Recombinant interferon-alpha2b poly(lactic-co-glycolic acid) microspheres: pharmacokinetics-pharmacodynamics study in rhesus monkeys following intramuscular administration1
Introduction
Interferon-alpha (IFN-α) is a multifunctional cytokine. It has the property of inhibiting the growth of tumor cells and regulating immune function, is widely used in the clinical treatment of viral diseases and cancers, and is the first antiviral drug used in the treatment of hepatitis B and C[1,2]. However, since its half-life is short, long-term frequent injection is needed. This unfortunately results in a large fluctuation in plasma concentration, limited positive effects, and relatively more side effects[3]. Therefore, the development of a sustained-release injection of IFN-α is urgently needed.
Studies on long-acting injection of IFN-α have been focusing on poly(ethylene glycol) (PEG) modification and biodegradable particle delivery system. The development of PEGylated IFN-α has been very successful, since the early 1990s, PEG-IFN-α2b with a sustained release over a 1 week period was approved by the Food and Drug Administration (FDA). It is now widely used in clinical practice, and good therapeutic effects have been achieved[4]. However, the biodegradable particle delivery system of IFN-α is still at the research stage. Since leuprorelin 3-month depot microspheres were approved in the market, the biodegradable polylactic acid/poly(lactic-co-glycolic acid) (PLA/PLGA) microspheres have been considered to be a good prospect in the development of long-acting injections of protein drugs due to their long sustained release. Studies on IFN-α microspheres have reported about PLA-encapsulated IFN-α microspheres comprising calcium alginate microcores[5], PLGA IFN-α microspheres using poloxamer as a stabilizer[6], and IFN-α PLGA microspheres modified with PEG[7]. However, most of these studies involved in vitro experiments on microspheres, such as increasing the encapsulation efficiency and loading capacity of microspheres, maintaining the stability of drugs, and reducing the burst-release effects. In contrast, few studies involved in vivo experiments, particularly, pharmacokinetic and pharmacodynamic study of IFN-α PLGA microspheres in primates. In this study, IFN-α2b PLGA microspheres were prepared with our patented technology to particularly investigate the sustained-release properties and biological activities of these microspheres in rhesus monkeys.
Materials and methods
Reagents Soluble (3.47×108 IU/mL containing 1% serum albumin) and commercial lyophilized (5×106 IU/vial) IFN-α2b were kindly provided by Shenzhen Neptunus Interlong Bio-Tech Co, Ltd (Shenzhen, China). The PLGA (LA/GA=50/50 with inherent viscosities of 0.39, 0.89, and 1.13 dL/g) was obtained from Absorbable Polymers International (Birmingham, AL, USA). Polyvinyl alcohol-124 (PVA-124) was obtained from Shandou Chemical Reagents Co (Shandou, China); poloxamer 118 and Tween 80 were purchased from BASF (Ludwigshafen, Germany); dichloromethane was obtained from Guangzhou Chemical Reagents Co (Guangzhou, China). IFN-α2b enzyme-linked immunosorbent assay (ELISA) kit was obtained from Shanghai Senxiong Technology Industry Co, Ltd (Shanghai, China). Neopterin ELISA kit was obtained from IBL Co, Ltd (Minneapolis, MN, USA). All other chemicals used were of analytical reagent grade.
Preparation of microspheres IFN-α2b PLGA microspheres were prepared using our patented water-in-oil-in-water (w/o/w) double emulsion-solvent evaporation method[8]. In brief, an appropriate amount of IFN-α2b was dissolved in a certain amount of phosphate buffer (pH=7.0) containing poloxamer 118 (as a stabilizer), thus forming the internal water phase; PLGA was dissolved in dichloromethane, thus forming the oil phase (PLGA concentration was 15%, w/v). The internal water phase was added in the oil phase and vortexed using a YQ-3 electric homogenator (Jiangyin Electronic Machinery Factory, Jiangyin, China) for 1 min in an ice water bath, thereby resulting in primary emulsion (w/o). The w/o emulsion was transferred in 150 mL of 2% PVA solution (external water phase) and stirred using a FSH-2A adjustable mixer (Medical Instrument Factory, Jintan City, Jiangsu Province, China) for 10 min in an ice water bath, thereby resulting in double emulsion (w/o/w). Finally, the w/o/w emulsion was transferred in 800 mL of 0.25% PVA solution and stirred for 3 h to allow volatilization of dichloromethane and solicitation of microspheres. After washing 3 times with distilled water, the microspheres were harvested using a refrigerated centrifuge (Hitachi Company, Tokyo, Japan). The microspheres were vacuum-dried for further experiments. PLGA with inherent viscosities of 0.39, 0.89, and 1.13 dL/g was used to prepare the microspheres.
