Comparative studies of huperzine A, donepezil, and rivastigmine on brain acetylcholine, dopamine, norepinephrine, and 5-hydroxytryptamine levels in freely-moving rats1
Introduction
Alzheimer’s disease (AD) is a slowly progressive neurodegenerative illness, principally characterized by cognitive and behavioral abnormalities. Progressive neurodegeneration of cholinergic system underlies in part the cognitive deterioration of AD[7]. It has become increasingly evident that the dysfunction of the central monoaminergic system, that is, the dopaminergic, noradrenergic and serotonergic systems, accounts for the cognitive deficits associated with aging and AD[2,18,24,30]. Alterations in cholinergic function might well interact with those in monoaminergic system to produce additive or even synergistic effects on AD-related cognitive decline[11]. Thus, the restoration of cholinergic and monoaminergic neurotransmission may help to ameliorate impaired memory in AD patients. Accordingly, drug therapies with multiple molecular targets may be required in the treatment of memory dysfunction characteristic of AD[9]. With this rationale, many treatment strategies have focused on replacement therapy for deficits of central neurotransmission. Encouraging results have been obtained with cholinesterase inhibitors (ChEI) aiming to amplify the physiological action of ACh by preventing its hydrolysis in surviving nerve terminals of AD patients[14]. These include donepezil and rivastigmine, which have received US FDA approval. Huperzine A, a novel Lycopodium alkaloid isolated from the Chinese herb Huperzia serrata (H serrata), is a reversible, potent and selective ChEI which has widely been used in the treatment of AD in China[37]. All of these ChEI significantly reduce performance deficits in a variety of learning and memory tasks in AD patients[20,31,37]. On the basis of preclinical and clinical studies, one would predict that the clinical efficacy of ChEI would correlate best with increases in steady-state levels of ACh in the brain[15]. Moreover, previously published studies have indicated that ChEI affect extracellular DA and NE contents and/or turnovers in the brain of rats[7,16,36,39], suggesting that an increase of monoaminergic activity may be a common feature of these drugs when extracellular ACh metabolism is sufficiently inhibited. The positive clinical effect of ChEI has been related to stimulation of both the central cholinergic and monoaminergic systems[1]. Therefore, the nootropic effects of ChEI may involve monoaminergic as well as cholinergic mechanisms. Thus, measurements of extracellular ACh and monoamine concentrations in the central nervous system may be a reliable way to compare the potential efficacy of various ChEI.
The cognitive deficit in AD is most severe in the memory of recent events, that is, the working memory, whereas long-term declarative memory remains relatively intact. The cognitive decline of AD has been attributed to abnormalities in the cerebral cortex and the hippocampal formation[7]. Behavioral and neurochemical studies show that in both rats and humans, the prefrontal cortex (PFC) and hippocampus are critical for the working memory[23,25]. Drug-induced improvements in the working memory have been shown to parallel an increase in ACh efflux in the hippocampus[23] or PFC[29]. Dopaminergic transmission in the PFC and hippocampus is also important for working memory performance[13] and a loss of this transmission contributes to age-related cognitive decline[5]. Noradrenergic-cholinergic and serotonergic-cholinergic interactions at the cortical and hippocampal levels could also be of importance in the mediation of learning and memory processes[24,30]. Such findings raise the question of how about the therapeutic agents used in AD compared in their effects on neurotransmitter levels. We used dual-probe intracerebral microdialysis to examine extracellular ACh, DA, NE, and 5-HT in the mPFC and hippocampus of conscious rats treated with huperzine A, donepezil and rivastigmine.
Materials and methods
Drugs Natural (-)huperzine A (colorless powder with >98% purity) isolated from H serrata, was prepared by the Department of Phytochemistry in our institute. Donepezil hydrochloride and rivastigmine tartrate (both colorless powders with >98% purity) were prepared by the Department of Synthetic Chemistry in our institute. Huperzine A was dissolved in 0.1 mol/L HCl (final pH >4), while the other drugs were dissolved in H2O; all were then diluted with saline before administration, in a volume of 0.5 mL/kg.
Animals Male Sprague-Dawley rats (250–300 g) were supplied by the Shanghai Experimental Animal Center, Chinese Academy of Science (SPF, Certificate N
Surgery and microdialysis The rats were anesthetized with chloral hydrate (350 mg/kg, ip) and immobilized into a stereotaxic frame (SAS-4100, ASI). The scalp was opened to uncover the skull. Two guide cannulas (MD-2251, Bioanaly-tical Systems Inc, West Lafayette, IN, USA) were implanted in the left mPFC (coordinates relative to bregma, midline and skull surface: A 3.2 mm; L 0.3 mm; V 1.7 mm) and left ventral hippocampus (coordinates: A -5.8 mm; L 5.0 mm; V -3.6 mm) according to a standard atlas[27]. The guides were then secured to the skull by screws and dental cement. Following surgery, each animal was housed individually and allowed to recover for approximately 2 d before microdialysis.
