for cytoprotection in stroke. NeuroRx 2004;1:46-70.
- Zhang B, Hata R, Zhu P, Sato K, Wen TC, Yang L, et al. Prevention of ischemic neuronal death by intravenous infusion of a ginseng saponin, ginsenoside Rb(1), that upregulates Bcl-x(L) expression. J Cereb Blood Flow Metab 2006;26:708-21.
- Noma A. ATP-regulated K+ channel in cardiac muscle. Nature 1983;305:147-8.
- Liss B, Roeper J. Molecular physiology of neuronal K-ATP channels. Mole Membr Biolo 2001;18:117-27.
- Taylor SC, Carpenter E, Roberts ML, Peers C. Potentiation of quantal catecholamine secretion by glibenclamide: evidence for a novel role of sulphonylurea receptors in regulating the Ca2+ sensitivity of exocytosis. J Neurosci 1999;19:5741-9.
- Wu J, Wakui M, Wang H, Hu G. Iptakalim hydrochloride and neuronal protection. Current Neuropharmacol 2005;3:249-56.
- Rodrigo GC, Standen NB. ATP-sensitive potassium channels. Curr Pharm Des 2005;11:1915-40.
- Zawar C, Plant TD, Schirra C, Konnerth A, Neumcke B. Cell-type specific expression of ATP-sensitive potassium channels in the rat hippocampus. J Physiol 1999;514:327-41.
- Wang H. Pharmacological characteristics of the novel antihypertensive drug, iptakalim hydrochloride, and its molecular mechanisms. Drug Develop Res 2003;58:65-8.
- Nakanishi H, Katsuta K, Koide T, Ueda Y, Shirakawa K, Yoshida K. Protective effect of FR115427 against ischemic hippocampal damage in gerbils. Jpn J Pharmacol 1994;64:189-93.
- Pines G, Kanner BI. Counterflow of 1-glutamate in plasma membrane vesicles and reconstituted preparations from rat brain. Biochemistry 1990;29:11209-14.
- Tang XC, Rao MR, Hu G, Wang H. Alterations of amino acid levels from striatum, hippocampus, and cerebral cortex induced by global cerebral ischemia in gerbil. Acta Pharmacol Sin 2000;21:819-23.
- Petito CK, Feldmann E, Pulsinelli WA, Plum F. Delayed hippocampal damage in humans following cardiorespiratory arrest. Neurology 1987;37:1281-6.
- Weiser T. AMPA receptor antagonists for the treatment of stroke. Curr Drug Targets CNS Neurol Disord 2005;4:153-9.
- Meddrum BS. Glutamate as a neurotransmitter in the brain: review of physiology and pathology. J Nutr 2000;130:1007s-15s.
- Lee KH, Park JY, Kim K. NMDA receptor-mediated calcium influx plays an essential role in myoblast fusion. FEBS Lett 2004;578:47-52.
- Kawasaki A, Han MH, Wei JY, Hirata K, Otori Y, Barnstable CJ. Protective effect of arachidonic acid on glutamate neurotoxicity in rat retinal ganglion cells. Invest Ophthalmol Vis Sci 2002;43:1835-42.
- Hu LF, Wang S, Shi XR, Yao HH, Sun YH, Ding JH, et al. ATP-sensitive potassium channel opener iptakalim protected against the cytotoxicity of MPP+ on SH-SY5Y cells by decreasing extracellular glutamate level. J Neurochem 2005;94:1570-9.
- Yang Y, Liu X, Ding JH, Sun J, Long Y, Wang F, et al. Effects of iptakalim on rotenone-induced cytotoxicity and dopamine release from PC12 cell. Neurosci Lett 2004;366:53-7.
- Yang Y, Liu X, Long Y, Wang F, Ding JH, Liu SY, et al. Systematic administration of iptakalim, an ATP-sensitive potassium channel opener, prevents rotenone-induced motor and neurochemical alterations in rats. J Neurosci Res 2005;80:442-9.
- Wang S, Hu LF, Zhang Y, Sun T, Sun YH, Liu SY, et al. Effects of systemic administration of iptakalim on extracellular neurotransmitter levels in the striatum of unilateral 6-hydroxydopa-mine-lesioned rats. Neuropsychopharmacology 2006;31:933-40.
- Wind T, Prehn JH, Peruche B, Krieglstein J. Activation of ATP-sensitive potassium channels decreases neuronal injury caused by chemical hypoxia. Brain Res 1997;751:295-9.
- Brockhaus J, Deitmer JW. Developmental downregulation of ATP-sensitive potassium conductance in astrocytes in situ. Glia 2000;32:205-13.
- Zhou M, Tanaka O, Suzuki M, Sekiguchi M, Takata K, Kawahara K, et al. Localization of pore-forming subunit of the ATPsensitive K(+)-channel, Kir6.2, in rat brain neurons and glial cells. Brain Res Mol Brain Res 2002;101:23-32.
- Yang J, Hu LF, Liu X, Zhou F, Ding JH, Hu G. Effects of iptakalim on extracellular glutamate and dopamine levels in the striatum of unilateral 6-hydroxydopamine-lesioned rats: a microdialysis study. Life Sci 2006;78:1940-4.
- Anderson CM, Swanson RA. Astrocyte glutamate transporter: review of properties, regulation, and physiological functions. Glia 2000;32:1-14.
- Kis B, Rajapakse NC, Snipes JA, Nagy K, Horiguchi T, Busija DW. Diazoxide induces delayed pre-conditioning in cultured rat cortical neurons. J Neurochem 2003;87:969-80.
- Yao HH, Ding JH, Zhou F, Wang F, Hu LF, Sun T, et al. Enhancement of glutamate uptake mediates the neuroprotection exerted by activating group II or III metabotropic glutamate receptors on astrocytes. J Neurochem 2005;92:948-61.