Electron-microscopic examination After the samples were gilded on an ion film-plating machine, the surface morphology of the microspheres was monitored by scanning electron microscopy (SEM) carried out using a 400F field emission scanning electron microscope (Zeiss, Oberkochen, Germany). The average diameter was calculated from 300 microspheres.
In vitro release First, 120 mg of IFN-α2b microspheres was placed in a 5 mL Eppendorf tube and incubated in 2 mL of phosphate buffer (pH=7.4) containing 0.02% v/v Tween 80. The tube was placed in a water bath/shaker (100 r/min) (Harbin Donglian Electronics Company, Harbin, China) at 37 °C. The solutions were filtrated regularly at 2, 6, and 12 h and 1, 2, 5, 9, 14, 19, 24, and 30 d. Two milliliters of fresh phosphate-buffered saline (PBS) was added to the microspheres after all the filtrates were collected. The protein level in each harvesting was determined by the Lowry method and cumulative release was calculated.
Animals and treatments Six male rhesus monkeys weighing 5±0.5 kg and about 4–5 years of age were purchased from the South-China Primate Research & Development Center, Chinese Academy of Sciences. The animals were clearly identified and received a standardized diet during the period of acclimation and were in good health at the time of the study. This study was conducted in compliance with the principles of laboratory animal care of Sun Yat-Sen University. The animals were randomly divided into 2 groups (n=3). The animals were injected intramuscularly with either 3 MIU/kg commercial IFN-α2b lyophilized powder or IFN-α2b PLGA microspheres (inherent viscosity of 0.89 dL/g) depending on which group they belonged to. Peripheral blood was harvested at different time points after the injections. The serum was isolated by centrifugation and stored at –20 ºC.
ELISA analysis The concentrations of IFN-α2b and neopterin were determined using commercial ELISA kits according to the manufacturers’ instructions. For determining the IFN-α2b concentration, all serum samples were diluted 20-fold to adjust within the detectable range (31.25–2000 pg/mL). The samples were harvested before or after 0.5, 1, 2, 4, 6, 9, 12, 24, 30, 33, 36, 48, 72, 96, 144, 192, 240, 288, 336, 384, 408, and 432 h treatment. For determining the neopterin (Np) concentration, the serum samples were harvested before or after 1, 4, 6, 9, 12, 22, 24, 27, 30, 33, 36, 48, 72, 96, 120, 144, 168, 192, 216, 240, 264, 288, 312, 336, and 360 h treatment. The measurements were repeated with different dilutions to confirm the validity of the analyses. To verify the specificity of this system, recovery tests were carried out by adding specific amounts of standards. The samples were read on an ELX-800 Bio-Tek Microplate reader (Winooski, VT, USA).
Data analysis Pharmacokinetic parameters were calculated with 3P97 software (China Mathematical Pharmacological Association, Shanghai, China). All values were expressed as mean±SD. Mean residence time (MRT), area under the concentration-time curve (AUC), and other pharmacokinetic parameters were obtained based on a non-compartment model. The t-test was used to analyze the differences in the pharmacokinetic and pharmacodynamic parameters. P<0.05 was considered to be statistically significant.
Results
Particle size and morphology of microspheres The surface morphologies of single and multiple IFN-α2b PLGA microspheres with inherent viscosities of 0.39, 0.89, and 1.13 dL/g are respectively shown in Figure 1. The average diameters of the microspheres are given in Table 1. SEM revealed the 3 types of microspheres to be spherical with a microporous surface. The microspheres prepared from the PLGA materials with high viscosity were smooth and compact.
