Cloning, expression, and characterization of pollen allergens from Humulus scandens (Lour) Merr and Ambrosia artemisiifolia L1
Introduction
Accurate allergy diagnosis and efficient immunotherapy protocols are strongly dependent on the use of standardized extracts or well-characterized allergen mixtures[1-3]. However, standardization of specific allergens from natural allergen extracts is a huge task[4] and it is almost impossible to carry out standardization on a large scale for day-to-day clinical practice. Recombinant allergens that have similar structures, functions and immunoreactivity to their native counterparts could be used to overcome this problem, given that they can be produced in large quantities, leading to more specific, effective and safer immunotherapy treatment[5].
Currently, gene cloning for novel allergens is mainly based on the construction of a cDNA library, which is time-consuming and expensive[6-9]. Other methods for isolating unknown allergens involve using the polymerase chain reaction (PCR) with either degenerate primers consisting of a pool of primers containing most or all of the possible nucleotide sequences encoding a conserved amino acid motif[10], or consensus primers consisting of a single primer containing the most common nucleotide at each codon position within the motif[11]. Although these strategies have been successful in isolating closely related sequences, they have generally failed when sequences are more distantly related[11]. Many programs and algorithms have been developed for sequence comparisons of multiple members of protein families[12-15]. By using alignment techniques, the first amino acid sequence that emerges, that is, the sequence aligned uppermost in each large and dense cluster in the output tree produced, has provided the most information about amino acid diversity in the cluster. In the current study, we refer to these sequences as "core sequences", and we designed the degenerate primers based on the core sequences and their cognates. Under special PCR conditions, we expected that the degenerate primers could match all associated sequences in the abovementioned cluster, and thus could be used for cloning new allergen gene members in a distantly related species. We will hereafter refer to these primers as "pan-degenerate primers". We expected that the process involving the pan-degenerate primers would be more efficient and less expensive than the cDNA library technique, and would have a higher success rate than an ordinary PCR method.
Humulus scandens (Lour) Merr (Moraceae: Cannaboideae; Lücao in Chinese) is a weed that grows in most provinces of China. The plant blossoms in summer and bears a large quantity of pollen. Short ragweed (Ambrosia artemisiifolia L; Compositae) is one of the most invasive weeds in the world. It was introduced to China in 1930s, and is now distributed from the Yantze River to the 3 northeastern provinces of China. Nanning, Wuhan, Nanchang-Jiujiang and Shenyang-Tieling-Dandong are the 4 areas in which the weed is most pervasive in China. Pollen from H scandens and A artemisiifolia have become the main source of pollen allergens in this country. However, little is known of the actual allergens in the pollen of the weeds. In this study, we report on the cloning, expression and characterization of 6 pollen allergen genes from the 2 weeds. Following the non-fusion expression and purification of the 5 encoded proteins, 2 representative proteins of the 5, provisionally named rAmb a 8(D03) and rHum s 1(LCM9), were immunologically characterized in detail.
Materials and methods
Sample preparations Wild plants of H scandens and A artemisiifolia were harvested in Wuhan. Flowers were bagged, and the pollen collected from each plant was mixed with other pollen collected from the same plant. All pollen collected was immediately submerged in liquid nitrogen until use. Twenty-five patients with A artemisiifolia pollen allergy and 10 patients with H scandens pollen allergy (as assessed by a positive skin prick test reaction to A artemisi-ifolia and a serum level of A artemisiifolia/H scandens specific IgE higher than 3.5 kUA/L) and 13 healthy volunteers were recruited. Blood samples were taken from all participants in the Allergy Clinic, Shenyang North Hospital. All the samples were tested by using the UniCAP Specific IgE Fluoroenzymeimmunoassay (Pharmacia Diagnostics AB, Uppsala, Sweden). After centrifugation at 500×g, 4°C, for 10 min, serum from each individual was collected and stored at -70°C until use. The study was approved by the Ethics Committee of Shenyang North Hospital, Shenyang, China, and written informed consent was obtained from all participants.
