Differentiation of bone marrow mesenchymal stem cells induced by myocardial medium under hypoxic conditions
Introduction
Cell transplantation to regenerate injured tissues is a promi- sing new treatment for patients suffering from several diseases. Bone marrows contain a population of progenitor cells known as mesenchymal stem cells (MSC), which can colonize different tissues, replicate and differentiate into multilineage cells. Recent evidence suggests that MSC can transdifferentiate into cardiomyocytes in vivo or in vitro, and that the cardiac microenvironment might play a critical role in MSC transdifferentiation. Furthermore, the intercellular direct interaction between cardiomyocytes and MSC may be the main inducing factor[1]. It is surprising that MSC transplanted into the infarcted myocardium could be induced to acquire the phenotypical characteristics of cardiomyocytes, which suggests that MSC transdifferentiation might be related to the hypoxic microenvironment[2]. Cardiac precursor cells are equipped for differentiating in a hypoxic environment using the anaerobic metabolism for energy production[3]. To understand whether MSC could differentiate into myocardial-like cells induced by the soluble signaling molecules in the cardiac microenvironment, we simulated the normal and hypoxic cardiac microenvironment in vitro by incubating MSC with the conditioned medium of normal cardiomyocytes or cardiomyocytes after hypoxia reoxygenation, and investigated whether the mediums could induce MSC differentiation.
Materials and methods
Cell cultures Sprague-Dawley rats (265±15 g, Grade II) were obtained from the Experimental Animal Center of Zhejiang Medical Sciences Academy (Zhejiang, Hangzhou, China). The MSCs were obtained from the femurae and tibiae of rats with a modified method originally described by Dobson et al[4,5]. In order to collect the cells more efficiently, the bones were mounted in microfuge tubes after the proximal ends were removed and centrifuged at 900×g for 2 min. The marrow pellet was washed in phosphate-buffered salt solution (PBS), centrifuged at 900×g for 10 min and then resuspended in Dulbecco’s modified Eagle’s medium (DMEM; Gibco, Carlsbad, CA, USA). Nucleated cells were isolated with a density gradient centrifugation (Ficoll or Paque), then introduced into a 25-cm2 flask (Falcon, Oxnard, CA, USA) and cultured (at a density of 5×107 cell/mL) at 37 oC in humidified air with 5% CO2 in DMEM containing 10% fetal calf serum (Gibco, Carlsbad, CA, USA), penicillin (100 U/mL) and streptomycin (100 mg/mL). The medium was changed to remove nonadherent cells 48 h after seeding and every 4 d thereafter. Each primary culture was replated to 2 new flasks when the MSC grew to approximately 70% confluence. For subculture, the cells were resuspended with 0.25% trypsin and passaged at a ratio of 1:2 plates. For the 2 passages, homogeneous MSC devoid of haematopoietic cells were used for the experiments. The cells were determined by fluorescence-activating cell sorting (FACS; Beckman Coulter, Fullerton, CA, USA) analysis before the experiments, using directly conjugated antibodies against anti-rat CD44 [fluorescein isothiocyanate conjugated (FITC), Caltag, San Francisco, CA, USA], anti-CD45 (FITC, Caltag, San Francisco, CA, USA) and anti-CD90 [phycoerythrin-conjugated (PE), Caltag, San Francisco, CA, USA].
Primary culture of neonatal rat cardiomyocytes was prepared by the method originally described by Simpson and Savion[6] with minor modifications. Briefly, the hearts from 1- or 3-d-old Sprague-Dawley rats were minced and dissociated with 0.125% trypsin (Gibco, Carlsbad, CA, USA) and 0.1% collagenase type II (Worthington, Lakewood, NJ, USA). After the incubation of dispersed cells on a 25-cm2 flask for 60 min at 37 °C in humidified air with 5% CO2, the unattached viable cells were collected and seeded into a 25 cm2 flask (2×106 cell/dish) or 24-well plates (2×105 cells/well) and incubated at 37 °C in a 5% CO2 incubator. The cells were then incubated with DMEM supplemented with 10% fetal calf serum and 0.1 mmol/L 5'-bromo-2'-deoxyuridine (Sigma, St Louis, MO, USA) for 72 h to prevent low-level nonmyocardial cell proliferation, and then replaced with DMEM plus 10% calf serum before the experiments. The cultured medium was changed 24 h after seeding and then every 2 d.