All microdialysis experiments were carried out on conscious, freely-moving rats. On the day of the experiment, the dummy stylet in the guide cannula was replaced with a microdialysis probe (4 mm membrane-length, 320 µm outer diameter; MD-2200, BAS). The probe was perfused at 1 µL/min with artificial cerebrospinal fluid (146 mmol/L NaCl, 1.2 mmol/L CaCl2, 3 mmol/L KCl, 1.0 mmol/L MgCl2, 1.9 mmol/L Na2HPO4, 0.1 mmol/L NaH2PO4, pH 7.4) without any ChEI. After at least 90 min equilibration, dialysate samples were automatically collected (HoneyComb fraction collector, BAS) every 15 min into vials containing 1 µL acetic acid (0.1 mol/L) to prevent oxidation of monoamines. For monoamine determinations, the samples were split into equal volumes in order to analyze catecholamines and 5-HT separately. All samples were analyzed immediately or kept frozen at -80 °C until analysis. As a self-control for administration, animals were pretreated with saline after 3 baseline sample collections. Test drugs were administered (oral administration or ip injection) 45 min after the saline-treatment. The position of the probe was verified by visual inspection at the end of each experiment.
Drug administration In acute treatments, different ChEIs were administered (po or ip) during the in vivo microdialysis. In chronic treatments, oral huperzine A (0.5 µmol/kg) was administered once daily for 30 consecutive days, and surgical procedures were carried out on d 28. During the micro-dialysis, a final dose of huperzine A was given after 3 baseline sample collections.
ACh assay The microbore high performance liquid chromatography with electrochemical detection (HPLC-ECD) system consisted of a Waters 515 pump, BAS LC-26C degassing system, a Rheodyne 9125 injector (Rohnert Park, CA, USA) with 10 µL sample loop, and a BAS LC-4C ampero-metric detector equipped with a BAS UniJet radial-flow cell. A microbore ACh/choline enzyme reactor (50 mm×1 mm id; MF-8903, BAS) preloaded with AChE and choline oxidase enzymes was placed between the microbore analytical column (530 mm×1 mm id; MF-8904, BAS) and the detector, a UniJet glassy carbon electrode (3 mm, MF-1003, BAS), was prepared with peroxidase-redox polymer solution (CF-1070, BAS) and operated at 0 mV vs Ag/AgCl reference electrode. The mobile phase contained 50 mmol/L Na2HPO4, 10 mmol/L NaCl and 0.005% ProClin (CF2150, BAS) at pH 8.50. The flow rate was set at 130 µL/min. The detection limit for ACh was 15 fmol/10 µL injection.
Monoamine assay Monoamine concentrations were measured on another microbore HPLC-ECD system: PM-92e pump (BAS), BAS LC-26C degassing system, Rheodyne 9125 injector with 5 μL sample loop, BAS LC-22C temperature controllers; and BAS LC-4C amperometric detector equipped with a BAS UniJet radial-flow cell. Catecholamine or 5-HT was separated on a microbore ODS column (100 mm×1 mm id; MF-8949, BAS) at 35 °C. A UniJet glassy carbon electrode (3 mm, MF-1003, BAS) was used for the detection of catecholamines or 5-HT at 550 mV vs Ag/AgCl. The mobile phase for DA and NE separations consisted of 0.1 mol/L NaH2PO4, 0.5 mmol/L EDTA, 1 mmol/L sodium octyl sulfate, pH 3.1 and 6% acetonitrile, and was pumped at 70 µL/min. For the determination of 5-HT, the mobile phase, at a flow rate of 100 µL/min, was 25 mmol/L NaH2PO4, 50 mmol/L sodium citrate, 27 mmol/L EDTA-Na2, 10 mmol/L diethylamine-HCl, 10 mmol/L NaCl, 2 mmol/L sodium decane-sulfonic acid, pH 3.2 and acetonitrile 17.5%. The respective detection limits for DA, NE and 5-HT were 0.65 fmol, 0.59 fmol and 1.13 fmol per 5 µL injection.
Determination of acetylcholinesterase activity For AChE assay, rats were sacrificed by decapitation after receiving ip saline, huperzine A (0.5 µmol/kg), donepezil (4 µmol/kg) or rivastigmine (1 µmol/kg) at 0 min, 30 min, 1 h, 2 h, 3 h, and 4 h time points. As a rule, these animals had not been subjected to microdialysis. Cerebral PFC from the left hemisphere was dissected on ice and homogenized in 50 (w/v) volumes of ice-cold sodium phosphate buffer (75 mmol/L, pH 7.0). AChE activity in the homogenates was assayed by a modification of the standard spectrophotometric method[12] after 5 min preincubation with 4 mmol/L tetraisopropyl pyrophos-phoramide, a selective inhibitor of butyrylcholinesterase. Reactions were then carried out in duplicate for 8 min at 37 °C in a final volume of 4 mL containing 0.1 mL homogenate, 0.3 mmol/L acetylthiocholine iodide, and 25 mmol/L sodium phosphate buffer, pH 7.4. Reactions were terminated by adding 1 mL each of 3% sodium dodecyl sulfate and 0.2% 5,5'-dithio-bis(2-nitrobenzoic) acid to produce yellow 5-thio-2-nitro-benzoic anion, which was measured spectrophotometrically at 440 nm. Protein concentration was measured by the Coomassie brilliant blue protein-binding method using bovine serum albumin as standard[4]. AChE activity was converted to percentage of inhibition by comparison with mean values obtained from the saline-treated rats.