Primer design The 478 pollen allergens and their related sequences were retrieved by keyword searches in the SWISS-PROT and TrEMBL websites (http://www.expasy.org) and were multiple-aligned online by using Clustal W software[16]. For alignment, the default values were used to obtain the output tree in a phylogram pattern, with the absolute distances labeled. The uppermost allergen sequence in each large and dense cluster in the output tree was taken as the core sequence. There were several of these core sequences found in the output phylogenetic trees. Based on the core sequences, allergen sequences with high homology (with BLAST E values ≤10-10) were selected for further analysis to acquire conservative gene domains. Pan-degenerate primers, which we expected to be successful in all species, were thus designed according to cDNA sequences of the conservative domains chosen.
RT-PCR analysis Frozen pollen samples were ground in a mortar filled with liquid nitrogen, and the total RNA was extracted by using an RNeasy Maxi Kit (Qiagen Valencia, CA, USA) according to the manufacturer's instructions. After quality and concentration assessments, the RNA was either immediately used for RT-PCR analysis or stored at -80 ºC until use.
After reverse transcription (2 µg total RNA per sample) was performed by using the ProtoScript First Strand cDNA Synthesis Kit (New England Biolabs, Beverly, MA, USA) according to the manufacturer's instructions, PCR was carried out by using 50 ng of the reverse transcription product in 25 ΜL of the reaction mixture containing 1.25 U HotstarTaq DNA polymerase (Qiagen), 1×PCR buffer (Qiagen), 0.2 mmol/L dNTP, and 0.4 µmol/L of each primer. Thermal cycling was performed in a PTC-200 Peltier Thermal Cycler (MJ Research, Watertown, MA, USA) as follows: after an initial step at 95°C for 15 min to activate the DNA polymerase, 9 touchdown cycles were carried out at 94°C for 45 s, 68°C for 30 s and 72°C for 30 s, with the annealing temperature decreasing by 1°C per cycle. This was followed by 26 gradient cycles at 94°C (45 s)→55°C →61°C (30 s)→72°C (30 s). After purification with a QIAquick PCR Purification Kit (Qiagen), the PCR products were cloned into the pGEM-T easy vector (Promega, Madison, WI, USA). The inserted plasmids were transformed into JM109 (Promega). Positive clones were confirmed first by colony PCR with the former PCR primers, and then by sequencing. DNA sequencing was carried out by the BioAsia Biotechnology Co (Shanghai, China) by using the dideoxy chain-terminating method.
Full-length cDNA synthesis and sequencing The clones derived from the degenerate primers did not cover the full-length coding region. Gene-specific primers were therefore synthesized (Table 1), and combined using the RACE process[17] with RA3, a 3' reverse primer from the GeneRacer Kit (Invitrogen Corporation, Carlsbad, CA, USA). The PCR conditions were similar to those for RT-PCR, with a slight modification in the denaturing temperature and extension time. PCR products were also cloned into the pGEM-T easy vector, and positive clones were confirmed by colony PCR and sequencing. Full-length cDNAs, acquired by deduction of the truncated gene and its 3' end, were translated into amino acid sequences, with their isoelectric point and molecular weight calculated (http://www.expasy.org). cDNA and protein homologues, retrieved by using the BLAST program in the NCBI website (http://www.ncbi.nlm.nih.gov/), were aligned by using the CLUSTAL W (1.82) program[16]. Phylogenetic trees were subsequently generated using MEGA2 software[18].