Hypoxia/reoxygenation model of cardiomyocytes The hypoxic condition was produced as reported previously[7,8]. Briefly, cardiomyocytes were placed in an air-tight plexiglass humidified chamber maintained at 37 °C with <1% O2 (measured with a CY-100 oxygen concentration monitor, Hangzhou Lihua Tech, Hangzhou, Zhejiang, China), where the air could be completely replaced by a gas mixture of 95% N2 and 5% CO2 to produce hypoxic conditions. Myocardial cells were placed into the hypoxia chamber where they remained for 2 h, then incubated overnight at 37 °C in 5% CO2 incubator for reoxygenation. The conditioned mediums of the normal cardiomyocytes and cardiomyocytes after hypoxia reoxygenation were sucked and centrifuged at 1200×g for 5 min, then collected for the experiments. Of the collected mediums, 1 mL was used for lactate dehydrogenase (LDH) examination by an automatic biochemistry meter (Beckman LX20, Fullerton, CA, USA). The LDH levels were also monitored in the ordinary DMEM as the negative control.
Protocols The present study comprised of 8 groups and the experimental protocols were performed as follows. In group A, the MSC were incubated with ordinary DMEM for 72 h as the negative control. The conditioned medium of the normal cardiomyocytes was added into the MSC culture systems and incubated for 24 h (group B1), 48 h (group B2) and 72 h (group B3). The conditioned medium of cardiomyocytes after hypoxia/reoxygenation was used to incubate MSC for 24 h (group C1), 48 h (group C2) and 72 h (group C3), respectively. Cardiomyocytes as the positive control in group D were also included.
Immunofluorescence microscopy Cells plated on 24-well plates were washed twice in PBS and fixed in methanol for 10 min at -20 °C. Fixed cells were permeabilized with 0.5% (v/v) Triton X-100 in PBS for 15 min and incubated with 10% normal goat serum or bovine serum albumin for 30 min at room temperature. The cells were then incubated with primary antibodies diluted in PBS (1:100) for 1 h at room temperature or overnight at 4°C[goat anti-myosin heavy chain (MHC K-16) and goat anti-troponinT-C (TnT C-19), Santa Cruz Biotech, Santa Cruz, CA, USA]. Mouse anti-connexin 43 (CX43C13-M; Analog Devices Inc, Norwood, MA, USA) was also diluted in PBS for 1 h at room temperature or overnight at 4 °C. After 3 washes with PBS for 10 min, the cells were reincubated with secondary antibodies (rhodamine-conjugated rabbit anti-goat IgG, FITC rabbit anti-goat IgG and rhodamine-conjugated goat-mouse IgG) for 1 h at room temperature. The cells were then rewashed in PBS on a rocking platform. Immunostained cells were reincubated with 4',6-diamidino-2-phenylindole (DAPI, Kirkegaard & Perry Laboratories Inc., Gaithersburg, MD, USA) for 15 min and rewashed in PBS, then immediately analyzed using confocal laser microscopy with a 60× objective (FV1000, Olympus, Shinjuku-ku, Tokyo, Japan). The negative control experiments used preimmunized serum as the primary antibody. The proportion of differentiated MSC into myocardial lineage was measured by counting the average numbers of stained cytoplasm of all the MSC per high-power field under fluorescence microscopy with a 20×bjective (Axiovert-200, Carl Zeiss, Oberkochen, Baden-Wuerttemberg, Germany). Each sample was counted randomly in 8 separate high-power fields.