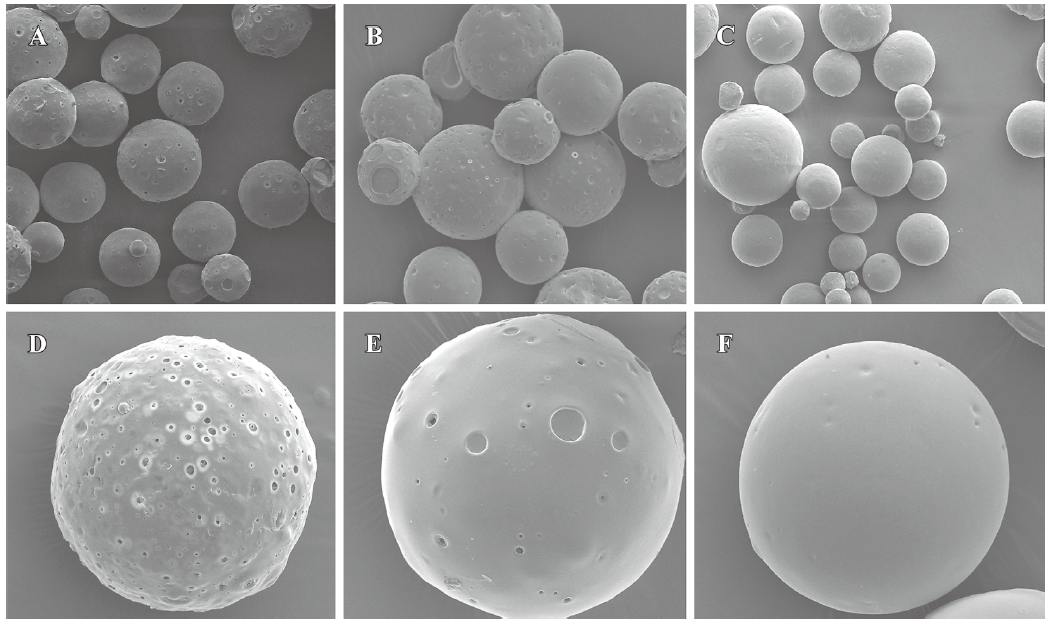
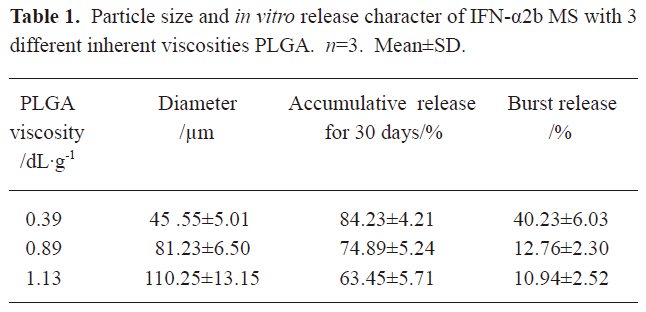
Full table
In vitro release of microspheres To select the best formulation with a lower burst release and optimal accumulative release profile, we used PLGA with 3 different inherent viscosities. The microspheres showed a burst release during the first 2 h, followed by a cumulative release until the 30th day (see Figure 2, 3). The accumulative release profile increased along with a decrease in the PLGA inherent viscosities and burst release became very important (see Table 1). These results indicated that the PLGA with the inherent viscosity of 0.89 dL/g was the best formulation. We used this PLGA for the in vivo experiments.
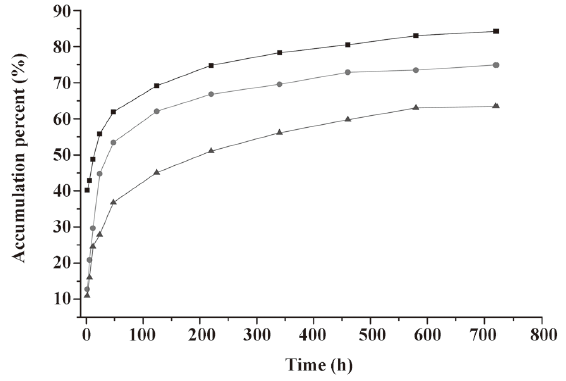
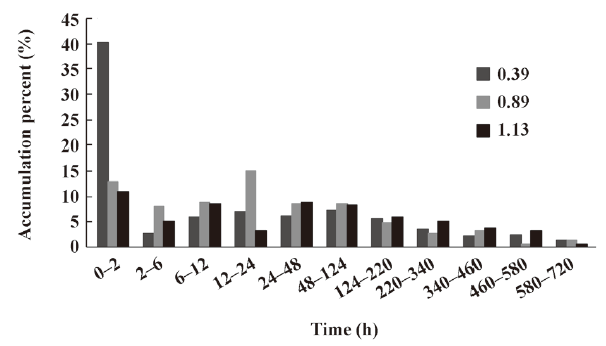
Pharmacokinetics of microspheres in healthy rhesus monkeys Figure 4 shows the blood IFN profiles after the administration of native IFN-α2b and IFN-α2b microspheres. The concentration of IFN-α2b in the native IFN group reached a peak at 1.96 h and then declined rapidly to the level before the injection at 96 h. On the other hand, the concentration of IFN-α2b in the microsphere group reached a peak at 7.29 h and then declined to the lowest level at around 192 h, remained at this level for 4 d, a small flat peak emerged later, and finally it decreased to the level before the injection at 432 h. Compared with the native IFN-α2b group, the Cmax, tmax, and serum residence time of IFN-α2b in the microsphere group were significantly prolonged, as reflected by the increasing AUC. The pharmacokinetic parameters showed that the differences in the averages of tmax, Cmax, kterminal, AUC, and MRT between the 2 groups were significant (P<0.01; Table 2).
