Bioactivity, pharmacokinetics, and immunogenicity assays in preclinical and clinical trials for recombinant human endostatin1
Introduction
Endostatin has attracted wide attention due to its specific inhibition of new vessel generation since its discovery by O’Reilly et al in 1997[1]. Human endostatin is the NC1 domain of the α1 chain of type XVIII collagen with 183 amino acids[2], and has been shown to have an inhibitory effect on new vessel formation in many experimental models, including the chick embryo allantochorion, rat cornea, and arterial circle cultured in vitro[1,3,4]. Several studies have also proven that endostatin has an inhibitory effect on vessel generation in tumors and in pathological vascular inflammation in a definite dose-dependent manner without any apparent side-effects, toxicity, or developmental drug resistance[1,5–7]. In addition, endostatin directly targets vascular endothelial cells whose hereditary feature is stable. Therefore, the chance of inducing drug resistance is markedly reduced. Due to the complexity of the therapeutic efficacy, recent studies have focused on the bioactivity of recombinant proteins of human endostatin[8–11]. With the development of recombinant DNA technology, many highly-effective expression and purification systems have been established to obtain recombinant human endostatin (rhEndostatin) with high purity[1,4,12].
Several reports have indicated that no toxic effects or resistance to therapy was observed even after prolonged therapy with endostatin in experimental mouse models[6,13,14]. RhEndostatin has also been proven to be biologically active and non-toxic in preclinical pharmacology and toxicology studies[15,16]. The results provide the impetus to initiate clinical trials of endostatin in patients with cancer. Some clinical trials have been performed during the past decade with the results demonstrating that rhEndostatin ranges from no apparent clinical activity (eg advanced neuroendocrine) to significant tumor inhibition though all clinical trials, showing high safety, rare untoward reactions or anaphylactic responses, and no drug resistance[17–25]. The differences of the expression system, protein structure, purification means, and tumor types may be responsible for the discrepancy in the bioactivity of rhEndostatin.
In our previous work, we expressed, purified, and assayed the bioactivity of rhEndostatin[26]; In the present study, we report on further tests of purified rhEndostatin expressed from Escherichia coli according to Pharmacopoeia of the People’s Republic of China (2005 edition, part Ш)[27]. We measured the in vitro bioactivity and in vivo antitumoral activity of rhEndostatin, and then analyzed the pharmacokinetics and immunogenicity of rhEndostatin in rhesus monkeys and patients. The results of these studies are described in this report.
Materials and methods
Samples The rhEndostatin samples were supplied by ZhongKai Bio-Pharma Co, Ltd (Suzhou, China).
Analysis of rhEndostatin preparations
Molecular weight test and purity determination[27] A mass spectrographic analysis was performed by the National Biomedicine Analysis Center (Beijing, China). SDS-PAGE was prepared and performed according to Appendix IVC of Pharmacopoeia of the People’s Republic of China (2005 edition, part Ш). The samples were analyzed for the molecular weight of the rhEndostatin protein by 12.5% reduced SDS-PAGE. Proteins were stained with Coomassie brilliant blue R250.
Chromatographic conditions for reverse phase HPLC were performed according to Appendix IIIB of Pharmacopoeia of the People’s Republic of China (2005 edition, part III). The fixed phase involved water symmetry C4, and the mobile phases involved 0.1% trifluoroacetic acid (TFA)-water (1:1000, v/v) and TFA-acetonitrile (ACN)-water (1:950:50, v/v/v), respectively. After balance, the sample (10 µL) was added to the column, and the flow rate was set at 1.0 mL/min. The separation and the analysis were conducted at 30 °C, and the detection wave length was set at 280 nm.
N- and C-terminal sequence assay and amino acid composition assay The N- and C-terminal sequence of rhEndostatin was analyzed by an amino acid sequenator, and the analysis was performed by the National Biomedicine Analysis Center. The amino acid composition was determined by an amino acid composition analyzer by the State Key Laboratory of Pharmaceutical Biotechnology of Nanjing University (Nanjing, China).
Peptide mapping and mass peptide spectrum analysis[27] Peptide mapping analysis was done based on the Appendix VIIE of Pharmacopoeia of the People’s Republic of China (2005 edition, part III). Briefly, the rhEndostatin samples were analyzed by reverse-phase HPLC after trypsin digestion. The chromatographic conditions for reverse-phase HPLC were the same as those mentioned earlier. The mass spectrum analysis of the peptides was determined by mass spectrometer, and the analysis was carried out by the Drug and Biological Product Standardization Research Center of China (Beijing, China).
Measurement of other parameters[27] Other parameters, in accordance with the requirement and methods of the Appendix of Pharmacopoeia of the People’s Republic of China (2005 edition, part III) were also measured. They included pH values (Appendix VA), residual antibiotics (Appendix IXA), exogenous DNA content (Appendix IXB), host cell protein content (Appendix IXC), sterility test (Appendix XIIA), pyrogen test (Appendix XIID), residual endotoxin content (Appendix XIIE), and undue toxicity test (Appendix XIIF). In addition, a disulfide bond allocation analysis was carried out by the Shanghai Institutes for Biological Sciences, Chinese Academy of Sciences (Shanghai, China).
In vitro activity study
Preparation and culture of human umbilical vein endothelial cells Human umbilical vein endothelial cells (HUVEC) were separated from the cord blood of neonates. Neonate cord blood was obtained and kept in preservation solution (supplemented with 140 mmol/L NaCl, 4 mmol/L KCl, 5.2 mmol/L Na2HPO4, 1.5 mmol/L KH2PO4, 110 mmol/L glucose, and 105 U/mL penicillin–streptomycin). After repeated rinsing with phosphate-buffered saline (PBS) containing penicillin (100 U/mL) and streptomycin (100 µg/mL), the umbilical vein was perfused with collagenase II prepared in Hanks’ solution for 20 min and rinsed with culture medium. The wash was pooled and centrifuged to recover HUVEC. The recovered HUVEC were cultivated in complete RPMI-1640 medium (supplemented with 20% fetal bovine serum [FBS], 2 ng/mL basic fibroblast growth factor (bFGF), 1% bovine serum albumin [BSA], 90 µg/mL heparin, 100 U/mL penicillin, and 100 µg/mL streptomycin) at 37 °C in a humidified 5% CO2 atmosphere.
Detection of proliferation-inhibition effect of rhEndostatin on HUVEC The rhEndostatin solutions were prepared with NaAc/HAc buffer (pH 7.0; 10 mmol/L) to various concentrations of 31.25, 62.5, 125, 250, 500, 1000, 2000, and 4000 ng/mL before use. According to a previously-established protocol[26], the plasmid pcDNA3.0–vascular endothelial growth factor (VEGF) was transfected using Lipofectamine 2000 as instructed by the manufacturer. Stable transfectants (HUVEC carrying plasmid pcDNA3.0–VEGF) were selected and preserved for future use after routine identification. The transfectants were seeded onto 96-well plate at 1×104/pool and maintained in complete RPMI-1640 medium with 10% FBS at 37 °C in a humidified 5% CO2 atmosphere for 24 h. RhEndostatin (150 µL/well) was then added at serial concentrations, while an equal amount of buffer was added to the control group. Each concentration of rhEndostatin had 9 repeat wells (3 wells per method). Seventy-two hours later, the number of cells was counted under a microscope after treatment with 0.05% trypsin (0.5 mL) or assayed using colorimetric 3-(4,5-dimethylthiazol-2-yl)-2,5-diphenyltetrazolium bromide (MTT) or lactate dehydrogenase (LDH) as the substrate following the manufacture’s instructions[28]. The proliferation–inhibition rate of rhEndostatin on HUVEC at each concentration was calculated using the following formula:
where OD is the optical density.
