Expression of interleukin-6 is downregulated by 17-(allylamino)-17-demethoxygeldanamycin in human prostatic carcinoma cells1
Introduction
The receptor of the interleukin (IL)-6 family of cytokines, gp130, has been identified in prostate tissue and prostate carcinoma cells[1,2]. Alterations in the expression to growth factors/cytokines may contribute to prostate cancer development and the androgen-independent phenotype[3]. The increase of serum cytokines, including IL-6, was found in cachectic patients with prostate carcinoma. Immunostaining levels of IL-6 and its receptor increased with Gleason grade[1,4]. Several studies have revealed that plasma IL-6 levels have prognostic significance in patients with metastatic hormone-refractory prostate cancer[5]. Those studies suggested that high levels of circulating IL-6 are correlated with advanced prostate cancer. IL-6 signaling can activate the androgen receptor in a ligand-independent manner and may play an important functional role in hormone-refractory prostate cancer progression[3,6]. In vitro studies have found that IL-6 is considered a positive growth factor for most prostate cells. On the contrary, IL-6 caused growth arrest and induced differentiation in LNCaP, androgen-sensitive prostate carcinoma cells, although IL-6 treatment accelerated the growth of chronic IL-6-treated LNCaP cells[7].
Heat shock proteins were originally identified as a group of proteins inducible by heat shock. It was also discovered that heat shock proteins could also be induced by some stimuli, such as growth factors, inflammation, and infection[8]. Heat shock protein 90 (Hsp90), which promotes the attainment and maintenance of proper conformation of its client protein, is an essential protein for all eukaryotes. Over hundreds of proteins have been reported to be the client protein of Hsp90, such as steroid hormone receptor, growth factor receptor, Akt serine/threonine and tyrosine protein kinases, and transcription factors[9]. Hsp90 also functions in multiple oncogenic pathways in prostate cancer, and multiple Hsp90inhibitors have also been applied to prostate cancer therapy[10,11].
Previous studies have indicated that elevated IL-6 levels in the serum of patients with systemic lupus erythematosus induce elevated levels of Hsp90 protein in peripheral blood mononuclear cells[12]. In addition, a recent study using tissue microarray revealed a positive correlation between Hsp90 and IL-6 in the epithelium and stroma of intra-epithelial prostatic neoplasia and prostatic carcinoma[13]. These studies suggested that an interregulation between Hsp90 and IL-6 expression. The objectives of our study were to determine the regulatory mechanisms of Hsp90 and Hsp90 inhibitor, 17-(allylamino)-17-demethoxygeldanamycin (17AAG), on the gene expression of IL-6 in human prostatic carcinoma cells (PC-3).
Materials and methods
Cell culture and chemicals The PZ-HPV-7, CA-HPV-10, LNCaP, PC-3, and DU145 cell lines were collected and maintained as described before[14]. 17AAG and phorbol 12-myristate 13-acetate (PMA) were purchased from Sigma (St Louis, MO, USA). The antihuman IL-6 monoclonal antibody (MAB2061) was purchased from R&D Systems (Minneapolis, MN, USA). Fetal bovine serum (FBS) was purchased from HyClone (Logan, UT, USA). All culture media and reagents were purchased from Life Technologies (Rockville, MD, USA). The cells were cultured in RPMI-1640 containing 10% FBS, and the medium was replaced twice a week.