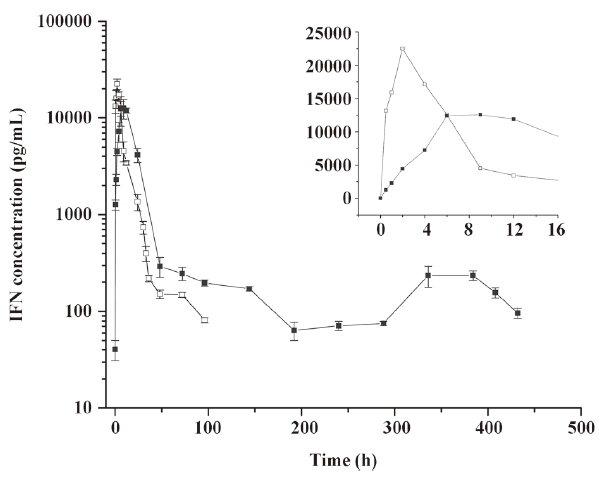
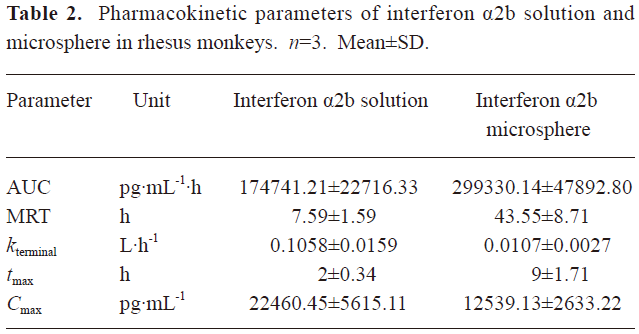
Full table
Pharmacodynamics of microspheres in healthy rhesus monkeys Figure 5 shows the blood neopterin profiles after the administration of native IFN-α2b or IFN-α2b microspheres. The neopterin concentration began to increase 6 h after injection in both groups and reached a peak around 24 h simultaneously in both groups. However, the peak concentration in the native IFN-α2b group was 2-fold higher than that in the microsphere group and decreased slowly to the level before the injection at about 288 h. In the microsphere group, the concentration declined to the lowest level when another small increase occurred and then declined to the level before the injection at 360 h. The serum residence time of neopterin was significantly different between the 2 groups (P<0.05; Figure 5).