Dosage linear regression analysis and the effective concentration for 50% inhibition of cell proliferation potency assay The proliferation-inhibition rate of HUVEC was performed as the ordinate, and the according logarithm of each concentration of rhEndostatin as the abscissa to make the linear regression. The regression equation y=a+bx was obtained, where a and b represent the intercept and slope rate of each regression curve, respectively. The effective concentration for 50% inhibition of cell proliferation (IC50) was calculated from the regression equation. The dose of IC50 representing 1×105 units was obtained according to the amount and concentration of the samples, and the specific activity was deduced from IC50.
In vivo activity study[29]
Animals and tumor cells Six-to-eight-week-old male Kunming mice (grade SPF; 20±2 g), were purchased from the Animal Center of Nanjing Medical University (Nanjing, China). The animals were supplied with laboratory chow and water ad libitum and kept in an animal facility maintained at 22 °C on a 12 h light/dark cycle. H22 murine hepatoma cells were obtained from the Shanghai Laboratory Animal Center, Chinese Academy of Sciences. The experiments were carried out by the Animal Welfare Committee of the institutions.
Tumor growth inhibition of rhEndostatin A liver tumor model was established by a subcutaneous injection of H22 tumor cells resuspended in PBS (1×106 cells/0.2 mL) into the flank of each mouse[30]. Forty mice were divided into 4 groups (n=10 in each group). The control group received diluents (10 mmol/L NaAc/HAc buffer, pH 7.0); the other 3 groups received rhEndostatin reference preparation at doses of 5, 10, and 20 mg/kg, respectively. Each mouse was intravenously injected with the diluents of rhEndostatin via the tail vein daily for 7 continuous days. The animal experiment was repeated 12 times. The tumor growth inhibition rate (IR) was determined by the following formula:
In order to quantify the antitumoral activity of rhEndostatin and compare the in vivo potency with the in vitro activity, we assumed that the estimated effective dose for 50% inhibition of tumor growth (ED50) represented 1×107 units, and the potency of each rhEndostatin preparation was calculated using the following formula:
Pharmacokinetics and immunogenicity of rhEndostatin in rhesus monkeys
Animals Rhesus monkeys (weighing 5.5±1.2 kg, approximately 5 years old, grade I, certificate SCXK [Su] 2002-0007) were provided by the Suzhou Xishan Laboratory (Suzhou, China). The animals were individually housed in stainless steel cages and fed a standard monkey diet; water was available ad libitum. The Tianjin Drug Academy (Tianjin, China) conducted the pharmacokinetic study, and Shanghai Medicine Industry Institute (Shanghai, China) made the immunogenicity assays for the preclinical trial, respectively. The experiments were carried out by the Animal Welfare Committee of the institutions.
Pharmacokinetics Six rhesus monkeys were administered rhEndostatin at the dose of 3 mg/kg by ulnar vein over a 30 min period. Whole blood samples (1.5 mL) were drawn from the saphenous veins of the animals at the following time points: before dose administration (0 h), during first infusion (0.083, 0.25, and 0.5 h), and after the first dose (0.583, 0.75, 1, 1.25, 1.5, 2.5, 4.5, 8.5, 12.5, 24.5, and 48.5 h) of rhEndostatin. The blood was centrifuged at 3000×g for 10 min, and the separated serum was stored at –20 ºC until assayed.
The human endostatin protein accucyte enzyme immunoassay kit (Cytimmune Sciences, Germany) was used to determine the rhEndostatin levels in the serum samples. The assay was performed according to the operating instructions specified by the manufacturer. A series of calibration standards were set in each microplate and the concentration range on the approaching straight line segment was utilized to assess the relative recovery and precision of rhEndostatin. The rhEndostatin levels in the unknown samples were obtained by calculation from the calibration standard curves on the same microplate. The serum drug concentration-time data were analyzed by a compartmental analysis using the practical pharmacokinetic program 3p97 (Chinese Pharmacological Society and the Mathematic and Pharmaco-Professional Committee, Beijing, China).
Serum antibodies to rhEndostatin in rhesus monkeys Twenty-four rhesus monkeys were divided into 4 groups (n=6 per group). One negative control group was administered with physiological saline (10 mL/kg) and 3 treated groups were administered rhEndostatin at doses of 6, 20, and 60 mg·kg-1·d-1 for 90 d using an iv drip, respectively. The serum samples were obtained at the following time points: before dose administration, 1, 2, 4, 11, and 13 weeks after the first dose, and 4 weeks after treatment.
ELISA was used to assess the immunogenicity of rhEndostatin during therapy. The assay was performed according to the guidelines of the procedure specified by the manufacturer. The serum samples were tested at dilutions of 1:40, 1:80, 1:160, and 1:320. In total, 200 µL horseradish peroxidase (HRP)-conjugated Staphylococcal protein A (SPA) at a dilution of 1:40 was added as a second antibody, and tetramethylbenzidine (TMB) was used as a substrate. Each sample was measured in duplicate. The OD450 was determined after 30 min of incubation. The data were analyzed to determine an endpoint titer of immunoreactivity.
Pharmacokinetics and immunogenicity of rhEndostatin in patients
Healthy volunteers, patients, and administration[31] The eligibility criteria excluded those who were pregnant or breast feeding or had a history of cardiac, hepatic, nephritic, or hematological diseases, those aged between 18 and 45 years, and those weighing above 50 kg. Twenty-four volunteers were divided into 3 groups (n=8 per group) and received rhEndostatin intravenously over a 2 h period at a single administration of 60, 120, and 180 mg/m2, respectively.
The eligibility criteria included patients aged between 18 and 65 years with progressive advanced tumors, for whom no standard treatment was available. The patients had to have a life expectancy of at least 3 months and an Eastern Cooperative Oncology Group performance status of 0 or 1. Patients were excluded if they were pregnant or breast feeding, had a history of a primary or metastatic brain tumor, or had a history of hematological diseases. The patients received a 28 d continuous iv infusion of rhEndostatin at dose of 10 (n=10), 20 (n=3), and 30 mg/m2 (n=3), respectively.
All volunteers and patients were informed about the experimental nature of this program and signed an approved informed consent form before therapy with rhEndostatin, as required by the institutional review boards of the respective institutions. The phase I clinical trial (lot 2005L02614) was licensed by the State Food and Drug Administration of China and carried out in Tianjin Tumor Hospital (Tianjin, China).
Pharmacokinetics of rhEndostatin in healthy volunteers and patients[31] In the single-dose group, whole blood samples (3 mL) were obtained before dose administration at 1, 5, 15, and 30 min, and 1, 2, 4, 8, 12, 24, 48, and 72 h after the first injection of rhEndostatin. In the successive administration of rhEndostatin (10 mg/m2) group, whole blood samples (3 mL) were obtained on d 1 and 7 before and at 15, 30, and 45 min, and 1, 1.5, 2, 4, 8, 12, and 24 h after administration. They were also obtained 2 and 24 h before administration on d 3, 5, 14, and 28, and at 48 and 96 h after administration on d 28. The blood samples were centrifuged at 3000×g for 10 min and the resulting serum was stored at –20 ºC until assayed.