Electrophoresis and immunoblotting Cells plated on the 25-cm2 flask were washed with cold PBS and scraped onto a 100 μL lysis buffer consisting of 50 mmol/L Tris (pH 8.0), 150 mmol/L NaCl, 1% Triton X-100, 0.5% NP-40, 1 mmol/L EDTA, 1 mmol/L EGTA, 1 mmol/L sodium orthovanadate, 1 mmol/L phenylmethylsulfonylfluoride, 50 mmol/L sodium fluoride, and 5 mg/mL aprotinin. The total protein of every sample was quantitated by pierce bicinchoninic acid (BCA) reagent (Pierce Biotechnology Inc, Rockford, IL, USA). Protein 80 mg was loaded per lane and electrophoresed in 12% sodium dodecyl sulfate polyacrylamide gels (SDS-PAGE). The transfer onto polyvinylidene fluoride (PVDF) membranes was made in 25 mmol/L Tris-HCl (pH 8.3), 192 mmol/L glycine and 20% methanol using a transblot apparatus (Bio-Rad, San Diego, CA, USA) at 350 mA for 1 h at 4 °C. The membranes were saturated in 10 mmol/L Tris-HCl (pH 8.0), 150 mmol/L NaCl, 0.05% Tween 20 and 10% non-fat dry milk for 20 min at room temperature and then probed with specific polyclonal goat anti-troponin-C (C-19, 1:200; Santa Cruz Biotech, Santa Cruz, CA, USA) and monoclonal mouse anti-connexin 43 (1:5000; Analog Devices Inc., Norwood, MA, USA) in the same buffer overnight at 4 °C with gentle agitation. The membranes were washed 3 times with 10 mmol/L Tris-HCl (pH 8.0), 150 mmol/L NaCl and 0.05% Tween 20. Bound antibodies were identified after incubation with peroxidase-conjugated anti-goat antibodies and peroxidase-conjugated anti-mouse antibodies (1:5000 dilution in saturation buffer, individually) for 1 h at room temperature. The membranes were then rewashed 3 times and the position of the individual proteins in separate lanes was detected by chemiluminescence ECL using radiographic film (X-Omat AR-5, Eastman Kodak, Kingsport, TN, USA). NIH ImageJ (National Institutes of Health, Bethesda, MD, USA) was used to analyze the protein loads in each lane.
Statistical analyses Data were expressed as Mean±SD. One-way analysis of variance (ANOVA) followed by multiple comparison methods by Scheffe or Student’s t-test was used for statistical analysis. A P value of less than 0.05 was considered statistically significant.
Results
Morphology and surface analysis of the mesenchymal stem cell line The MSC attached on culture dishes sparsely and the majority of the cells displayed a spindle-like shape (Figure 1A). A true surface marker for MSC has yet to be identified. The putative MSC used in the experiments expressed CD44 and CD90 at moderate to high levels by flow cytometry analyses. The cells were negative for CD45 (a surface marker for haematopoietic stem cells) (Figure 1B).
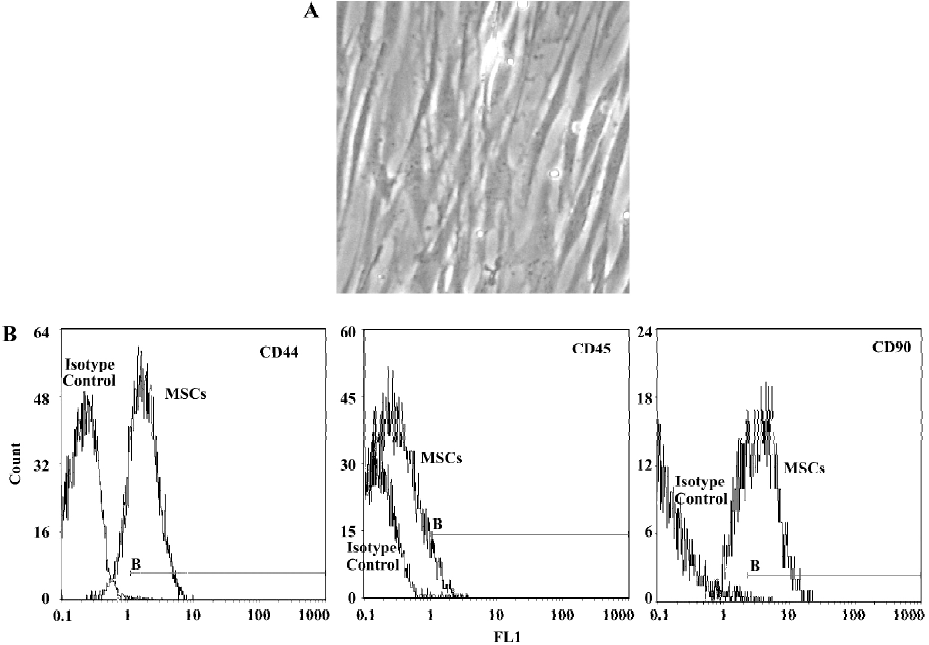
Determination of LDH values in mediums The LDH levels in the conditioned medium of cardiomyocytes after hypoxia reoxygenation were significantly higher than those in the conditioned medium of the normal cardiomyocytes and control DMEM medium (80.8?4.3 U/L vs 42.0?8.7 U/L and 28.7?6.4 U/L; P<0.05).