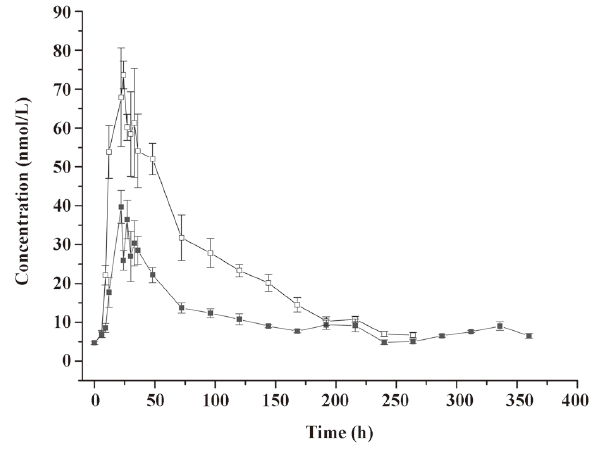
Discussion
In the present study, the IFN-α2b PLGA microspheres were prepared with our patented method. The microspheres with 3 different viscosities of PLGA appeared under an electron microscope as follows: spherical, with a smooth surface, with no adhesion, and with good morphological features. The higher the viscosity of PLGA as the skeleton material was, the longer the diameter. It caused the less insignificant burst release of the produced microspheres, and the lower cumulative release within 30 d. This phenomenon may occur because with an increase in viscosity, the molecular weight of polymer materials increases. Thus, the formulated sustained-release skeleton becomes more compact and the resistance for drugs to be released out of the skeleton is then raised, resulting in a slow release, insignificant burst release, and low total release within a certain time. The surface features of the microspheres prepared from the different viscosities of PLGA materials (Figure 1) can also be explained. With an increase in viscosity, the surface micropores are reduced and the texture becomes more compact, thereby making it more difficult for drugs to be released from the microsphere skeleton. Similar observations have been previously reported[9]. Figure 2 demonstrates that for all 3 types of microspheres, the in vitro sustained release may last for 30 days; however, the release features are obviously different. In this study, the PLGA (viscosity of 0.89 dL/g) microspheres with higher in vitro cumulative release but lower burst release were chosen for investigating the pharmacokinetic and pharmacodynamic features.
In the rhesus monkeys, after an intramuscular injection of 3 MIU/kg IFN-α2b powder and microspheres, the main pharmacokinetic parameters tmax, MRT, and AUC in the microsphere group were 4.5 times, 5.74 times, and 1.7 times, respectively, of those in the native IFN-α2b group, and the plasma concentration could be maintained for 18 days. The results of the pharmacokinetic analysis revealed that the in vivo release of IFN-α2b was faster than its in vitro release. This may be due to the complex environment in the rhesus monkey, particularly, the existence of enzyme effects. In addition, the plasma concentration in the microsphere group showed a small peak at the end of the concentration-time curve. This may have occurred because at the end of the release, the hydrolysis of the skeleton materials was accelerated and the microspheres were disintegrated rapidly and the remaining drugs were released suddenly, thereby leading to the fluctuation in the plasma concentration, which was also observed in our pharmacodynamic experiments.
In the pharmacodynamic study, neopterin was used as the indicator. Based on the changes in the neopterin content in the serum after injection, the biological activity of the IFN-α microspheres in rhesus monkeys was investigated. Neopterin is the marker of IFN activity in primate animals. It has been used to study IFN-γ[10,11] and IFN-β and IFN-α[12,13]. Since the neopterin production of monocyte-macrophages induced by IFN-α is far below that induced by IFN-γ, based on the pharmacodynamic research method for PEG-IFN-β[14], the dosage adopted in this study was relatively high. The experimental results revealed that the IFN-α2b microspheres had biological activities within a time period as long as 12 d, ie, longer by 72 h than the time in the native IFN-α2b group. We found that within 6 h after injection, the neopterin concentrations in the native IFN-α2b group and the microsphere group did not change, probably because the neopterin generation induced by IFN is the result of the total effects of many immunological responses mediated by cellular receptors; to cause changes in neopterin concentration requires a certain time. The neopterin concentrations in the 2 groups reached a peak at the same time – not just a parallel shift in the peak time of IFN-α2b. After the peak, the decline in the 2 curves was essentially the same. Although the maintenance time of the concentration of IFN-α2b was shorter in the native IFN-α2b group than in the microsphere group, the neopterin concentrations almost declined to the lowest level at the same time, suggesting that the in vivo generation and changes in neopterin obey a relatively independent law associated with time. The above phenomenon is consistent with the results of the pharmacodynamic research on IFN-β and PEG-IFN-β[15]. In addition, we noticed that the peak concentration of IFN-α2b was higher in the native IFN-α2b group, whose neopterin concentration was also higher, while the peak concentration of IFN-α2b was lower in the microsphere group, whose neopterin concentration was also lower. The peak concentrations of IFN-α2b and neopterin were positively correlated. Figure 6 revealed that the concentration-time curves of IFN and neopterin in the microsphere group had similar changing laws, ie, they both had a small peak at the end, thus indicating that a change in neopterin concentration is in fact a reflection of a change in the IFN concentration based on the features observed at the end of the release. The changing laws of the mutual effects of neopterin and IFN concentrations are being studied by us.