Statistical analysis For the analysis of neurotransmitter concentration, all results (mean±SEM) were expressed as percentage increases over the baseline. Paired-sample t-tests were used to assess the difference between baseline and postinjection values of neurotransmitters within the same treatment group. Independent-sample t-tests were used to compare the levels of AChE inhibition in the drug-treated groups. Comparison of the area under the curve (AUC fmol·h), integrated by neurotransmitter concentration between the different treatment groups, was performed by independent-sample t-tests or one-way analysis of variance followed by Tukey’s multiple test. In addition, two-way analysis of variance with repeated measures was used to compare means at various times between the different treatment groups. Post hoc comparisons were performed with independent-sample t-tests. A P value of 0.05 or less was considered significant.
Results
Effect of saline on levels of neurotransmitters Administration of saline during microdialysis produced no significant change in the levels of ACh, DA, NE, or 5-HT collected from the mPFC and ventral hippocampus in any tested rat.
Effects of huperzine A, donepezil and rivastigmine on extracellular ACh level Oral administration of huperzine A caused a dose-dependent increase in ACh levels in the mPFC and ventral hippocampus in the rats. Huperzine A produced maximal increases of 115%, 182%, and 307% in the mPFC and 125%, 158%, and 294% in the ventral hippocampus at doses of 0.25, 0.50, and 0.75 μmol/kg, respectively (Figure 1A,1B). The increases reached their highest level within 45–75 min and lasted about 6 h after the 0.75 µmol/kg dose. The magnitude and duration of ACh increase in the mPFC were similar to those in the ventral hippocampus after equimolar doses of huperzine A. The maximal increases of ACh after injecting 0.50 µmol/kg, ip (159% in the mPFC and 103% in the ventral hippocampus, respectively) were similar to those observed after oral administration of the same huperzine A dose (Figure 2A, 2B).
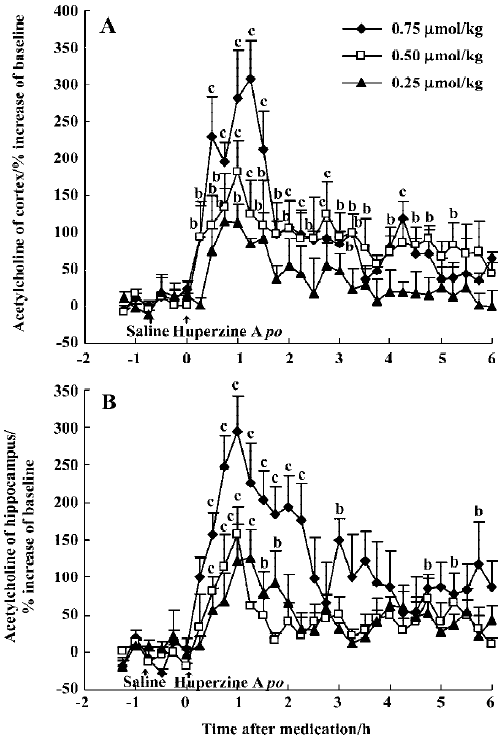
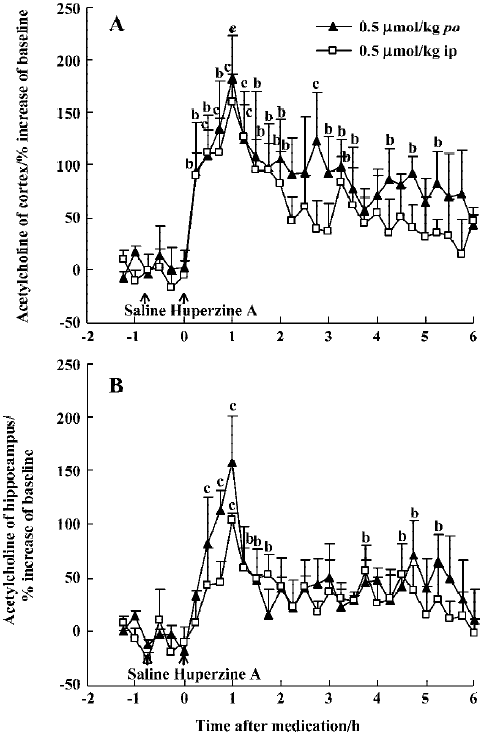
The effect of oral huperzine A at 0.50 µmol/kg was matched by oral donepezil at 45 min (211% in the mPFC and 215% in the ventral hippocampus), but this required a dose that was 11-fold higher (5.4 µmol/kg), and the effects lasted only 4 h (Figure 3A, 3B). Like huperzine A, donepezil was also effective after ip injection with maximal ACh increases of 200% in the mPFC and 165% in the ventral hippocampus, 30–60 min after the 4 μmol/kg dose (Figure 3A, 3B).