Expression of cardiac proteins in MSC MSC could be induced to express cardiac specific proteins after incubation with the conditioned medium of cardiomyocytes after hypoxia/reoxygenation for 24 h, 48 h and 72 h. Immunofluorescence analyses clearly detected cardiac MHC and TnT in the MSC of group C (C1 to C3) as shown in Figures 2C and 2G, while not in the MSC of group B (B1 to B3) as shown in Figures 2B and 2F. The differentiation proportion of MSC into myocardial lineage was 95.0%±1.8%, 97.2%±1.0% and 98.6%±1.4%, respectively in groups B1, B2 and B3; there was no significance of the differentiation percentages among the 3 subgroups. Connexin 43 could was not detected in the MSC of groups B and C. Western blotting analyses could detect TnT expressions in the MSC of group C, but could not detect the expressions of connexin 43. Neither TnT nor connexin 43 could be detected in the MSC of group B (Figure 3). The reliability of the analyses was confirmed with cultured cardiomyocytes under the same conditions. Semiquantitive analysis showed there was no statistical significance in the expressions of TnT among group C (P>0.05).
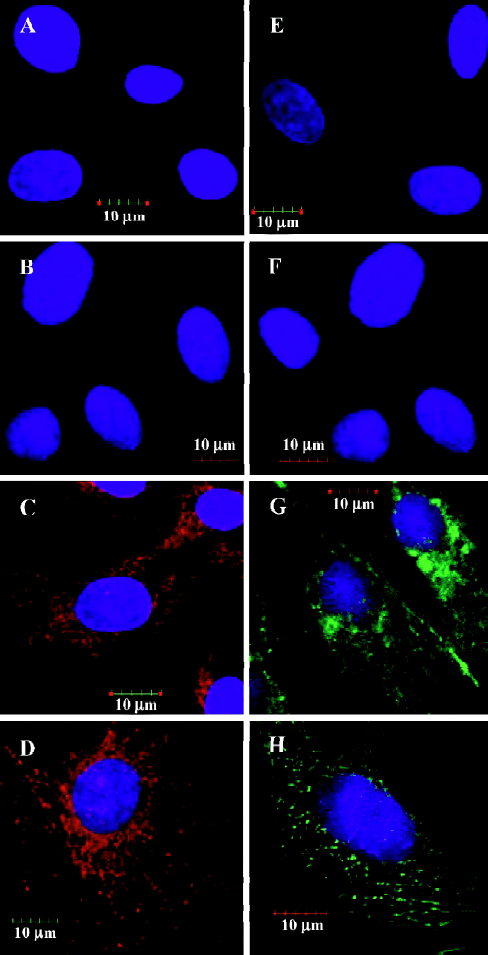
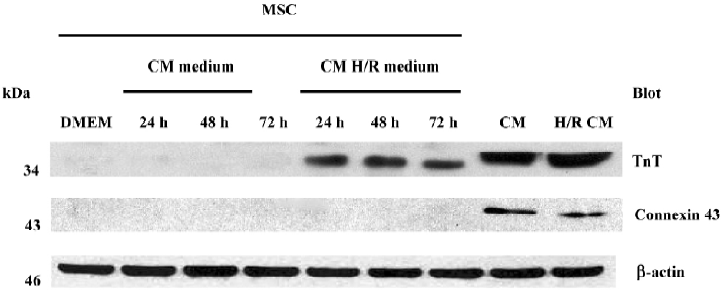
Discussion
The major new finding in the present study is that MSC incubated with the conditioned medium of cardiomyocytes after hypoxia/reoxygenation can express cardiac MHC and TnT.