Our data suggest that the IFN-α2b PLGA microspheres prepared in this study can improve the pharmacokinetic and pharmacodynamic features of IFN-α2b, effectively increase the residence time, and maintain longer biological effects in the rhesus monkey. This IFN-α delivery system provides a new approach to improve the present IFN-α-based therapies.
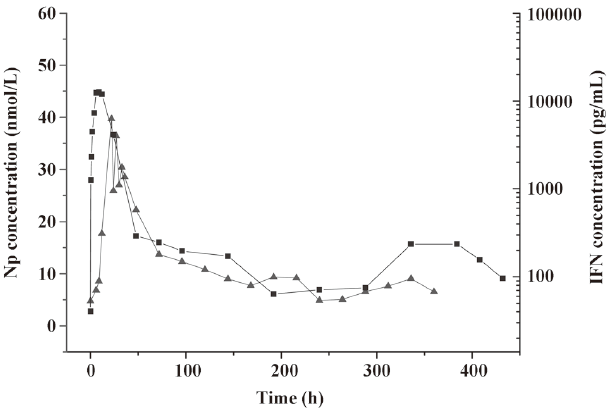
References
- Férir G, Kaptein S, Neyts J, De Clercq E. Antiviral treatment of chronic hepatitis B virus infections: the past, the present and the future. Rev Med Virol 2008;18:19-34.
- Yu ML, Huang CF, Dai CY, Huang JF, Chuang WL. Long-term effects of interferon-based therapy for chronic hepatitis C. Oncology 2007;72:16-23.
- Uzé G, Schreiber G, Piehler J, Pellegrini S. The receptor of the type I interferon family. Curr Top Microbiol Immunol 2007;316:71-95.
- Pogorzelska J, Flisiak R. Long-term effects of HBV treatment Peg-IFN alfa-2a alone or combined with lamivudine. Przegl Epidemiol 2007;61:433-7.
- Zhou S, Deng X, He S, Li X, Jia W, Wei D, et al. Study on biodegradable microspheres containing recombinant interferon-alpha-2a. J Pharm Pharmacol 2002;54:1287-92.
- Sánchez A, Tobío M, González L, Fabra A, Alonso MJ. Biodegradable micro- and nanoparticles as long-term delivery vehicles for interferon-alpha. Eur J Pharm Sci 2003;18:221-9.
- Krasowska D, Chodorowska G, Bartosińska J, Warmińska J, Jermak A, Kur A, et al. Serum levels of neopterin in patients with lichen planus. Ann Univ Mariae Curie Sklodowska 2004;59:346-50.
- Xu AL, Yang F, Gao JP, Zhao YM, Wang L, Dong ML, inventors; Sun Yat-Sen University, Guangzhou. A method for preparation of Interferon-loaded biodegradable PLGA microspheres. China patent 1660412A. 2005 Aug 31.
- Reich G. Ultrasound-induced degradation of PLA and PLGA during microsphere processing: influence of formulation variables. Eur J Pharm Biopharm 1998;45:165-71.
- Cesur S. Neopterin: a marker used for monitoring infections. Mikrobiyol Bul 2005;39:251-60.
- Lortkipanidze NT, Tevzadze MSh, Kamkamidze GK. Interferon-gamma and neopterin in alopecia areata. Georgian Med News 2005.53-7.
- Iyer A, Hatta M, Usman R, Luiten S, Oskam L, Faber W, et al. Serum levels of interferon-gamma, tumour necrosis factor-alpha, soluble interleukin-6R and soluble cell activation markers for monitoring response to treatment of leprosy reactions. Clin Exp Immunol 2007;150:210-6.
- Brearley C, Jaber A, Bertolino M, Priestley A, Seiberling M. Assessment of the safety, tolerability, and PK/PD properties of two new formulations of subcutaneously administered IFN-beta1a: a double-blind, placebo-controlled comparison with the currently available formulation. Int J Clin Pharmacol Ther 2007;45:307-18.
- Mager DE, Neuteboom B, Jusko WJ. Pharmacokinetics and pharmacodynamics of PEGylated IFN-beta 1a following subcutaneous administration in monkeys. Pharm Res 2005;22:58-61.
- Pepinsky RB, LePage DJ, Gill A, Chakraborty A, Vaidyanathan S, Green M, et al. Improved pharmacokinetic properties of a polyethylene glycol-modified form of interferon-beta-1a with preserved in vitro bioactivity. J Pharmacol Exp Ther 2001;297:1059-66.