RT–PCR The total RNA was isolated with TRIzol reagent, and cDNA was synthesized by using the superscript III pre-amplification system as described before[15]. The expression of multiple cytokine genes (TNF-α, IL-1β, IL-6, and IL-8) were determined by a multiplex polymerase chain reaction (MPCR) kit for human sepsis cytokines set (Maxim Biotech, San Francisco, CA, USA). Primers were used for the amplification of sequences specific to human IL-6 [5´-AAAGAGGCACTGGCAGAAAA-3´ (sense) and 5´-CATGCTACATTTGCCGAAGA-3´ (antisense)], IL-1β [5´-TTCGACACATGGGATAACGA-3´ (sense) and 5´-TCTTTCAACACGCAGGACAG-3´ (antisense)], and IL-6 receptor (IL-6R; [5´-AAAGAGGCACT-GGCAGAAAA-3´ (sense) and 5´-CATGCTACATTT-GCCGAAGA-3´ (antisense)] mRNA. The cDNA quality was verified by performing controlled reactions by using primers derived from β-actin-P and β-actin-R [5´-GAAGATCAAGATCATTGCTCCTCC-3´ (sense) and 5´-CTGT GCTCAAGTCAGTGTACAGG-3´ (antisense)]. The PCR reaction was carried out in a thermal cycler (Thermolyne, Dubuzue, IA, USA) and the parameters were as follows: 30 cycles of 94 °C for 0.5 min, 55 °C for 1 min, and 72 °C for 1 min. The PCR products were separated by 2% agarose gel electrophoresis and visualized by ethidium bromide staining.
Cell proliferation assay The cell proliferation of PC-3 cells in response to 17AAG or PMA was measured by the conversion of a MTS tetrazolium salt into a formazan product by viable cells and absorbance at 490 nm using the cell Titer96 Aqueous cell proliferation assay as described before[15]. In this assay, the cells (5000 cells/well) were subcultured to each well of a 96-well plate with 100 µL RPMI-1640 medium with 10% FBS and different treatments as indicated. After 24 or 48 h, the cells were washed twice with phosphate-buffered saline (PBS), and the reaction was stopped by adding the MTS and PMS reagent (20:1) into 100 μL PBS. The number of cells in each well was counted using an ELISA microplate reader (Dynex Technologies, Chantilly, VA, USA) after 3 h incubation at 37 °C.
Reporter vector constructs The control reporter vector (ppGL3), which contains the SV40 promoter, was purchased from Promega Biosciences (San Luis Obispo, CA, USA). The DNA fragment containing the enhancer/promoter of the IL-6 gene was isolated from the BAC clone (RP11-240H8; Invitrogen, Carlsbad, CA, USA). The BAC clone was digested with XhoI, and then 1 2550 bp DNA fragment, which was subcloned into the pGL3 reporter vector (Promega Biosciences, USA), was sequenced and identified as the 5´ flanking region of the human IL-6 gene (–2541 to +8) and termed pIL6X. The gene maps of the human IL-6 reporter vectors (pIL6SX: –2171 to +8, pIL6B: –1536 to +8, and pIL6NH: –226 to +8) are shown in Figure 1A. The pIL6NHS (–149 to +8) was synthesized from pIL-6NH by PCR using the GL2 oligonucleotide primer (Promega Biosciences, USA) and primer 5´-GAGTCTTAATAAGGTTTCCAATCAG-3´. The DNA fragment was ligated into the pGEMTeasy vector and then digested and cloned into pGL3 at the SacI and HindIII sites. The reporter vector (pIL6NFm), containing the mutant NF-κB response element, was constructed by the QuikChange site-directed mutagenesis kit (Stratagene, La Jolla, CA, USA) using complementary double-stranded primers 5´- GATTTATCAAATGT
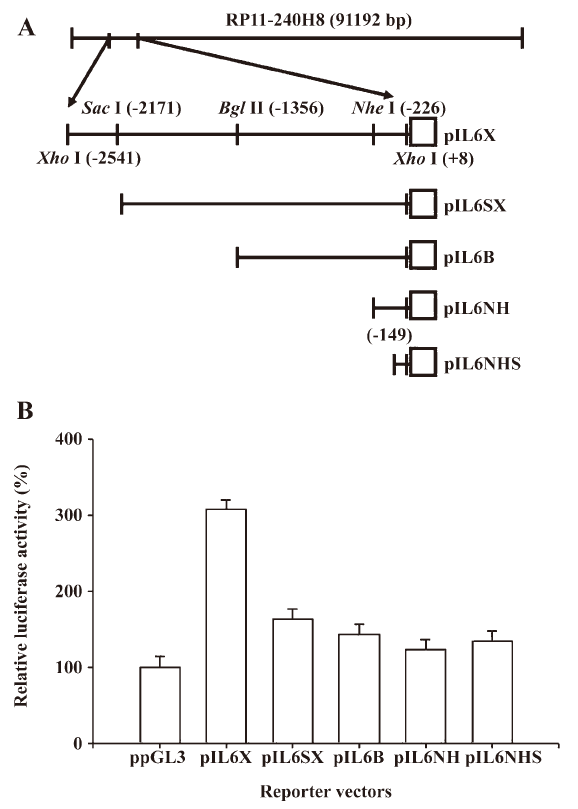