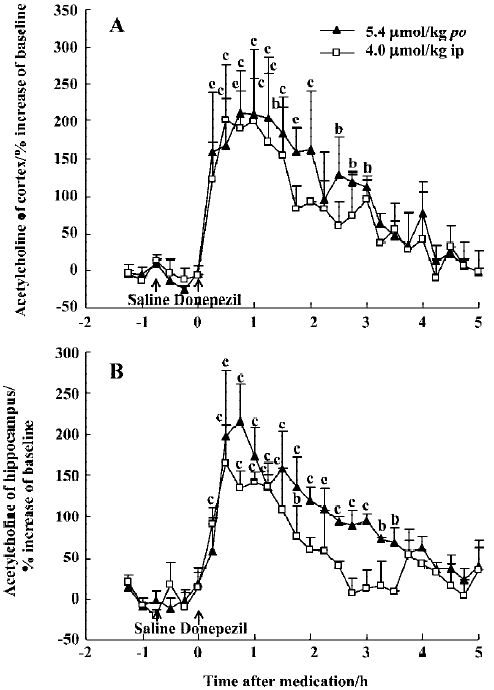
The potency of oral rivastigmine at a dose of 1 µmol/kg was similar to huperzine A (0.5 µmol/kg, po), with maximal ACh increases at 30–60 min of 148% in the mPFC and 166% in the ventral hippocampus, respectively (Figure 4A, 4B). The effects of rivastigmine, however, lasted only about 2 h. As with huperzine A, ip and oral administration were equi-effective at the same dose (Figure 4A, 4B).
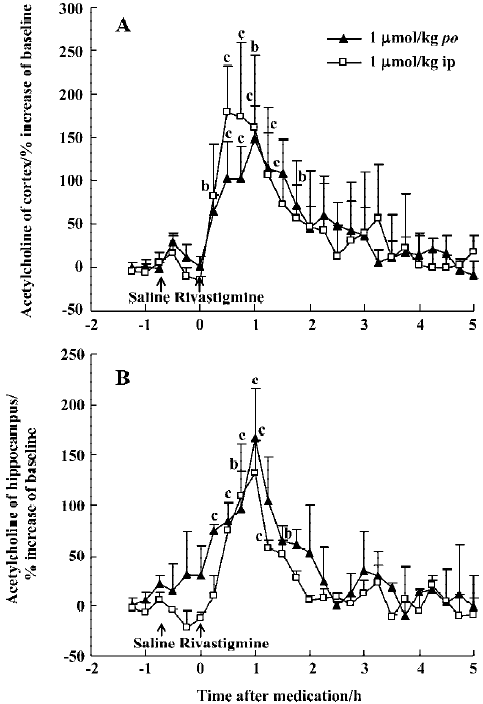
The time courses of huperzine A, donepezil, and rivastig-mine in equi-effective oral doses are shown in Figure 5. The difference between each of the drug-treated groups was significant [F(2,357)=10.73, P<0.01]. Comparison of the effects between oral huperzine A and donepezil or rivastigmine showed significant differences after 4.5 h. The AUC integrated by ACh concentrations after huperzine A was greater than that after donepezil (P>0.05) and rivastig-mine (P<0.05); oral huperzine A showed a longer lasting effect.
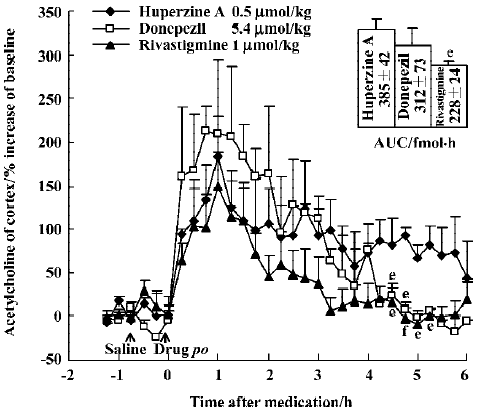
The peak increase of ACh levels in the mPFC coincided with the peak inhibition of AChE after ip injection of huperzine A (0.5 µmol/kg), donepezil (4 µmol/kg) and rivastigmine (1 µmol/kg). Doses of huperzine A and donepezil that caused similar increases of ACh levels also caused similar degrees of AChE inhibition (Figure 6). Rivastigmine, however, caused at least 3 times more AChE inhibition than the other 2 drugs, at comparable levels of ACh increase.