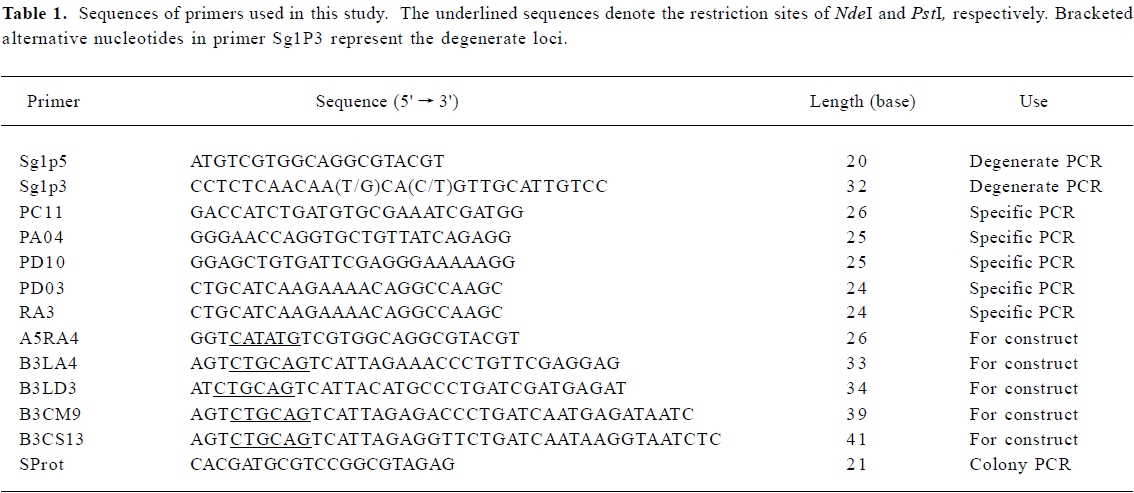
Full table
Northern blot Northern blots were performed according to the membrane manufacturer's instructions. Briefly, a total of 20 μg RNA was loaded on to a formaldehyde-containing gel. After running the gels at 40 V for 3 h, the RNA bands were electrically transferred onto Nytran SuPerCharge nylon transfer membrane (Schleicher & Schull BioScience, New Hampshire, USA). After UV cross-linking treatment, the membrane was stained with methylene blue [0.02% [w/v] methylene blue in 0.3 mol/L sodium acetate(pH 5.5)] to investigate the transferring efficiency. Following destaining, BrightStar Psoralen-Biotin (Ambion Inc, Autin, TX, USA)-labeled probe D03 was applied for hybridization in ULTRhyb hybridization buffer (Ambion Inc). The membrane was washed twice with a buffer containing 2? SSC (0.3 mol/L NaCl, 0.03 mol/L sodium citrate) and 0.1% sodium dodecylsulfate before the probes were detected by using the Phototope Star Detection Kit (New England Biolabs). Finally, the filter was exposed to Fuji Medical X-ray film at room temperature for 5?8 min.
Protein expression and immunoblotting To enable non-fusion protein expression, the S-protein tag and His-tag were removed from the pET-44 vector and the allergen-coding regions were decorated by introducing restriction sites (5' NdeI and 3' PstI) by PCR using the corresponding primers (Table 1). Subsequent to double restriction digestion and purification, the PCR products were ligated with the pET-44 EK/LIC vector (Novagen, Madison, WI, USA). After transformation of the ligation products into NovaBlue Singles cells (Novagen), positive clones and in-frame insertions were confirmed, initially by colony PCR with the primer SProt and 3' primers related to each clone, and then by sequencing with the S-Tag 18-mer primer or ColiDown primer (Novagen). Plasmid DNA was extracted with the QIAprep Miniprep Kit (Qiagen), and transformed into E coli RosettaBlue (DE3) cells (Novagen) for expression, following the manufacturer's instructions. Cells were grown in 1 L Luria-Bertani (LB)-medium until the OD600 value reached 0.6. Target protein expression was induced by the addition of isopropyl-D-thio-galactopyranoside (IPTG) to 1 mmol/L for 4 h at 30°C before harvest. Finally, the recombinant proteins were purified by affinity chromatography using poly-(L)-proline as the solid phase. The bacterium lysate was applied to the column, followed by washing with 1 mol/L urea and elution with 6 mol/L urea. The elution product was dialyzed stepwise against decreasing amounts of urea (6, 4, 2 mol/L, no urea) in phosphate-buffered saline (PBS; 1.8 mmol/L potassium phosphate, pH 7.4, 0.137 mol/L NaCl) and then dialyzed against double-distilled water, followed by freeze-drying.