The concentration of rhEndostatin in the serum specimens was measured using an enzyme immunoassay kit (R&D Systems, Minneapolis, USA). Pharmacokinetic parameters were estimated by compartmental modeling of the serum concentration-time data obtained from each patient. Parameters such as time-to-peak concentration (Tmax), the maximum serum concentration (Cmax), half-lives, and clearance were calculated from the primary parameters. The area under the curve (AUC) was calculated from the start until the end of the iv infusion by the linear trapezoid method.
Serum antibodies to rhEndostatin in patients Serum samples were obtained from patients in the successive administration of rhEndostatin (10 mg·m-2·d-1) group on d 7, 14, and 28 during therapy. Serum was removed for storage at –70 °C until endpoint titers of anti-rhEndostatin immunoglobulin (Ig)G and IgM antibodies were determined by ELISA. Briefly, rhEndostatin (300 µg/mL), resolved in carbonate–bicarbonate buffer (0.05 mol/L, pH 9.6) was coated in Immulon 96 plates (Costar, Corning, NY, USA) as the antigen. After blocking for 45 min at 37 °C with 0.2 mL PBS containing 5% BSA and 1‰ Tween-80, 0.1 mL serum samples non-diluted and diluted ath 1:2, 1:4 with PBS plus 2% BSA, 2‰ Tween-20, 1% glycerol, and 1‰ sodium azide were added in duplicate. After 2 h incubation at 37 °C, the wells were aspirated and washed 3 times with washing solution (4 g NaCl, 0.1 g KCl, 1.445 g Na2HPO4 12H2O, 0.1 g KH2PO4, and 0.05 mL Tween-20, pH 7.2). The plates were tamped dry, and the wells were incubated for 40 min at 37 °C with 0.1 mL of either a 1:300 dilution of protein A-peroxidase (Boster Biological Technology Company, Wuhan, China) or rabbit antihuman IgM (Beijing Zhongshan Jinqiao Biotechnology Co, Beijing, China). In the anti-rhEndostatin IgM antibody assay, the wells were incubated for another 40 min at 37 °C with 0.1 mL of a 1:300 dilution of HRP-goat antirabbit IgG (ZyMax; ZYMED Laboratories, San Francisco, California, USA). All of the wells were then washed 3 times with the above-mentioned washing solution, and 0.1 mL TMB substrate was added. The OD450 was determined after 40 min of incubation. Positive results was defined as the OD450 value resulting in a 2-fold increase over baseline absorbance.
Statistical methods The Student’s t-test and one-way ANOVA were employed for the statistical analysis of difference in 2 groups and multiple groups, respectively. All values were expressed as mean±SD. and P<0.05 was considered to be significant.
Results
Analysis of rhEndostatin The mass spectrographic analysis showed that the ion peak of the molecular weight (MW) of rhEndostatin was 20.227 kDa (data not shown). The MW of rhEndostatin detected by SDS-PAGE was 20.873 kDa and 20.564 kDa for 2 repeats, respectively (data not shown), thus the average value of 20.718 kDa was chosen and conformed to the theoretical value. The mass spectrum analysis of peptides showed that the MW of 11 main peptide fragments in 19 enzymolysis fragments conformed to theoretical value. The purity of rhEndostatin as measured by reversed phase (RP)–HPLC and non-reduced SDS–PAGE both exceeded 98% (data not shown). The disulfide bridges were determined to locate at Cys-33/Cys-173 and Cys-135/Cys-165, and N- and C-terminal analyses showed that the frontal 16 amino acids of the N-terminus were M-H-S-H-R-D-F-Q-P-V-L-H-L-V-A-L and the last 3 amino acids of the C-terminus were A-S-K. These results were in accordance with previous studies[32]. Furthermore, the amino acid composition analysis revealed that the amino acid composition was consistent with previously-published results with the exception of asparagines and glutamine being replaced by aspartate and glutamate, respectively. Ryptophane was destroyed by acid hydrolysis. The amino acid sequence of rhEndostatin was consistent with the sequence deduced by the DNA sequence. Peptide mapping of 3 batches of rhEndostatin digested by L-1-chloro-3-[4-tosylamido]-4-phenyl-2-butanone (TPCK)-TRYPSIN was completely identical (Figure 1). All parameters measured in this study conformed to the relevant regulations as shown in Table 1.
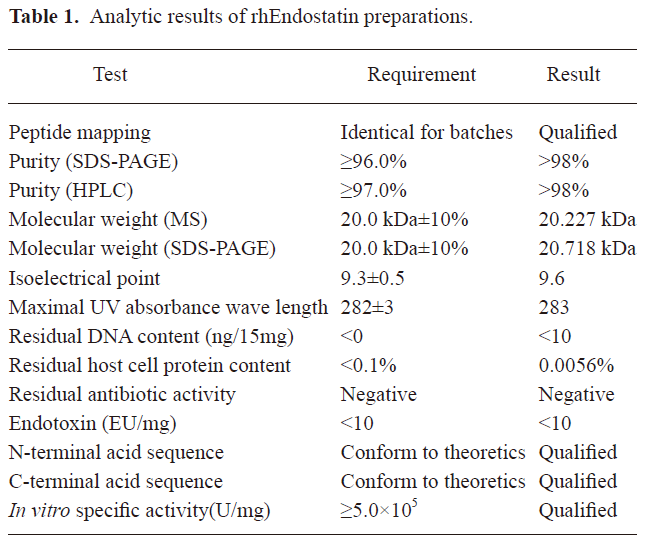
Full table
Proliferation-inhibition of rhEndostatin on HUVEC In the current study, the proliferation of HUVEC was significantly suppressed when concentrations of rhEndostatin reached 1000 ng/mL in any method used. A dose-dependent inhibition of proliferation was observed in the treated groups, while no proliferation-inhibition occurred in the control group. For the proliferation-inhibition assessment, the MTT colorimetric method was shown to have lower sensitivity and efficiency than the LDH colorimetric method (P<0.05 and P<0.01, respectively) because of its lower OD values under specific absorbance wavelengths (Figure 2). These results were consistent with those of our previous observation[26].
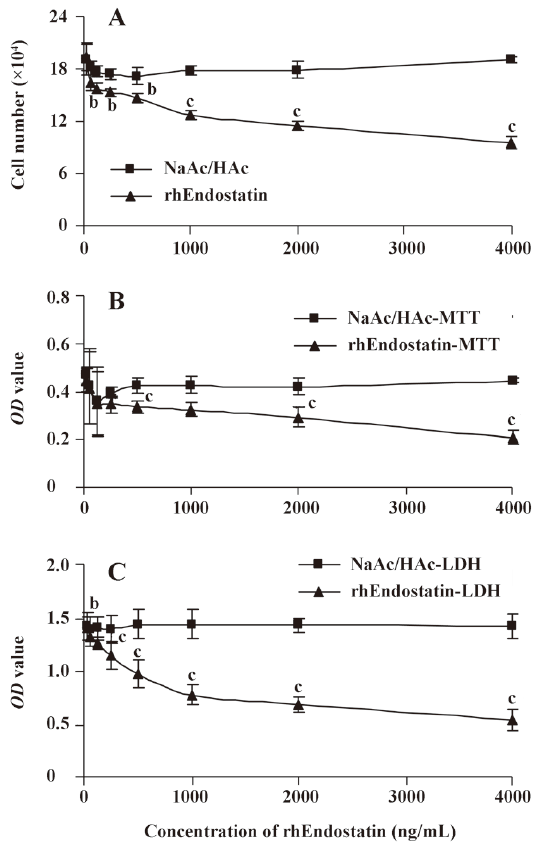
Although the stability for passages of HUVEC transfected with VEGF was improved and the deviation induced by the operation was reduced without stimulation by the addition of bFGF or VEGF in the culture, the cell proliferation-inhibition rate of rhEndostatin measured by different methods were shown to be highly variable (Table 2). Thus the in vitro-specific activity of rhEndostatin determined by the cell counting method, MTT assay, and LDH assay were 6.4×107, 6.7×107, and 3.8×108 U/mg, respectively, and the corresponding regression coefficiency was 0.95, 0.93, and 0.98, respectively, as shown in Table 2. Therefore, we could conclude from the regression coefficiency that comparatively, the LDH assay was the most sensitive and effective method.