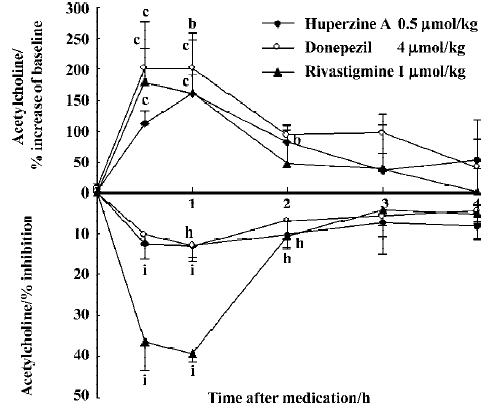
No cholinergic side effect was observed for any tested dosage of huperzine A or rivastigmine. However, the effects of donepezil (5.4 µmol/kg, po or 4 µmol/kg, ip) were accompanied by appreciable cholinergic signs, mainly muscle fasciculation.
Effects of huperzine A, donepezil and rivastigmine on extracellular monoamine levels Huperzine A (0.50 µmol/kg, po) significantly increased DA levels by 112% in the mPFC and 89% in the ventral hippocampus at 30–45 min after administration, respectively, with the effect on the mPFC lasting 60 min (Figure 7A, 7B). Similar maximal increases of DA levels in the mPFC occurred at 45 min after oral administration of donepezil (5.4 µmol/kg, 92%) and rivastigmine (1 µmol/kg, 106%) (Figure 7A). However, neither donepezil (5.4 µmol/kg, po) nor rivastigmine (1 µmol/kg, po) induced consistent changes in DA levels in the ventral hippocampus (Figure 7B). Despite this, there was no significant difference between the different drug-treated groups [F(2,228)=0.79, P>0.05, in the mPFC and F(2,228)=0.26, P>0.05, in the ventral hippocampus).
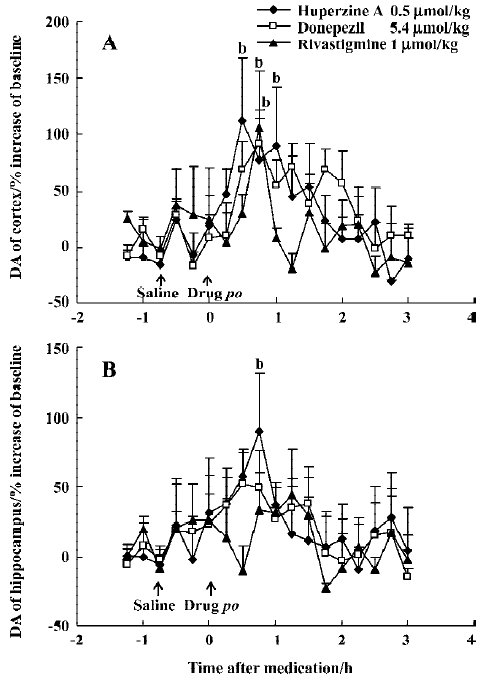
Huperzine A (0.50 µmol/kg, po) caused minor elevation of extracellular NE in the mPFC (42%) and ventral hippocampus (48%) in rats (Figure 8A, 8B), but with no statistical significance. A comparably weak and transient increase of NE levels in the mPFC (39%) was observed 30 min after oral donepezil administration (5.4 µmol/kg) (Figure 8A), while rivastigmine (1 µmol/kg, po) caused no effects at all (Figure 8A, 8B). Baseline levels of 5-HT were readily measurable in the mPFC (1.8±0.2 nmol/L) and ventral hippocampus (1.3±0.1 nmol/L). This transmitter, however, was even more resistant to change and did not respond to oral administration of huper-zine A (0.50 µmol/kg), donepezil (5.4 µmol/kg) or rivastigmine (1 µmol/kg) (data not shown).
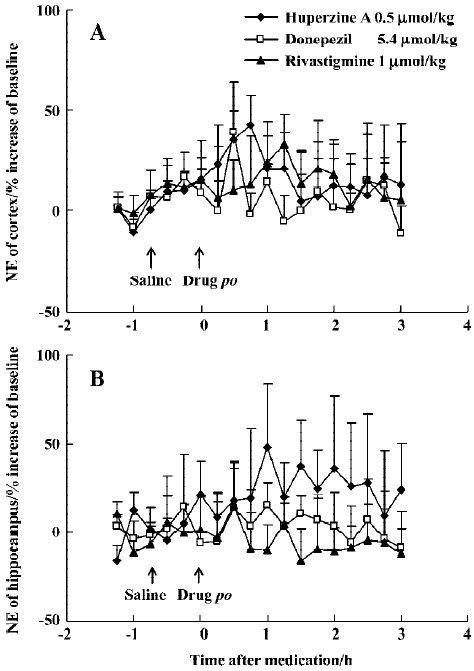
Effect of huperzine A on extracellular ACh levels in chronic experiment In order to determine if tolerance developed after repeat administration, 1 group of rats was subjected to daily oral doses of huperzine A for 30 consecutive days (0.5 µmol/kg). A final dose during microdialysis raised ACh levels over a period of 6 h, with maximal increases of 159% in the mPFC and 124% in the ventral hippocampus, respectively (Figure 9A, 9B). The AUC integrated by ACh concentrations in the mPFC or ventral hippocampus after administration showed no significant differences between single and multiple oral huperzine A. In fact, the responses to huperzine A in naïve and chronically-treated rats were not significantly different.