Transient transfections and reporter assay The PC-3 cells were plated onto 24-well plates at 1×104 cells/well 1 day prior to transfection. Cells were transiently transfected using TransFast transfection reagent (0.6 µg/well; Promega Biosciences, USA) with 1 µg/well reporter vector and 0.5 µg/well pCMVSPORTβgal (Life Technologies, USA) for 4 h. The cells were then incubated in RPMI-1640 medium with 10% FBS overnight. The transfected cells were treated with RPMI-1640 medium with 10% FBS and different concentrations of drugs as indicated for an additional 16 h. The cell extracts were assayed for the luciferase activity and for the β-galactosidase (β-GAL) activity, as described by the manufacturer (Promega Biosciences, USA). The activity of luciferase was determined using a LumiCount microplate reader (Packard Bioscience, Meriden, CT, USA) and was adjusted according to the β-GAL enzymatic activity as previously described[15].
ELISA The PC-3 cells were incubated with 1 mL of RPMI-1640 medium and PMA or 17AAG, respectively, as indicated, in a 24-well-plate (2×104 cells/well) for 36 h. Following incubation, the supernatants from each well were collected for IL-6 assays. Cell pellets were washed twice with ice-cold PBS and then dissolved in 500 μL PBS. After sonication for 10 s, the cell extracts were centrifuged at 23 000×g for 20 min. The IL-6 levels in 20 µL culture media were measured by IL-6 ELISA, as described by the manufacturer (PeproTech, London, UK). The IL-6 level in each sample was adjusted by the concentrations of protein in the whole cell extract, which was measured using a BCA protein assay kit (Pierce, Rockford, IL, USA).
Statistical analysis Results are expressed as the mean±SEM of at least 3 independent replications of each experiment. Statistical significance was determined by paired t-test analysis using SigmaStat software for Windows (version 2.03; SPSS, Chicago, IL, USA).
Results
In order to investigate the gene regulation of human IL-6, we constructed a series of human IL-6 reporter vectors from a BAC clone (RP11-240H8) and determined their reporter activities. The results of the 5´ deletion transient gene expression assay indicated that the pIL6NHS reporter vector still expressed almost the same amount of reporter activity as the ppGL3 vector, which contained the SV40 promoter. The promoter of the human IL-6 gene in the PC-3 cell line was found to be located within 149 bp upstream of the transcriptional initiation site (Figure 1B).
The results from multiple PCR indicated that both IL-6 and IL-8 were expressed in advanced prostatic carcinoma cells, PC-3 and DU145, but not in the HPV-7-PZ, HPV-10-CA, and LNCaP prostatic cells. The expression of TNF-α was found in the HPV-7-PZ, HPV-10-CA, and PC-3 cells (Figure 2A). However, there was little IL-1β expression found in all of the prostate cells. Therefore, we repeated the RT–PCR assay using the specific primers for IL-1β. The results confirmed that the IL-1β expression in the prostate cell lines was similar to that of TNF-α; the LNCaP and DU145 cells were found to have lower expression than other cells (Figure 2B). We also determined the expression of the IL-6R using RT–PCR with a pair of specific primers. The results showed that all of the cells used in this study expressed IL-6R in different amounts (Figure 2B). When the PC-3 cells were treated with 17AAG (0.5 and 1 μmol/L), the RT–PCR indicated that 17AAG specifically downregulated the gene expression of IL-6, but not that of IL-6R (Figure 2C).