Purified rAmb a 8(D03) and rHum s(LCM9) were selected as representatives for immunoblotting. Fifteen micrograms of protein were loaded onto the 15% SDS-PAGE gel, and were then electrically transferred to a polyvinylidene difluoride (PVDF) membrane (Amersco, Solon, OH, USA). Following blocking the membrane overnight with a 4% bovine serum albumin solution at 4°C, allergic sera (diluted 1:7) were added to each membrane strip for 2 h before the addition of peroxidase conjugated goat anti-human IgE (Sigma-Aldrich, Saint Louis, MO, USA). The blotted band was finally visualized with a diaminobenzidine (DAB) chromogenic substrate solution (Amersco).
N-terminal end sequencing Sequencing of the N-terminal end of recombinant proteins was performed by using the Applied Biosystems Procise 491 Sequencer from Applied Biosystems, according to the manufacturer's instructions.
Results
Homology between different allergen genes The 478 pollen allergens obtained from the SWISS-PROT and TrEMBL websites were clustered into 8 large groups, which consisted of a number of subgroups. Further searching with the core sequences from each subgroup demonstrated that these core sequences matched a large quantity of closely related homologues. For instance, Q9XF40, the first core sequence in group 8, was an apple pollen allergen with extensive homology with other pollen allergens from different species (Figure 1), such as Poa pratensis, Lolium perenne, Dactylis glomerata, Hordeum vulgare, Holcus lanatus (e values <2e-25). The conservative domains in the Q9XF40 cDNA sequence (1?20 bp, 343?369 bp) as resolved by multiple sequence alignment of Q9XF40 and its cognates were taken as the Sg1p5/Sg1p3 primer sequence (Table 1). Other degenerate primers were designed following the same principle.
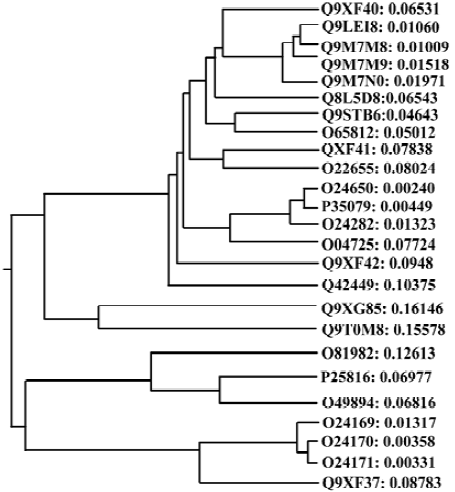
Full-length cDNA generation By combining the gradient PCR with touchdown PCR, 3 gene fragments were obtained from each weed species, and were subsequently inserted into the pGEM-T vector. Sequence analysis showed that the inserts, 5 of 363 bp and 1 of 369 bp, were homologous to the known allergen gene profilin from different species with up to 90% (5 clones except for D03) and 79% (for D03) identity, but with ~30 bp truncated at the 3' ends, suggesting a further requirement of the RACE process for the full-length cDNA. After cloning of the amplificants from the specific PCR with primer pairs PC11/RA3, PM9/RA3, PD10/RA3 and PD03/RA3, a total of 29 positive RACE clones were detected. Subsequent sequencing reactions demonstrated that they all contained the initiation and stop codons, primer sequences and poly (A) tails, reflecting the mRNA source of the clones. Northern blotting with a clone D03 probe further confirmed that the cDNA cloned was derived from the pollen mRNA (Figure 2). Bridging the 3' end and the corresponding fragment genes produced 6 novel full-length coding genes, among which LCS13, LCM20 and LCM9 were from H scandens, whereas A0418, D106 and D03 were from A artemisiifolia. They are all available in GenBank under accession Nos AY268422-AY268427.