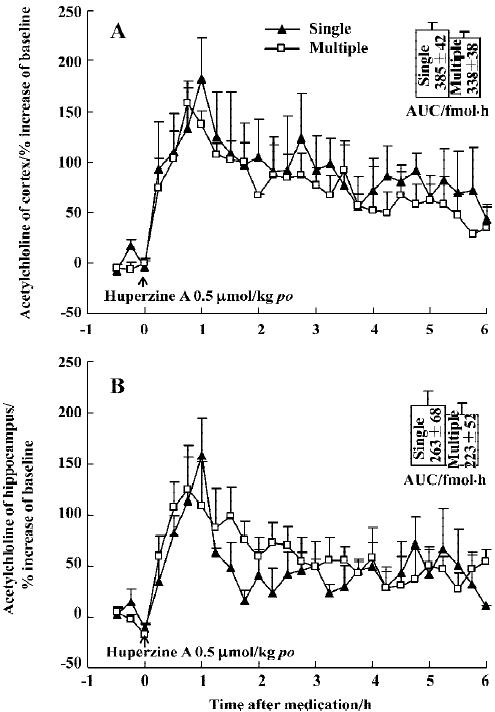
Discussion
The present study utilized a sensitive microdialysis system that detects femtomolar amounts of extracellular ACh with no need for ChEI in the probe itself to prevent ACh degradation. Our results provide the direct evidence that huperzine A enhances the levels of ACh and DA in the cortex and hippocampus. We also found that these enhancing effects were superior to those of donepezil and rivastigmine in equimolar doses. These results are consistent with previous studies, in which oral huperzine A was more effective than donepezil in alleviating working memory deficits induced by cholinergic lesions[37]. Thus, neurobehavioral efficacy appears to correlate with efficacy in elevating brain neurotransmitter levels.
In this study, huperzine A increased ACh levels in the mPFC and ventral hippocampus to a similar degree, while a previous study reported considerable regional variation[34]. Improved methodology may explain the discrepancy, since intracerebral microdialysis in freely-moving rats represents physiological conditions more closely than techniques based on post-mortem sampling.
The present results show that the increase of extracellular ACh following ChEI administration was mirrored by a decrease in brain AChE activity. However, in doses that caused the same enhancement of ACh levels, rivastigmine appeared to cause 3 times more AChE inhibition than the other 2 inhibitors. Kosasa et al[21] reported a similar differ-ence. In fact, the divergent outcomes are likely to reflect different modes of action at the active site of AChE. Tissue dilution during homogenization and assay will reduce the inhibition by a purely reversible ChEI such as huperzine A or donepezil. In contrast, dilution effects develop more slowly with rivastigmine, where enzyme recovery involves regeneration of a carbamylated active site[35]. Measurements of cholinesterase inhibition therefore underestimate the effects of reversible inhibitors[16]. In reality, the AChE inhibition by huperzine A or donepezil in vivo was probably greater than we measured ex vivo. Overall, extracellular ACh concentration measured by microdialysis is more reliable than AChE assays as an index of the functional activity of cholinergic systems in the brain and the response of these systems to the inhibitor.
Neuropharmacological findings in cognitive tasks document dopaminergic effects on cognitive performance[11]. The activity of cortical projections from the nucleus basalis is regulated in an excitatory manner by central dopaminergic neurons[10]. There is evidence that DA in the PFC contributes to information storage, particularly for the working memory[13]. Dopaminergic transmission in the hippocampus may likewise modulate spatial memory processing[32]. In light of these findings, it is not surprising that enhancement of dopaminergic function improves rodent performance in cognitive tests[19]. Therefore, the indirect effects of huperzine A on dopaminergic systems may constitute a significant advantage for patients with AD, a disorder in which the dopaminergic system is impaired along with cholinergic pathways.
In contrast with the effects on DA, huperzine A, donepezil and rivastigmine did not affect cortical or hippocampal NE and 5-HT levels. A previously published study showed that huperzine A induced an increased NE release at a high dose[39], whereas we failed to observe significant changes of NE level after a low dose of huperzine A. No effect on 5-HT by the 3 inhibitors matched the findings with the other tested ChEI[8]. Evidently, cholinergic systems exert much weaker effects on serotonergic activity than catecholaminergic activity in the cortex and hippocampus.