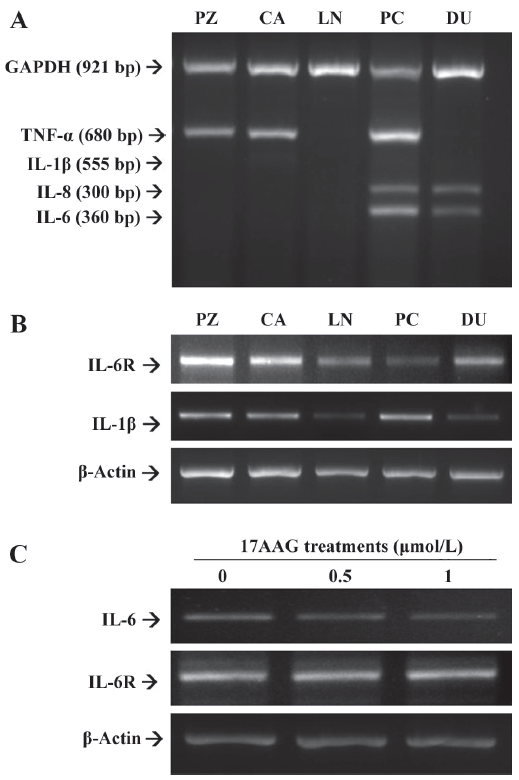
We used the MTS assay to determine the cell proliferation of the PC-3 cells after 17AAG and PMA treatments. The PMA treatments significantly (P<0.05) induced 1.5- to 2-fold of cell proliferation only when the cells were treated with 80–160 nmol/L PMA for 48 h. However, only 10%–20% of cell proliferation was upregulated after 48 h incubation when the cells were treated with 40 nmol/L PMA (Figure 3A). 17AAG significantly (P<0.05) affected approximately 10%–25% of the cell proliferation when the treatment was used up to 1 μmol/L with incubation for 48 h (Figure 3B). We also determined whether PMA-enhanced cell proliferation was due to the effect of the IL-6 secretion. The MTS assay revealed that PMA treatment (160 nmol/L) for 48 h induced cell proliferation 2-fold, but IL-6 MAB treatment for 48 h downregulated cell proliferation by 40%. However, the IL-6 antibody treatment blocked the stimulation of PMA treatment on cell proliferation (Figure 3C). In order to eliminate the factors affecting cell proliferation on IL-6 synthesis, 0.5 μmol/L 17AAG and 40 nmol/L PMA treatments were used in the following experiment. In in vitro studies, ELISA indicated that 0.5 μmol/L 17AAG treatment blocked 20% of IL-6 secretion in the PC-3 cells, but 40 nmol/L PMA treatment enhanced it by 220%. Moreover, 17AAG treatment blocked the stimulation of PMA treatment on IL-6 synthesis (Figure 3D).
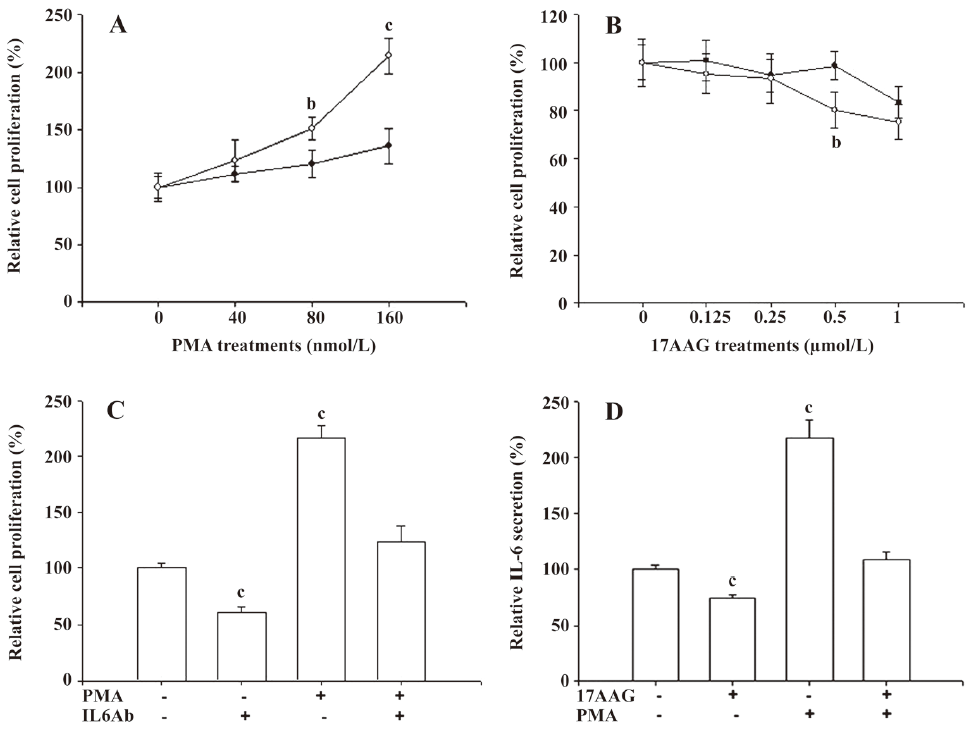
The transient gene expression assay revealed that 17AAG decreased the IL-6 gene expression. When the IL-6 reporter vector-transfected PC-3 cells were treated with 0.25–1 μmol/L 17AAG for 24 h, reporter activity was blocked by 60% compared to the control-treated group (Figure 4A). The transient gene expression assay also revealed that PMA induced IL-6 promoter activity 2- to 3-fold (Figure 4B). Further reporter assays also revealed that 0.5 μmol/L 17AAG blocked the stimulation of PMA on IL-6 promoter activity (Figure 4C).