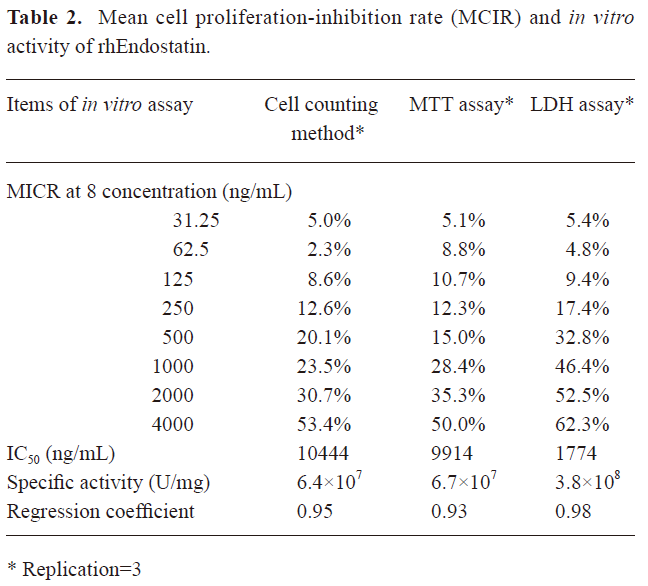
Full table
Owing to the significant differences among the in vitro activity assays, it is necessary to develop in vivo activity assays for the quantification of bioactivity for rhEndostatin.
In vivo antitumoral potency of rhEndostatin After treatment with rhEndostatin at doses of 5, 10, and 20 mg/kg or 10 mmol/L NaAc/HAc buffer daily for 7 continuous days, the tumor-bearing mice were killed. The tumors were then excised and weighed after an additional 3 d following treatment. The analysis was repeated 12 times. The values of the y intersection (a), the slope (b), and correlation coefficient (r) of each regression line were analyzed using the least-squares linear regression analysis. The averaged values were 20.16, 2.42, and 0.98 and the standard deviations were 3.79, 0.39, and 0.02, respectively, suggesting that the potencies determined by the ED50 potency assay were reproducible. The results demonstrated that rhEndostatin significantly inhibited the growth of the H22 tumor and its inhibitory effect was dose-dependent. The tumor inhibition rate and the in vivo potency of rhEndostatin calculated from the corresponding formula are shown in Table 3.
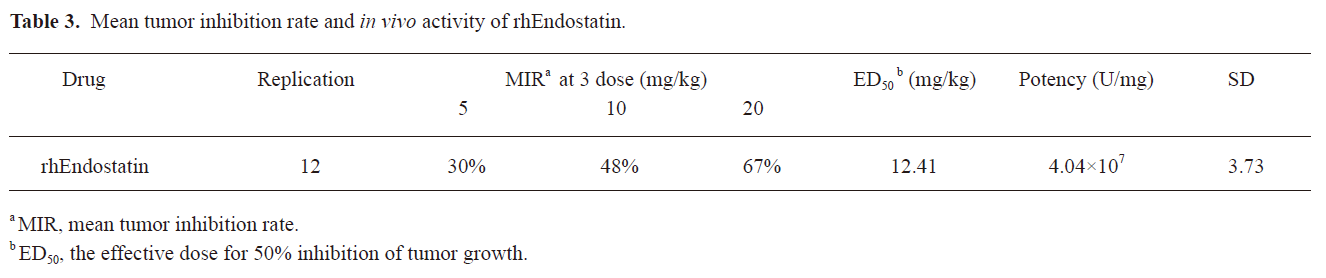
Full table
Pharmacokinetics of rhEndostatin in rhesus monkeys and patients In preclinical trials, the concentration–time curves of rhEndostatin were best fitted to a 2-compartment open model. Following the repeated iv administration of rhEndostatin over a 90 d period (once daily) at the dose of 3 mg/kg, the serum concentration of rhEndostatin in the rhesus monkeys significantly decreased after the injection, with an initial half-life (t1/2α) of 0.293±0.096 h, and a terminal elimination phase (t1/2β) half-life of 6.0±1.7 h. A serum concentration-time profile is shown in Figure 3A. The mean area under the serum concentration–time curve (AUC(0–∞)) was 10.31±1.98 h·µg/mL, Cmax was 5.14±0.88 µg/mL, and the systemic clearance (Cls) was 325±53 mL·kg-1·h-1. No gender difference was found in the pharmacokinetic studies of rhesus monkeys as illustrated in Table 4. The main pharmacokinetic data are shown in Table 5.
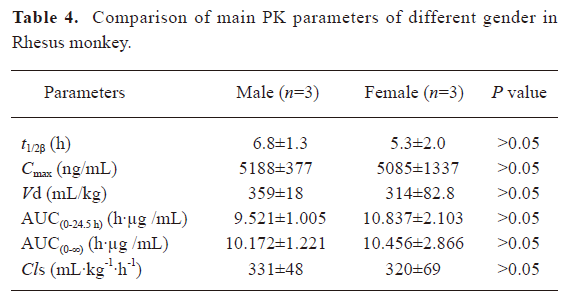
Full table
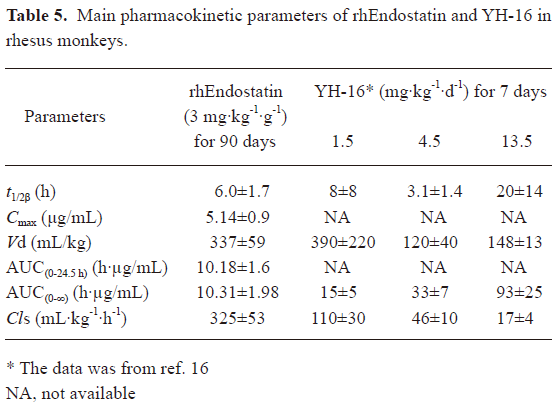
Full table
In clinical trials[31], patients treated with 10 mg·m-2·d-1 rhEndostatin, given as a 2 h iv infusion for 28 d, were included in the pharmacokinetic analysis. A serum concentration-time curve from 0 to 24 h was determined after the administration of the first dose of rhEndostatin, as shown in Figure 3B. The serum concentration of rhEndostatin significantly decreased after the iv injection and returned to baseline level 12 h after the injection. No drug accumulation was found, and the serum concentration of rhEndostatin reached a steady-state level after 7 d of successive administration with the Cavss of 272.44±91.98 ng/mL. The Cmaxss and the Cminss were 861.29±160.25 ng/mL and 166.31±53.36 ng/mL, respectively. The AUCss ranged from 31.76 to 48.88 h·µg/mL. The Vss, mean residence time (MRT), Cls, and t1/2z were 5.96±2.07 L/m2, 105.68±17.87 h, 0.23±0.07 L/h·m2, and 19.56±10.07 h, respectively. The main pharmacokinetic data are shown in Table 6.