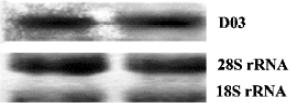
Sequence analysis Sequence analysis revealed that there were several altered nucleotides in the cDNA sequences compared with the degenerate primers used in the fragment-gene cloning process (data not shown), which means that the degeneracy was effectively expanded. The predicted amino acid sequences of the 6 genes were acidic proteins (pI of approximately 5.0) with molecular weights of approximately 14 kDa. Multiple sequence alignment demonstrated that these 6 novel sequences shared a high homology, up to 83%. Moreover, cDNA and protein sequence searches with Blastn and Blastx programs in GenBank showed that the new genes were homologous to profilin genes from more than 10 plant families including Rosaceae, Euphorbiaceae, Phaseo-leae, Liliaceae, Poaceae, Urticaceae, Apiaceae, Oleaceae, Sola-naceae, Brassicaceae, and Compositae. Alignment of the cognates derived from this search produced a phylogenetic tree, which showed that the 6 novel genes cloned were clearly separated into 3 subgroups, but with high homology (Figure 3).
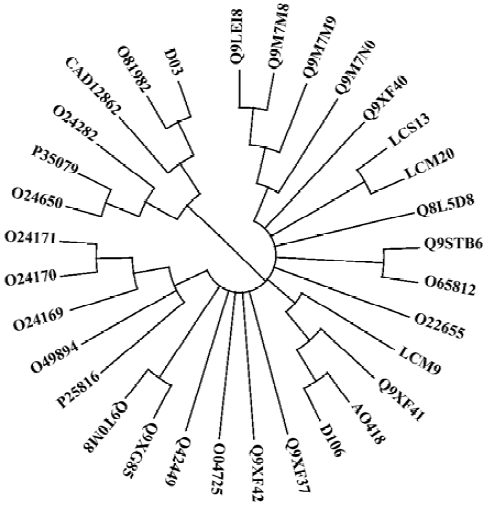
Of the 6 clones, A0418, D106 and LCM9, 3 closely related clones that encoded 131-amino acid residues, exhibited high homology with apple pollen allergen Q9XF41 (Mal d 4; GenBank accession number: AF129427). LCS13 and LCM20, 2 clones from H scandens, had only differences in the cDNA non-coding regions and therefore encoded the same protein, which had 80% and 85% identity with Q8L5D8 from date palm pollen and Q9XF40 from apple pollen, respectively. Clone D03 encoded 133 amino acid residues, and had an identity of 79% and 72% with O81982 (Hela 2)[19] and CAD12862[20], 2 profilin proteins from common sunflower and mugwort. Hel a 2 and D03, along with the timothy grass profilins O24282, P35079, O24650, were clustered into one subgroup (Figure 3). Furthermore, D03 was quite different from the other 5 clones in 4 regions of its amino acid sequence, which represents an approximately 90% difference between D03 and other profilins (Figure 4).
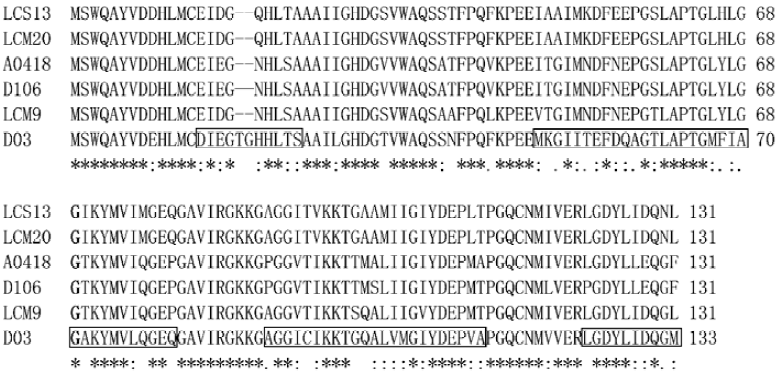
Protein expression and purification, and immunoblotting After detecting the correct reading frame of the target protein gene in the expression vector, the in-frame plasmids were then successfully transformed into E coli RosettaBlue (DE3) cells. Following induction and further incubation at 30°C for 6 h, the recombinant non-fusion proteins were visualized using SDS-PAGE, and had an apparent molecular mass of ~14 kDa (Figure 5A). The N-terminal sequence of 10 amino acids of rAmb a 8(D03) confirmed that the recombinant protein was the same as that deduced from the cDNA sequence. The yield of purified protein was approximately 8 mg per L of cultured bacteria.