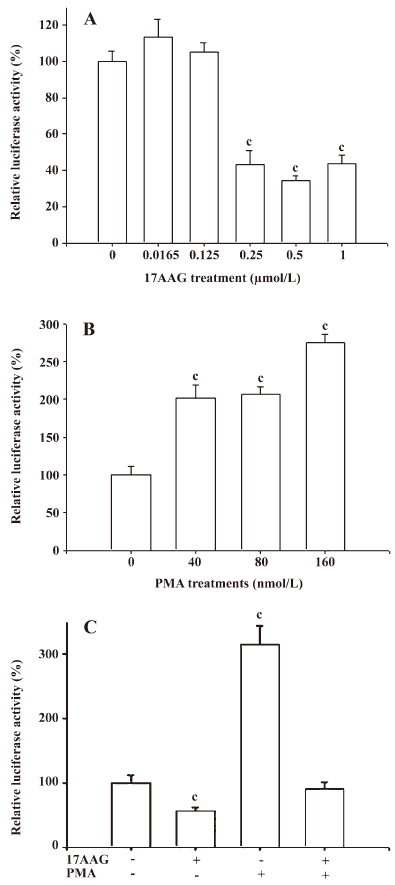
The results from the 5´ deletion reporter assay using the pIL6X, pIL6SX, pIL6B, pIL6NH, and pIL6NHS reporter vectors indicated that the response element for the effects of 17AAG on IL-6 promoter activity was located at –149 to +8 of the 5´ flanking region of the human IL-6 gene (Figure 5A). Since the enhancement of PMA on the IL-6 expression is dependent on the NF-κB binding site, we continue to determine whether the deregulation of 17AAG on IL-6 gene expression is also dependent on the NF-κB signal pathway. The PC-3 cells were cotransfected with IL-6 reporter vector pIL6NHS containing the NF-κB response element and the super repressor IκBα (S32A/S36A), a dominant negative inhibitor of the NF-κB overexpression vector. The results of the transient gene expression assays showed that the overexpression of the IκBα vector blocked the NF-κB nuclear translocation and decreased promoter activities. However, the reporter activity was not affected by the forced overexpression of the IκBα vector when the NF-κB binding site was mutated from AAATGTGGGATTTTCCC to AAATGTTACATTTTCCC by site-directed mutagenesis (Figure 5B). The results from the 5´ deletion and site-directed mutagenesis reporter assay revealed that the effects of PMA appeared to be through the NF-κB pathway. When the NF-κB response element of the IL-6 promoter was mutated, the stimulation of PMA on IL-6 promoter activity was blocked (Figure 5C). On the contrary, 17AAG still downregulated the gene expression of IL-6 reporter activity, even when the NF-κB binding site on the IL-6 promoter was mutated (Figure 5D).