The key principle of indirect cholinomimetic therapy with ChEI is to reduce ACh breakdown in the central nervous system by inhibiting AChE. ChEI-induced increase in DA level may reflect increased subcortical actions of extracellular ACh[39]. The different effects of the 3 inhibitors on neurotransmitters may relate to differences in a number of features, including actions on AChE, rates of dissociation from the inhibited enzyme, rates of metabolism and rates of release of active compounds. Computer-assisted docking studies and X-ray crystallography have shown that binding to different sites and utilization of different inhibitory mechanisms appear to explain the greater selectivity of huperzine A for AChE compared with donepezil[26,28].
Rivastigmine is a pseudo-irreversible, carbamate-based ChEI[38], which behaves kinetically and structurally as a poor substrate for AChE[3]. Our findings are consistent with a previous report[21] that found that rivastigmine affected brain ACh concentration with greater potency than donepezil or tacrine, but more briefly. Such findings may reflect the different modes of action. However, the short time course of rivastigmine is contrary to expectations since regeneration of carbamylated enzyme is inherently slower than desorption of a reversible agent-like huperzine A and donepezil. Further research is needed to clarify the relative importance of enzyme regeneration and ChEI metabolism in determining the duration of ACh elevation.
It is well established that some ChEI elicit tolerance[6]. Previous results have shown that the response to certain agents, especially tacrine, diminishes considerably after a second injection. Selective down-regulation of muscarinic receptors reduces the efficacy of long-term treatment with tacrine in AD[17]. In contrast, the present results show no significant decay in the magnitude of ACh elevations after chronic treatment with huperzine A. These data are in accord with earlier results with regard to AChE inhibition[22] and the attenuation of scopolamine-induced amnesia[37]. The lack of tolerance to the therapeutic effect may be important. Combined with the effects on multiple neurotransmitter systems, the ability of huperzine A to continue evoking increases in cerebral ACh during chronic administration should be clinically advantageous for improving cognitive function in AD.
Acknowledgement
We thank Prof Stephen W BRIMIJOIN (Mayo Clinic, USA) for his critical comments and English revision on the manuscript.
References
- Alhainen K, Helkala EL, Reinikainen K, Riekkinen P Sr. The relationship of cerebrospinal fluid monoamine metabolites with clinical response to tetrahydroaminoacridine in patients with Alzheimer’s disease. J Neural Transm 1993;5:185-92.
- Barili P, De Carolis G, Zaccheo D, Amenta F. Sensitivity to ageing of the limbic dopaminergic system: a review. Mech Ageing Dev 1998;106:57-92.
- Bar-On P, Millard CB, Harel M, Dvir H, Enz A, Sussman JL, et al. Kinetic and structural studies on the interaction of cholinesterase with the anti-Alzheimer drug rivastigmine. Biochemistry 2002;41:3555-64.
- Bradford MM. A rapid and sensitive method for the quantitation of microgram quantities of protein utilizing the principle of protein-dye binding. Anal Biochem 1976;72:248-54.
- Brozoski T, Brown RM, Rosvold HE, Goldman PS. Cognitive deficit caused by regional depletion of dopamine in prefrontal cortex of rhesus monkey. Science 1979;205:929-32.
- Costa LG, Schwab BW, Murphy SD. Tolerance to anticholinesterase compounds in mammals. Toxicology 1982;25:79-97.
- Coyle JT, Price DL, DeLong MR. Alzheimer’s disease: a disorder of cortical cholinergic innervation. Science 1983;219:1184-90.
- Cuadra G, Summers K, Giacobini E. Cholinesterase inhibitor effects on neurotransmitters in rat cortex in vivo. J Pharmacal Exp Ther 1994;270:277-84.
- Davidson M, Bierer LM, Kaminsky R, Ryan TM, Davis KL. Combined administration of physostigmine and clonidine to patients with dementia of the Alzheimer type: a pilot safety study. Alzheimer Disease Assoc Disorders 1989;3:224-7.
- Day J, Fibiger HC. Dopaminergic regulation of cortical acetylcholine release. Synapse 1992;12:281-6.
- Decker MW, McGaugh JL. The role of interactions between the cholinergic system and other neuromodulatory systems in learning and memory. Synapse 1991;7:151-68.
- Ellman GL, Courtney KD, Andre V Jr, Featherstone RM. A new and rapid colorimetric determination of acetylcholinesterase activity. Biochem Pharmacol 1961;7:88-95.
- Floresco SB, Seamans JK, Phillips AG. Selective roles for hippocampal, refrontal cortical, and ventral striatal circuits in radial-arm maze tasks with or without a delay. J Neurosci 1997;17:1880-90.
- Giacobini E, Becker R. Development of drugs for Alzheimer therapy: a decade of progress. In: Giacobini E, Becker R, editors. Alzheimer Disease: Therapeutic Strategies. Boston, MA: Birkhäuser Press; 1994. p 1–7.
- Giacobini E, Cuadra G. Second and third generation cholinesterase inhibitors: from preclinical studies to clinical efficacy. In: Giacobini E, Becker R, editors. Alzheimer Disease: Therapeutic Strategies. Boston, MA: Birkhäuser Press; 1994. p 155–71.