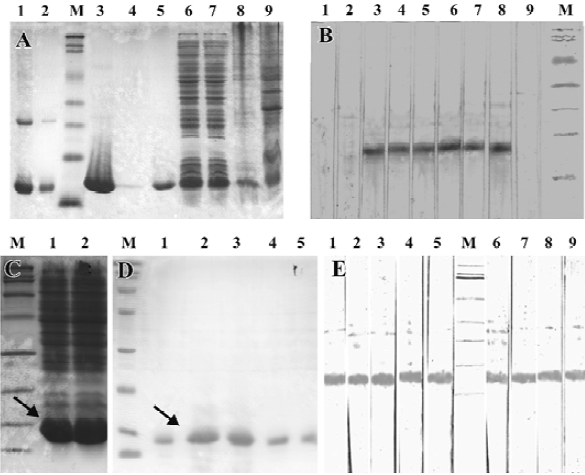
IgE antibodies from 6 of 8 sera (75%) from the A artemisi-ifolia allergic patients reacted with recombinant protein rAmb a 8(D03) in the immunoblot experiments. The bands occurred at approximately 14 kDa (Figure 5B). Other recombinant proteins were also very efficiently expressed and purified in non-fusion form (Figure 5C, 5D). As a representative from H scandens, the immunoblotting profile of rHum s 1(LCM9) is shown in Figure 5E. Interestingly, rHum s 1(LCM9) not only reacted with the sera from 5 patients with H scandens allergy, but also reacted with sera from patients with A artemisiifolia allergy, which suggests that cross-reactivity occurred for rAmb a 8(D03) and rHum s 1(LCM9).
Discussion
In the past, most allergen genes have been cloned by cDNA library screening[7-9], and hundreds of pollen allergen genes from more than 170 species are available in online databases, which can be sorted into several groups. This means that homologous genes exist in many related or unrelated species, which provided support for the current method. In this study, we designed pan-degenerate primers and succeeded in cloning pollen allergen cDNAs from different weeds, thus showing that there is a way to clone homologous genes from distantly related species or little-studied species. Furthermore, by using a combination of the gradient PCR process and touchdown steps, the genes with some divergence in their primer regions could be obtained in the weed genome; that is, the degeneracy of the primers was expanded. The method could therefore be used to clone the same allergens in other species or to clone other kinds of allergen genes.
It has been argued that allergenicity cannot be rationalized on the basis of either overall folding pattern or biological function, and thus any protein should be regarded as a potential allergen[19]. According to alignment studies, the proteins coded by our new clones had more than 60% identity over the full-length peptide with the known allergens, suggesting that the proteins would be allergenic. Thus, D03 and LCM9, the most different variants of the 6 clones obtained, were expressed as recombinant proteins. Through efficient expression in a pET-44 system, ideal solubility of all the recombinant proteins was obtained, which facilitated protein purification through affinity chromatography, and would ensure efficiency in commercial production.