MSC are nonhematopoietic multipotent stem cells that exist in bone marrows for the whole lifespan of mammals. Considering their advantages, such as the ease of obtaining bone marrow aspiration by a simple routine, the ability to self-renew, and the recently reported potential to differentiate into cardiomyogenic cells, MSC have been considered one of the most promising candidates for repairing damaged cardiomyocytes. The potential of MSC differentiation into myogenic cells was first reported by Wakitani et al[9] and then by a number of other researchers[10]. MSC exposed to 5-azacytidine were able to form myotubes and express myocardial specific proteins, such as cardiac troponin I and MHC, which also exhibit sinus node-like or ventricular cell-like action potentials and beat spontaneously. Combined treatment with bone morphogenetic protein-2 (BMP-2) and fibroblast growth factor-4 (FGF-4) could also result in MSC cardiac differentiation[11]. It was reported that the cardiac microenvironment could induce transplanted MSC to differentiate into myocardial-like cells. MSC directly co-cultured with adult rat cardiomyocytes for 1 week could express cardiac contractile proteins, such as alpha-actin, desmin and cardiac troponin. These cells expressed Nkx2.5, GATA-4, TEF-1, and MEF-2C mRNA after co-culture and presented gap junctions with cardiomyocytes nearby. Direct cell-to-cell contact between MSC and adult cardiomyocytes might be necessary in the differentiation of MSC into cardiomyocytes[1,12]. Moreover, myocardial cell lysate could also induce MSC differentiating into myocardial-like cells in vitro[13]. It remains to be identified whether the soluble signaling molecules of the cardiac microenvironment have any effect on MSC differentiation and which molecules are most important for the induction.
LDH is usually used as a marker of the integrity of cell membranes[14]. Hypoxia can cause the increase of myocardial apoptotic rates and the release of LDH, while reoxygenation not only further increases the apoptotic rates and leakage of LDH, but also induces necrosis of cardiomyocytes. Severe hypoxia is cytotoxic to cardiomyocytes, while mild hypoxia has not been shown to induce significant cytotoxicity in cultured cardiomyocytes[15]. Moreover, protein synthesis is significantly upregulated under mild hypoxic conditions, while stress mechanisms are stirred up resulting in the intracellular release of cytokines[16,17]. In present study, the myocardial cells was treated with mild hypoxic preconditioning, and the LDH levels in the mediums were monitored to quantify the reversible damages of myocardial cells and explain the possibility of signaling molecules released by cardiomyocytes.
We simulated the normal and hypoxic microenvironment of myocardium in vitro to investigate whether the intracellular soluble signaling molecules of myocardial medium could induce MSC differentiation into cardiomyocytes. The present study demonstrates that conditioned medium with cardiomyocytes after hypoxia/reoxygenation could induce MSC to express MHC and TnT in vitro, but conditioned medium with normal cardiomyocytes had no induction of MSC differentiation. Based on these results, we proposed that MSC differentiation might be associated with some soluble signaling molecules in the conditioned medium with cardio-myocytes after hypoxia/reoxygenation. It still remains to be clarified which of the signaling molecules plays a critical role in MSC differentiation. Several recent reports have emphasized the regulation of transcription and growth factors that are involved in cardiac cell differentiation, such as Nkx2.5, GATA-4, MEF-2C, and TGF-β[18]. Intracellular signaling transduction pathways are activated to result in induction events or milieu-dependent differentiation. Factors acting in both an autocrine or paracrine manner can activate new gene expression resulting in subsequent extracellular and intracellular changes, including contractile protein synthesis and membrane receptor expression. Cultured cardiomyocytes in vitro could secrete some signaling molecules and cytokines induced by hypoxic preconditioning, such as cyclophilin A, adenosine and hypoxia-inducible factor-1α[8,19,20]. Linask and colleagues identified the presence of the hypoxia markers during early cardiomyogenesis, indicating that cardiac cell differentiation occurs in a hypoxic environment using the anaerobic metabolism for energy production[3]. This led us to support the hypothesis that myocardial cells could secrete some signaling molecules and result in activation of the differentiation process.
The MSC in our study did not express connexin 43, which could lead to the presumption that the expressions of an intracellular gap junction would be dependent on direct electrical and mechanical contact between MSC and cardiomyocytes.
In conclusion, the results of the present study show that conditioned medium with cardiomyocytes after hypoxia/reoxygenation can induce MSC to express cardiac specific contractile proteins in vitro. This study confirms that the soluble signaling molecules in the hypoxic microenvironment are also ideal inducers of MSC differentiation in myocardial-like cells.
References
- Shim WS, Jiang S, Wong P, Tan J, Chua YL, Tan YS, et al. Ex vivo differentiation of human adult bone marrow stem cells into cardiomyocyte-like cells. Biochem Biophys Res Commun 2004;324:481-8.
- Wang JS, Shum-Tim D, Galipeau J, Chedrawy E, Eliopoulos N, Chiu RC. Marrow stromal cells for cellular cardiomyoplasty: feasibility and potential clinical advantages. J Thorac Cardiovasc Surg 2000;120:999-1005.