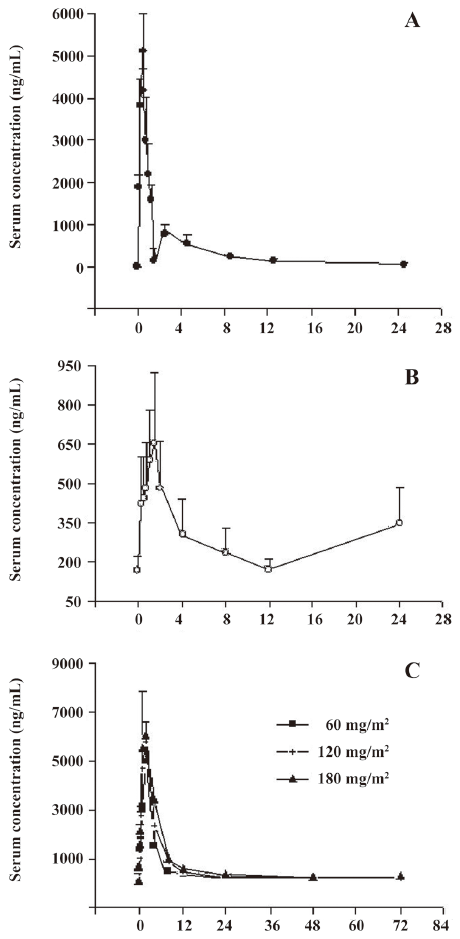
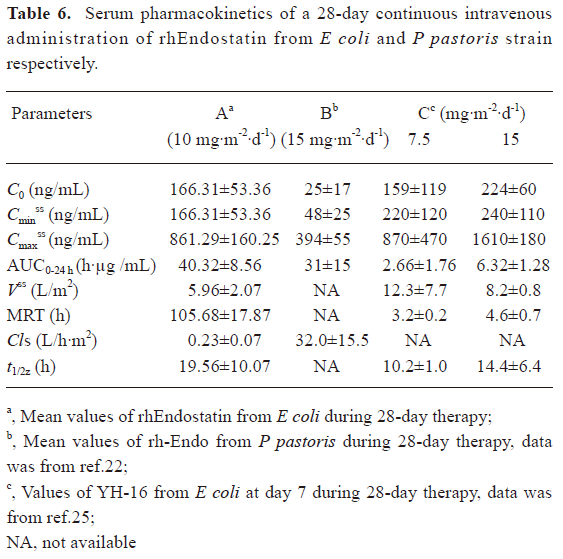
Full table
In the single-administration groups of the clinical trials, baseline endostatin serum concentrations were measured in 24 volunteers. The mean baseline (before rhEndostatin therapy) circulating endostatin concentrations were 126.54±25.04, 110.44±22.82, and 114.41±29.18 ng/mL in the 60, 120, and 180 mg/m2 dose groups, respectively, and showed little interclass difference. Serum concentration-time profiles of rhEndostatin following iv injections in the single-dose group were best fitted to a 2-compartment open model. The results are shown in Figure 3C. After injection, rhEndostatin was rapidly absorbed with the Tmax of 1.79±0.35, 1.75±0.46, and 1.75±0.46 h, respectively. The terminal half-lives (t1/2β) differed dramatically with values of 8.76±19.34, 6.59±9.33, and 26.84±27.19 h, respectively, while the biological half-lives (t1/2z) were 22.87±12.01, 25.11±15.73, and 24.93±17.10 h, respectively, with no significant difference. The Cls were 3.48±1.03, 4.92±2.28, and 5.31±1.73 L/h·m2, respectively, in the 60, 120, and 180 mg/m2 dose groups. The main pharmacokinetic parameters are shown in Table 7. As illustrated in Figure 4, the fact that the Cmax and AUC with different rhEndostatin dosages increased as the dosage increased suggested that the Cmax and AUC were dose-dependent in the single-administration clinical studies.
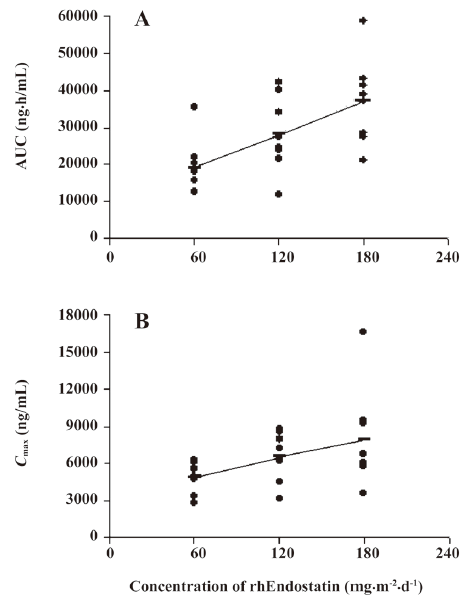
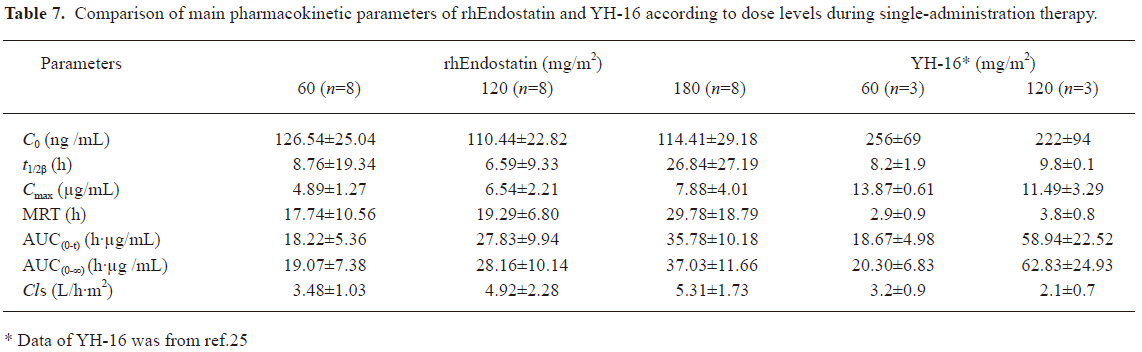
Full table
Immunogenicity of rhEndostatin in rhesus monkeys and patients In the preclinical trials, studies to assess the immunogenicity of rhEndostatin were performed in rhesus monkeys. IgG antibodies to rhEndostatin were not detectable in the sera obtained both from rhesus monkeys in the treated groups before treatment and in the control group during administration. After receiving rhEndostatin for a week, 1 animal in the group received a low-dose rhEndostatin injection (6 mg/kg, n=6), which had positive IgG immunoreactivity with the titer of 1:160. Two animals in the high-dose group (60 mg/kg, n=6) were positive with titers of 1:320. All of the animals in the middle-dose group (20 mg/kg, n=6) showed negative results. The number of animals that showed an immunoreaction to rhEndostatin rapidly increased after 2 weeks of administration. Nearly all of treated groups were positive for IgG immunoreactivity to rhEndostatin during the next 10 weeks with little difference in titers. The administration of rhEndostatin was stopped at d 91 (13 weeks), and the animals showing immunoreactivity and titers in each group began to decrease. Thirteen weeks later, 3 of 6 (50%), 2 of 6 (33.3%), and 1 of 6 (16.7%) animals in the low-, middle-, and high-dose groups showed negative results. Two of 6 (33.3%) and 3 of 6 (50%) in each corresponding group were positive with titers of 1:320; other titers ranged from 1:40 to 1:160. No positive results were obtained 4 weeks after the cessation of administration, with the exception of 1 in the high-dose group which had a low titer of 1:40. The number of positive results in the treated groups at different times is shown in Figure 5A. The data strongly suggest that immunoreactivity of rhesus monkeys to rhEndostatin used in this trial is common.