The molecular basis of cross-reactivity has been of primary concern in numerous publications[19-21]. The relationship between sequence similarity, as obtained by pair-wise alignment, and structural or functional properties has been the goal of much research[22-24]. Recent studies have confirmed a widely accepted rule-of-thumb that 30% or 35% identity over aligned regions suffices for structural or functional deduction[25,26]. A remote homologue study[27] demonstrated that allergens from diverse sources have a common structural motif, namely a groove located inside an α-β motif, which could potentially serve as a ligand-binding site, thus leading to the stimulation of the T cell helper type 2 (Th2) response and a subsequent bias towards the synthesis of IgE. This result expanded cross-reactivity to between microbes and higher plants. In the present study, the clones were different from each other in their cDNA or protein sequence, suggesting open pollination and/or large genetic variation in the weeds. This was also consistent with other studies on ragweed and timothy grass sequence polymorphism[28,29]. However, multiple sequence analysis demonstrated that the newly cloned genes had up to 83% homology with each other and 60%?90% identity with previously described allergen profilins from latex, food, and pollens from various organisms, which can produce extensive cross-reactivity[30,31]. This result demonstrated that proteins from H scandens and A artemisiifolia would have cross-reactivity. In a previous study we also inferred that the proteins coded by clone D106 would have cross-reactive properties for food- and/or pollen-sensitive allergic individuals[32,33]. All of these results imply that the newly cloned genes would function the same way as pan-allergen profilins. Based on the issues described herein and the results obtained in the current study, we assume that cross-reactivity would occur so widely that atopic individuals would be surrounded by a dynamic network of allergens, and would be predisposed towards allergic diseases.
References
- van Ree R. Analytic aspects of the standardization of allergenic extracts. Allergy 1997;52:795-805.
- Durham SR, Walker SM, Eva-Maria V, Ikilar J. Long-term clinical efficacy of grass-pollen immuno-therapy. N Engl J Med 1999;341:468-75.
- van Hage-Hamsten M, Johansson E, Roquet A, Peterson C, Andersson M, Greiff L, et al. Nasal challenges with recombinant derivatives of the major birch pollen allergen. Clin Exp Allergy 2002;32:1448-53.
- Valenta R, Kraft D. Recombinant allergen molecules: tools to study effector cell activation. Immunol Rev 2001;179:119-27.
- Villalba M, Batanero E, Monsalve RI, Gonzalez de la Pena MA, Lahoz C, Rodriguez R. Cloning and expression of Ole e I, the major allergen from olive tree pollen. Polymorphism analysis and tissue specificity. J Biol Chem 1994; 269: 15 217-22.
- Schramm G, Bufe A, Petersen A, Schlaak M, Becker WM. Molecular and immunological characterization of group V allergen isoforms from velvet grass pollen (Holcus lanatus). Eur J Biochem 1998;52:200-6.
- Kawamoto S, Fujimura T, Nishida M, Tanaka T, Aki T, Masubuchi M, et al. Molecular cloning and characterization of a new Japanese cedar pollen allergen homologous to plant isoflavone reductase family. Clin Exp Allergy 2002;32:1064-70.
- Scholl I, Wiedermann U, Forster-Waldl E, Ganglberger E, Baier K, Boltz-Nitulescu G, et al. Phage-displayed Bet mim 1, a mimotope of the major birch pollen allergen Bet v 1, induces B cell responses to the natural antigen using bystander T cell help. Clin Exp Allergy 2002;32:1583-8.
- Yu CJ, Lin YF, Chiang BL, Chow LP. Proteomics and immunological analysis of a novel shrimp allergen, Pen m 2. J Immunol 2003;170:445-53.
- Lüttkopf D, Müller U, Skov PS, Ballmer-Weber BK, Wüthrich B, Skamstrup Hansen K, et al. Comparison of four variants of a major allergen in hazelnut (Corylus avellana) Cor a 1.04 with the major hazel pollen allergen Cor a 1.01. Mol Immunol 2001;38:515-25.
- Rose TM, Schultz ER, Henikoff JG, Pietrokovski S, McCallum CM, Henikoff S. Consensus-degenerate hybrid oligonucleotide primers for amplification of distantly related sequences. Nucleic Acids Res 1998;26:1628-35.
- Corpet F. Multiple sequence alignment with hierarchical clustering. Nucleic Acids Res 1988;16:10881-90.
- Heger A, Holm L. Sensitive pattern discovery with ‘fuzzy’ alignments of distantly related proteins. Bioinformatics 2003;19:I130-7.