- Han M, Trotta P, Coleman C, Linask KK. MCT-4, A511/Basigin and EF5 expression patterns during early chick cardiomyogenesis indicate cardiac cell differentiation occurs in a hypoxic environment. Dev Dyn 2006;235:124-31.
- Dobson KR, Reading L, Haberey M, Marine X, Scutt A. Centrifugal isolation of bone marrow from bone: an improved method for the recovery and quantitation of bone marrow osteopro-genitor cells from rat tibiae and femurae. Calcif Tissue Int 1999;65:411-3.
- Makino S, Fukuda K, Miyoshi S, Konishi F, Kodama H, Pan J, et al. Cardiomyocytes can be generated from marrow stromal cells in vitro. J Clin Invest 1999;103:697-705.
- Simpson PC, Savion S. Differentiation of rat myocytes in single cell culture with and without proliferating non myocardial myocytes: hypoxic cross striation, ultrastructure, and chronotropic response to catecholamines. Circ Res 1982;50:101-6.
- Negoro S, Kunisada K, Fujio Y, Funamoto M, Darville MI, Eizirik DL, et al. Activation of signal transducer and activator of transcription 3 protects cardiomyocytes from hypoxia/reoxygena-tion-induced oxidative stress through the upregulation of manganese superoxide dismutase. Circulation 2001;104:979-81.
- Xu FF, Liu XH, Cai LR. Role of hypoxia-inducible factor-1alpha in the prevention of cardiomyocyte injury induced by hypoxic preconditioning. Acta Physiol Sin 2004;56:609-14.
- Wakitani S, Saito T, Caplan AI. Myogenic cells derived from rat bone marrow mesenchymal stem cells exposed to 5-azacytidine. Muscle Nerve 1995;18:1417-26.
- Fukuda K. Molecular characterization of regenerated cardio-myocytes derived from adult mesenchymal stem cells. Congenti Anom 2002;42:1-9.
- Yoon J, Min BG, Kim YH, Shim WJ, Ro YM, Lim DS. Differentiation, engraftment and functional effects of pre-treated mesenchymal stem cells in a rat myocardial infarct model. Acta Cardiol 2005;60:277-84.
- Xu M, Wani M, Dai YS, Wang J, Yan M, Ayub A, et al. Differentiation of bone marrow stromal cells into the cardiac phenotype requires intercellular communication with myocytes. Circulation 2004;110:2658-65.
- Yuan Y, Chen LF, Zhang SY, Wu W, Chen H, Yan XW. Differentiation of mesenchymal stem cells into cardiomyogenic cells under the induction of myocardial cell lysate. Zhonghua Xin Xue Guan Bing Za Zhi 2005;33:170-3.
- Rodriguez-Sinovas A, Garcia-Dorado D, Pina P, Ruiz-Meana M, Soler-Soler J. Effect of sarcolemmal rupture on myocardial electrical impedance during oxygen deprivation. Am J Physiol Heart Circ Physiol 2005;288:H1396-403.
- Ito H, Adachi S, Tamamori M, Fujisaki H, Tanaka M, Lin M, et al. Mild hypoxia induces hypertrophy of cultured neonatal rat cardiomyocytes: a possible endogenous endothelin-1-mediated mechanism. J Mol Cell Cardiol 1996;28:1271-7.
- Shen JG, Quo XS, Jiang B, Li M, Xin W, Zhao BL. Chinonin, a novel drug against cardiomyocyte apoptosis induced by hypoxia and reoxygenation. Biochim Biophys Acta 2000;1500:217-26.
- Kacimi R, Long CS, Koudssi F, Karliner JS. Expression and regulation of adhesion molecules in cardiac cells by cytokines: Response to acute hypoxia. Circ Res 1998;82:576-86.
- Linask KK. Regulation of heart morphology: current molecular and cellular perspectives on the coordinated emergence of cardiac form and function. Birth Defects Res C Embryo Today 2003;69:14-24.
- Seko Y, Fujimura T, Taka H, Mineki R, Murayama K, Nagai R. Hypoxia followed by reoxygenation induces secretion of cyclophilin A from cultured rat cardiac myocytes. Biochem Biophys Res Commun 2004;317:162-8.
- Germack R, Dickenson JM. Adenosine triggers preconditioning through MEK/ERK1/2 signalling pathway during hypoxia/reoxygenation in neonatal rat cardiomyocytes. J Mol Cell Cardiol 2005;39:429-42.