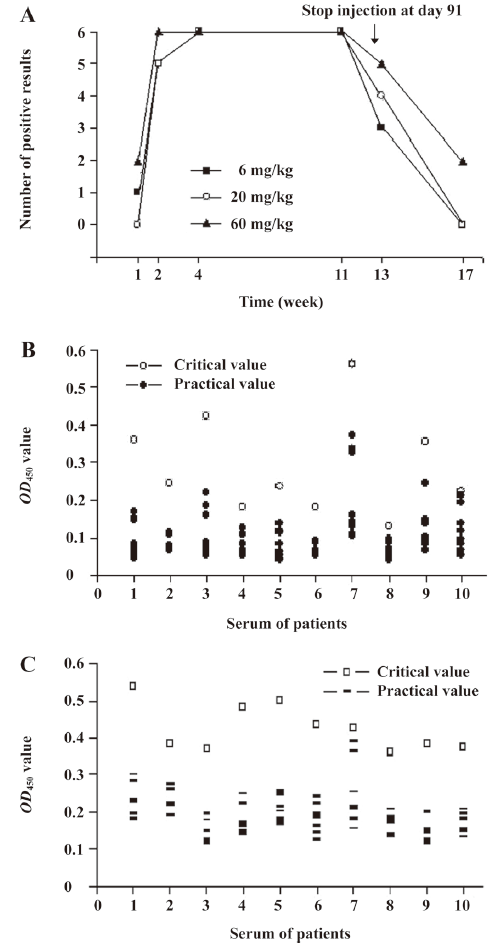
In the clinical trials, IgG and IgM antibodies specific for rhEndostatin were not detectable in the sera obtained from patients before treatment. Sera from 10 patients at d 7, 14, and 28 were assessed for IgG and IgM antibodies to rhEndostatin. As shown in Figure 5B and 5C, the OD450 value in all of the serum samples did not exceed critical value, which was defined as a 2-fold increase of the initial value. The results demonstrated that neither IgG nor IgM antibodies to rhEndostatin were detected, and rhEndostatin had no immunogenicity in this trial.
Discussion
Anti-angiogenetic therapy targeting the internal vas capillary network of tumors may become predominant tumor biotherapy in this century. It is considered to be another effective means for tumor treatment besides conventional surgery, chemotherapy, and radiotherapy[33]. As an angiogenetic inhibitor, endostatin can inhibit the growth or metastasis of tumors by promoting apoptosis of malignant cells and has a definite antitumoral effect without toxicity and drug resistance after prolonged use[6,34]. Therefore, the advantages of endostatin make it a promising candidate for antitumor therapy. It is known that quality assurance is of great important in the biological product preparation process. In the present study, authorized institutions analyzed main parameters of rhEndostatin to make sure that the samples met set standards. For genetically-engineered products, peptide mapping is an important index for evaluating the integrity of the protein structure and the stability of production technology. In addition, we determined other relevant parameters according to Pharmacopoeia of the People’s Republic of China (2005 edition, part Ш) and ensured the safety and availability for the application of rhEndostatin preparations in animals and patients.
At the beginning of the experiments, the bioactivity of rhEndostatin was measured by in vitro inhibition of proliferation or migration of endothelial cells after stimulation by bFGF or VEGF, and the instability of bFGF or VEGF in the culture medium contributed to low sensibility and reproducibility[35,36]. The stability of cells was improved by transfecting the endothelial cells with VEGF or bFGF, which made the in vitro activity assay more convenient, efficient, and rigorous[26]. To gain a more accurate insight into the biological activity of rhEndostatin, animal models, including chick embryo chorioallantoic membrane (CAM) and tumor-bearing models, have been used to assess the therapeutic effectiveness of rhEndostatin[1,14]. However, it was found that CAM has a number of disadvantages, including its limited availability and quantification difficulty since no dose-dependent relationship was observed[37]. Alternatively, many results have revealed that rhEndostatin acts in a significant dose-dependent manner in tumor-bearing mouse models[26,38]. In the present study, the application of HUVEC transfected with VEGF resulted in highly reproducible results and reduced deviations. Three methods were used the in vitro activity assay for comparison. The cell counting method proved to be inconvenient and time costly. The results suggested that the LDH assay was more sensitive with low deviations and high reproducibility. Based on these results, we believe that the LDH assay is a practical method for quantifying the in vitro activity of rhEndostatin. We used H22-tumor-bearing mice to assay the in vivo activity of rhEndostatin, which was repeated 12 times in order to diminish the deviation from individual differences of the animals, concentration of the drugs, and potency of the samples so that the authenticity of the experiments and fidelity of potency was ensured. Due to the fact that the in vitro activity was not always a good predictor for in vivo therapeutic effectiveness, we created a different definition of units. We used the ED50 from our previous study in the present study to ensure the concordance of the in vitro and in vivo bioactivities[29]. The results revealed that the in vitro specific bioactivity (6.4×107, 6.7×107, and 3.8×108 U/mg) was 1.6–9.4 times that of the in vivo antitumoral potency (4.04×107 U/mg). Thus we reached the conclusion that the in vivo and in vitro bioactivities were on the whole concordant, and this method serves as a candidate pharmacological method for the potency assessment of the bioactivities of rhEndostatin in vivo and in vitro.
Based on above results, preclinical trials in rhesus monkeys and a phase I clinical trial were carried out to assess the safety and pharmacokinetic profile of rhEndostatin. We compared main pharmacokinetic parameters of rhEndostatin (Met-endostatin) with that of YH-16 (MGGSHHHHH-endostatin) in both preclinical and clinical trials. The results were dramatically different, although 2 protein products were derived from Escherichia coli. In rhesus monkeys, the results of the pharmacokinetic studies of YH-16 suggested that endostatin tended to accumulate in the body, and that an exorbitant dose (13.5 mg/kg) of endostatin might affect its clearance[16]. The main pharmacokinetic parameters of rhEndostatin at a dose of 3 mg/kg were similar to the results of YH-16 at a dose of 1.5 mg/kg, with the exception that the Cls was much higher (325±53 vs 110±30 mL·kg-1·h-1), as shown in Table 5. The difference of the Cls implied that rhEndostatin may not contribute to drug accumulation. Furthermore, the YH-16 studies show great individual differences while there was little difference between the individuals in our study. In the successive-administration clinical studies, the main pharmacokinetic parameters of rhEndostatin (10 mg·m-2·d-1, 28 d, o.d iv) was compared to P pastoris-derived rhEndostatin (15 mg·m-2·d-1, 28 d, o.d iv) and YH-16 (7.5, 15 mg·m-2·d-1, 28 d, o.d iv), as illustrated in Table 6[22,25]. Interestingly, the difference of the basal endogenous endostatin levels in Chinese (100−300 ng/mL) and American (<50 ng/mL) patients suggest that race disparity exist[22,25,31]. The discrepancy between rh-Endostatin and rhEndostatin was extremely great, many pharmacokinetic parameters such as the MRT, Cls, and Vss resulted in tens, even hundreds times’ differences, except that the AUC0–24 h was close (40.32±8.56 vs 31±15 h·µg/mL). The greatest difference of baseline endostatin levels between Chinese and American patients may be as a result of the significant differences of pharmacokinetic parameters between rhEndostatin and rh-Endostatin. The difference between YH-16 and rhEndostatin was much less: the Cminss (166.31±53.36 vs 220±120 ng/mL) and Cmaxss (861.29±160.25 vs 870±470 ng/mL) of rhEndostatin were very close to those of YH-16 at a dose of 7.5 mg·m-2·d-1. RhEndostatin showed a little lower Vss (5.96±2.07 vs 12.3±7.7 and 8.2±0.8 L/m2) and a little longer t1/2z (19.56±10.07 vs 10.2±1.0 and 14.4±6.4 h), but the AUC0–24 h and MRT of rhEndostatin were much higher than those of YH-16, as shown in Table 6. In the single-administration groups of the clinical trials, different dosages of rhEndostatin had a similar Tmax, because the same delivery route was applied. Despite the different basic endogenous endostatin levels (C0), the t1/2β, AUC(0–t), AUC(0–∞), and Cls were highly similar at the dose of 60 mg/m2, as shown in Table 7. The significant differences in Cmax (4.89±1.27 vs 13.87±0.61 µg/mL) and MRT (17.74±10.56 vs 2.9±0.9 h) suggests that rhEndostatin is released slower than YH-16 and may exert a prolonged action[25]. Moreover, the Cmax and AUC of both rhEndostatin and YH-16 were dose-dependent and showed a much rise in the YH-16 studies as the dose increased.