- Plewniak F, Bianchetti L, Brelivet Y, Carles A, Chalmel F, Lecompte O, et al. PipeAlign: a new toolkit for protein family analysis. Nucleic Acids Res 2003;31:3829-32.
- Bennett SP, Lu L, Brutlag DL. 3MATRIX and 3MOTIF: a protein structure visualization system for conserved sequence motifs. Nucleic Acids Res 2003;31:3328-32.
- Higgins D, Thompson JD, Higgins DG, Gibson TJ. CLUSTAL W: Improving the sensitivity of progressive multiple sequence alignment through sequence weighting, position-specific gap penalties and weight matrix choice. Nucleic Acids Res 1994;22:4673-80.
- Frohman MA, Dush MK, Martin GR. Rapid production of full-length cDNAs from rare transcripts: amplification using a single gene-specific oligonucleotide primer. Proc Natl Acad Sci USA 1988;85:8998-9002.
- Kumar S, Tamura K, Jakobsen IB, Nei M. MEGA2: molecular evolutionary genetics analysis software. Bioinformatics 2001;17:1244-5.
- Mirza O, Henriksen A, Ipsen H, Larsen JN, Wissenbach M, Spangfort MD, et al. Dominant epitopes and allergic cross-reactivity: complex formation between a Fab fragment of a monoclonal murine IgG antibody and the major allergen from birch pollen Bet v 1. J Immunol 2000;165:331-8.
- Vieths S, Scheurer S, Ballmer-Weber B. Current understanding of cross-reactivity of food allergens and pollen. Ann NY Acad Sci 2002;964:47-68.
- Fluckiger S, Fijten H, Whitley P, Blaser K, Crameri R. Cyclophilins, a new family of cross-reactive allergens. Eur J Immunol 2002;32:10-7.
- Bolten E, Schliep A, Schneckener S, Schomburg D, Schrader R. Clustering protein sequences: structure prediction by transitive homology. Bioinformatics 2001;17:935-41.
- Pearson WR. Identifying distantly related protein sequences. Comput Appl Biosci 1997;13:325-32.
- Brenner SE, Chothia C, Hubbard TJ. Assessing sequence comparison methods with reliable structurally identified distant evolutionary relationships. Proc Natl Acad Sci USA 1998;95:6073-8.
- Rost B. Twilight zone of protein sequence alignments. Protein Eng 1999;12:85-94.
- Hileman RE, Silvanovich A, Goodman RE, Rice EA, Holleschak G, Astwood JD, et al. Bioinformatic methods for allergenicity assessment using a comprehensive allergen database. Int Arch Allergy Immunol 2002;128:280-91.
- Furmonaviciene R, Shakib F. The molecular basis of allergenicity: comparative analysis of the three dimensional structures of diverse allergens reveals a common structural motif. J Clin Pathol Mol Pathol 2001;54:155-9.
- Rafnar T, Griffith IJ, Kuo MC, Bond JF, Rogers BL, Klapper DG. Cloning of Amb a I (Antigen E), the major allergen family of short ragweed pollen. J Biol Chem 1991;266:1229-36.
- Asturias JA, Arilla MC, Bartolome B, Martinez J, Martinez A, Palacios R. Sequence polymorphism and structural analysis of timothy grass pollen profilin allergen (Phl p1l). Biochim Biophys Acta 1997;1352:253-7.
- Federov AA, Ball T, Mahoney NM, Valenta R, Almo SC. The molecular basis for allergen cross-reactivity: crystal structure and IgE-epitope mapping of birch pollen profilin. Structure 1997;5:33-45.
- Yagami T. Allergies to cross-reactive plant proteins. Latex-fruit syndrome is comparable with pollen-food allergy syndrome. Int Arch Allergy Immunol 2002;128:271-9.
- Tao AL, He SH. Bridging PCR and partially overlapping primers for novel allergen gene cloning and expression insert decoration. World J Gastroenterol 2004;10:2103-8.