- Giacobini E, Zhu XD, Williams E, Sherman KA. The effect of the selective reversible acetylcholinesterase inhibitor E2020 on extracellular acetylcholine and biogenic amine levels in rat cortex. Neuropharmacology 1996;35:205-11.
- Hallak M, Giacobini E. Physostigmine, tacrine and metrifonate: the effect of multiple doses on acetylcholine metabolism in rat brain. Neuropharmacology 1989;28:199-206.
- Haroutunian V, Santucci AC, Davis KL. Implications of multiple transmitter system lesions for cholinominetic therapy in Alzheimer’s disease. Prog Brain Res 1990;84:333-46.
- Hersi AI, Rowe W, Gaudreau P, Quirion R. Dopamine D1 receptor ligands modulate cognitive performance and hippocampal acetylcholine release in memory-impaired aged rats. Neuroscience 1995;69:1067-74.
- Jann MW. Rivastigmine, a new-generation cholinesterase inhibitor for the treatment of Alzheimer’s disease. Pharmacotherapy 2000;20:1-12.
- Kosasa T, Kuriya Y, Matsui K, Yamanishi Y. Effect of donepezil hydrochloride (E2020) on basal concentration of extracellular acetylcholine in the hippocampus of rats. Eur J Pharmacol 1999;380:101-7.
- Laganiere S, Corey J, Tang XC, Wulfert E, Hanin I. Acute and chronic studies with the anticholinesterase huperzine A: effect on central nervous system cholinergic parameters. Neuropharmacology 1991;30:763-9.
- Levy A, Kong RM, Stillman MJ, Shukitt-Hale B, Kadar T, Rauch TM, et al. Nimodipine improves spatial working memory and elevates hippocampal acetylcholine in young rats. Pharmacol Biochem Behav 1991;39:781-6.
- Marien MR, Colpaert FC, Rosenquist AC. Noradrenergic mechanisms in neurodegenerative diseases: a theory. Brain Res Rev 2004;45:38-78.
- McCarthy G, Blamire AM, Puce A. Functional magnetic resonance imaging of human prefrontal cortex activation during a spatial working memory task. Proc Natl Acad Sci USA 1994;91:8690-4.
- Pang YP, Kozikowski AP. Prediction of the binding site of huperzine A in acetylcholinesterase by docking studies. J Comput Aided Mol Des 1994;8:669-81.
- Paxinos G, Watson C, editors. The rat brain in stereotaxic coordinates. Sydney: Academic Press; 1997.
- Raves ML, Harel M, Pang YP, Silman I, Kozikowski AP, Sussman JL. Structure of acetylcholinesterase complexed with the nootropic alkaloid (-)-huperzine A. Natural Struct Biol 1997;4:57-63.
- Scali C, Casamenti F, Pazzagli M, Bartolini L, Pepeu G. Nerve growth factor increases extracellular acetylcholine levels in the parietal cortex and hippocampus of aged rats and restores object recognition. Neurosci Lett 1994;170:117-20.
- Steckler T, Sahgal A. The role of serotonergic-cholinergic interactions in the mediation of cognitive behaviour. Behav Brain Res 1995;67:165-99.
- Sugimoto H, Ogura H, Arai Y, Limura Y, Yamanishi Y. Research and development of donepezil hydrochloride, a new type of acetylcholinesterase inhibitor. Jpn J Pharmacol 2002;89:7-20.
- Taghzouti K, Le Moal M, Simon H. Dopaminergic innervation of the lateral septum and learning processes. Neurosci Lett Suppl 1986;26:s332.
- Tang XC, Huperzine A. Shuangyiping): A promising drug for Alzheimer’s disease. Acta Pharmacol Sin 1996;17:481-4.
- Tang XC, De Sarno P, Sugaya K, Giacobini E. Effect of huperzine A, a new cholinesterase inhibitor, on the central cholinergic system of the rat. J Neurosci Res 1989;24:276-85.
- Taylor P. Anticholinesterase agents. In: Hardman JG, Limbird LE, Molinoff PB, Ruddon RW, Gilman AG, editors. The pharmacological basis of therapeutics. New York: McGraw-Hill; 1996. p 161–76.
- Trabace L, Coluccia A, Gaetani S, Tattoli M, Cagiano R, Pietra C, et al. In vivo neurochemical effects of the acetylcholinesterase inhibitor ENA713 in rat hippocampus. Brain Res 2000;865:268-71.
- Wang R, Yan H, Tang XC. Progress in studies of huperzine A, a natural cholinesterase inhibitor from Chinese herbal medicine. Acta Pharmacol Sin 2006;27:1-26.
- Weinstock M, Razin M, Chorev M, Enz A. Pharmacological evaluation of phenyl-carbamates as CNS-selective acetylcholinesterase inhibitors. J Neural Transm 1994;43:219-25.
- Zhu XD, Giacobini E. Second generation cholinesterase inhibitors: effect of (L)-huperzine A on cortical biogenic amines. J Neurosci Res 1995;41:828-35.