As for serum antibodies to rhEndostatin, we found that anti-rhEndostatin IgG antibodies generated quickly during administration and decreased rapidly after treatment in rhesus monkeys. We consider the result reasonable because rhEndostatin is a heterogeneous protein. In our clinical studies, neither IgG nor IgM antibodies for rhEndostatin were detected in the patients (n=10). The clinical trial of YH-16 showed that 2 of 20 (10%) patients were positive for IgA immunoreactivity to the rhEndostatin formulation, 1 of 20 (5%) patients was positive for IgA immunoreactivity to His-tag, and 2 of 11 (18%) healthy volunteers were positive for IgG immunoreactivity to rhEndostatin. The immunogenicity of rh-Endostatin was found to be more common with 9 of 13 (69%) patients positive for IgG immunoreactivity to the rh-Endostatin formulation after the first 28 d of treatment. Eight of 11 (73%) patients had positive results after the second 28 d treatment; the data were similar for IgM immunoreactivity[23]. A recent study showed that a natural immune reaction against endostatin can occur in breast cancer patients, and the presence of antibodies to endostatin is not associated with circulating endostatin levels[19].
The difference between rhEndostatin, YH-16, and rh-Endostatin in pharmacokinetic parameters and immunogenicity were significant, as described earlier. First, the difference between rhEndostatin and YH-16 was minimal. The difference between rhEndostatin and rh-Endostatin was much greater. RhEndostatin and YH-16 are derived from Escherichia coli while rh-Endostatin is derived from P pastoris, therefore, different sources may be responsible for the great disparity. Second, no IgG antibodies for endostatin were detected in phase I clinical studies of rhEndostatin, but 18% of volunteers had positive results for IgG immunoreactivity and 5% of patients had positive results for His-tag immunoreactivity in YH-16 clinical trials. The positive rates of IgG and IgM antibodies for rh-Endostatin in patients were both above 60%. Generally, the genecity of antibodies for certain drugs may contribute to drug resistance or pharmacodynamic action attenuation which is closely associated with clinical outcome. The last point refers to the amino sequence. Compared with the amino sequence of rh-Endostatin reported in previous literature[22], Met was added at the N-terminal of the protein in rhEndostatin, an additional 9 amino acid sequence (MGGSHHHHH) was added at the N-terminal of the protein in YH-16[16]. A recent report revealed that approximately 50% of the clinical grade endostatin (rh-Endostatin) molecules lack 4 amino acids at their NH2 termini. The lack of these amino acids gives rise to a molecule that is devoid of zinc, resulting in no antitumoral activity[39]. In contrast, no lack of amino acids in rhEndostatin was found in our trials. This result indicates that our rhEndostatin preparation was more stable and available. Based on the above analysis, we conclude that the expression system, immunoreactivity, and structure of the rhEndostatin protein may be the main reasons contributing to different outcomes of endostatin in clinical trials. Other factors, such as race disparity of basal endogenous endostatin levels, viable therapeutic regimens, and the application of different compartment models, were also included. In conclusion, we have established a method determining the bioactivity of rhEndostatin. We also analyzed the main differences between preclinical and clinical outcomes of different recombinant human endostatin proteins, including rhEndostatin, YH-16 and especially rh-Endo and the pharmacokinetic parameters and immunogenicity. The ongoing phase II trial of rhEndostatin (lot 2007L01486) will help to address some of the questions raised during the current study.
Acknowledgements
We thank Zhong-sheng TONG, Zhao YAN, Yi BA, Shu-fen LI, Ye-hui SHI, Li-hong HE, Xun WANG, Chen WANG, Chen-fen HAO, and the staff of Tianjin Tumor Hospital (Contract Research Organization [CRO]; Tianjin, China). We are also grateful to Zheng-min CHEN, Chang-xiao LIU, Yong ZENG, Yong-ming CAI, Ming LI, Chun-mei CHEN, Feng WANG, Dong-dong ZHANG, the staff of Tianjin Drug Academy (CRO; Tianjin, China), Xiu-hua CHEN, Yu-long YAO, Wen-long REN, Juan WANG, Chang PAN, and the staff of Shanghai Medicine Industry Institute (CRO; Shanghai, China) for their technical assistance.
References
- O’Reilly MS, Boehm T, Shing Y, Fukai N, Vasios G, Lane WS, et al. Endostatin: an endogenous inhibitor of angiogenesis and tumor growth. Cell 1997;88:277-85.
- Ferreras M, Felbor U, Lenhard T, Olsen BR, Delaisse J. Generation and degradation of human endostatin proteins by various proteinases. FEBS Lett 2000;486:247-51.
- Zhang P, Wu D, Ge J, Zhu Z, Feng G, Yue T, et al. Experimental inhibition of corneal neovascularization by endostatin gene transfection in vivo. Chin Med J (Engl) 2003;116:1869-74.
- Dhanabal M, Ramchandran R, Volk R, Stillman IE, Lombardo M, Iruela-Arispe ML, et al. Endostatin: yeast production, mutants, and antitumor effect in renal cell carcinoma. Cancer Res 1999;59:189-97.
- Bloch W, Huggel K, Sasaki T, Grose R, Bugnon P, Addicks K, et al. The angiogenesis inhibitor endostatin impairs blood vessel maturation during wound healing. FASEB J 2000;14:2373-6.
- Boehm T, Folkman J, Browder T, O’Reilly MS. Antiangiogenic therapy of experimental cancer does not induce acquired drug resistance. Nature 1997;390:404-7.
- Yin G, Liu W, An P, Li P, Ding I, Planelles V, et al. Endostatin gene transfer inhibits joint angiogenesis and pannus formation in inflammatory arthritis. Mol Ther 2002;5:547-54.
- Pia N, Pia H, Timo S, Jani L, Ritva H, Ulf-Hakan S, . Endostatin inhibits human tongue carcinoma cell invasion and intravasation and blocks the activation of matrix metalloprotease-2, -9, and -13. J Biol Chem 2003; 278: 22 404–11.
- Skovseth DK, Veuger MJ, Sorensen DR, Angelis PM, Haraldsen G. Endostatin dramatically inhibits endothelial cell migration, vascular morphogenesis, and perivascular cell recruitment in vivo. Blood 2005;105:1044-51.
- Davis DW, Shen Y, Mullani NA, Wen S, Herbst RS, O’Reilly M, et al. Quantitative analysis of biomarkers defines an optimal biological dose for recombinant human endostatin in primary human tumors. Clin Cancer Res 2004;10:33-42.
- Sorensen DR, Read TA, Porwol T, Olsen BR, Timpl R, Sasaki T, et al. Endostatin reduces vascularization, blood flow, and growth in a rat gliosarcoma. Neuro-oncol 2002;4:1-8.
- Park JH, Chang KH, Lee JM, Lee YH, Chung IS. Optimal production an in vitro activity of recombinant endostatin from stably transformed Drosophila melanogaster S2 cells. Cell Dev Biol Anim 2001;37:5-9.
- Sim BK, MacDonald NJ, Gubish ER. Angiostatin and endostatin: Endogenous inhibitors of tumor growth. Cancer Metastasis Rev 2000;19:181-90.
- Huang XJ, Wong MK, Zhao Q, Zhu ZY, Wang KZ, Huang N, et al. Soluble recombinant endostatin purified from Escherichia coli: antiangiogenic activity and antitumor effect. Cancer Res 2001;61:478-81.
- Sim BK, Fogler WE, Zhou XH, Liang H, Madsen JW, Luu K, et al. Zinc ligand-disrupted recombinant human endostatin: Potent inhibition of tumor growth, safety and pharmacokinetic profile. Angiogenesis 1999;3:41-51.
- Song HF, Liu XW, Zhang HN, Zhu BZ, Yuan SJ, Liu SY, et al. Pharmacokinetics of His-tag recombinant human endostatin in rhesus monkeys. Acta Pharmacol Sin 2005;26:124-8.
- Wang JW, Sun Y, Liu YY, Yu QT, Zhang QP, Li K, et al. Results of randomized, multicenter, double-blind phase Ш trial of rh-endostatin (YH-16) in treatment of advanced non-small cell lung cancer patients. Chi J Lung Cancer 2005;8:283-90.
- Kulke MH, Bergsland EK, Ryan DP, Enzinger PC, Lynch TJ, Zhu AX, et al. Phase II study of recombinant human endostatin in patients with advanced neuroendocrine tumors. J Clin Oncol 2006;24:3555-61.
- Bachelot T, Ratel D, Menetrier-Caux C, Wion D, Blay JY, Berger F. Autoantibodies to endostatin in patients with breast cancer: correlation to endostatin levels and clinical outcome. Br J Cancer 2006;94:1066-70.
- Herbst RS, Mullani NA, Davis DW, Hess KR, McConkey DJ, Charnsangavej C, et al. Development of biologic markers of response and assessment of antiangiogenic activity in a clinical trial of human recombinant endostatin. J Clin Oncol 2002;20:3804-14.
- Hansma AHG, Broxterman HJ, van der Horst I, Yuana Y, Boven E, Giaccone G, et al. Recombinant human endostatin administered as a 28-day continuous intravenous infusion, followed by daily subcutaneous injections: a phase I and pharmacokinetic study in patients with advanced cancer. Ann Onc 2005;16:1695-701.
- Eder JP, Supko JG Jr, Clark JW, Puchalski TA, Garcia-Carbonero R, Ryan DP, et al. Phase I clinical trial of recombinant human endostatin administered as a short intravenous infusion repeated daily. J Clin Oncol 2002;20:3772-84.
- Herbst RS, Hess KR, Tran HT, Tseng JE, Mullani NA, Charnsangavej C, et al. Phase I study of recombinant human endostatin in patients with advanced solid tumors. J Clin Oncol 2002;20:3792-803.
- Thomas JP, Arzoomanian RZ, Alberti D, Marnocha R, Lee F, Friedl A, et al. Phase I pharmacokinetic and pharmacodynamic study of recombinant human endostatin in patients with advanced solid tumors. J Clin Oncol 2003;21:223-31.
- Yang L, Wang JW, Tang ZM, Liu XW, Huang J, Li ST, et al. A phase I clinical trial for recombinant human endostatin. Chin J New Drugs 2004;13:548-53.
- Zhang HY, Han QW, Xu YF, Fan YR, Dai QM, Xu JM, et al. The methods for determining the purity and in vitro or in vivo activity of recombinant human endostatin. Cancer Biol Ther 2005;4:207-12.
- The State Pharmacopoeia Committee of the People’s Republic of China. Pharmacopoeia of the People’s Republic of China, part III, 2005 ed. Beijing: Chemical Industry Press; 2005.
- Lee Sim BK, O'Reilly MS, Liang H, Fortier AH, He WX, Madsen JW, et al. A recombinant human angiostatin protein inhibits experimental primary and metastatic cancer. Cancer Res 1997;57:1329-34.
- Xu YF, Zhu LP, Hu B, Rong ZG, Zhu HW, Xu JM, et al. A quantitative method for assessing the antitumor potency of human recombinant endostatin. Eur J Pharmacol 2007;564:1-6.
- Wei YQ, Wang QR, Zhao X, Yang L, Tian L, Lu Y, et al. Immunotherapy of tumors with xenogeneic endothelial cells as a vaccine. Nat Med 2000;6:1160-6.
- Tong ZS, Yan Z, Ba Y, Li SF, Shi YH, He LH, et al. Phase I clinical study of recombinant human endostatin. J Chin Modern Med 2006;3:1150-5.
- Sasaki T, Fukai N, Mann K, Gohring W, Olsen BR, Timpl R. Structure, function and tissue forms of the C-terminal globular domain of collagen XVIII containing the angiogenesis inhibitor endostatin. EMBO J 1998;17:4249-56.
- Abdollahi A, Hlatky L, Huher P. Endostatin: the logic of anti-angiogenic therapy. Drug Resist Updat 2005;8:59-74.
- Yamaguchi N, Anand-Apte B, Lee M, Sasaki T, Fukai N, Shapiro R, et al. Endostatin inhibits VEGF-induced endothelial cell migration and tumor growth independently of zinc binding. EMBO J 1999;18:4414-23.
- Jung SP, Siegrist B, Hornick CA, Wang YZ, Wade MR, Anthony CT, et al. Effect of human recombinant endostatin protein on human angiogenesis. Angiogenesis 2002;5:111-8.
- Wang YS, Eichler W, Friedrichs U, Yafai Y, Hoffmann S, Yasukawa T, et al. Impact of endostatin on bFGF-induced proliferation, migration, and matrix metalloproteinase-2 expression/secretion of bovine choroidal endothelial cells. Curr Eye Res 2005;30:479-89.
- McCarty MF, Baker CH, Bucana CD, Fidler IJ. Quantitative and qualitative in vivo angiogenesis assay. Int J Oncol 2002;21:5-10.
- Li Q, Olsen BR. Increased angiogenic response in aortic explants of collagen XVIII/endostatin-null mice. Am J Pathol 2004;165:415-24.
- Lee TY, Tjin Tham Sjin RM, Movahedi S, Ahmed B, Pravda EA, Lo KM, et al. Linking antibody Fc domain to endostatin significantly improves endostatin half-life and efficacy. Clin Cancer Res 2008;14:1487-93.