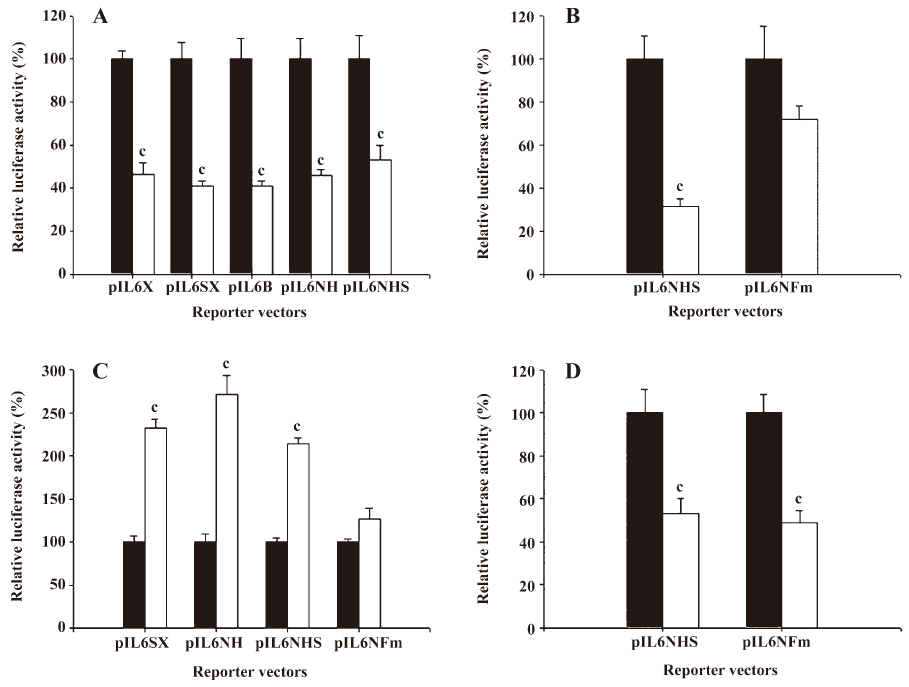
Discussion
IL-6 is a member of the gp130 family of cytokines. In vitro and in vivo studies have indicated that IL-6 accelerates growth in human prostate cancer[7,16]. Several studies have revealed that circulating IL-6 levels or IL-6R in the solid tumor has prognostic significance in patients with metastatic hormone-refractory prostate cancer[1,13,17]. Our in vitro study revealed that only advanced prostate carcinoma cells (PC-3 and DU145) express IL-6 and IL-8. However, IL-6R is ubiquitously expressed in all 5 prostate epithelium cells. These results are in agreement to prior experiments performed in other laboratories[1,2,18]. Although our study indicated that the expression of TNF-α was found in the HPV-7-PZ, HPV-10-CA, and PC-3 cells, an early study indicated that none of the prostate cell lines secreted detectable quantities of TNF-α[19]. The expression of IL-1β was found in PC-3 cells, but not in LNCaP and DU145 cells, as reported in a previous study[20].
An immunohistochemical study indicated that prostatic epithelium and stroma both expressed IL-6; however, IL-6 expression increased in the epithelium and decreased in the stroma as the prostatic malignancy progressed[13]. Moreover, results from in vitro studies have indicated that IL-6 is produced by androgen-independent prostate cancer cells and acts in an autocrine and/or paracrine manner to stimulate their growth, suppressing the growth of androgen-dependent cells[7]. In cell cultures or xenografts in nude mice, anti-IL-6 MAB treatment induces cell apoptosis and tumor regression[21].
Previous reports have indicated that IL-6 serum levels are positively correlated to the Hsp90 levels of peripheral blood mononuclear cells in systemic lupus erythematosus patients[12]. Hsp90 is critical in the intracellular signaling pathway that promotes inflammatory cytokine biosynthesis and prognostic value in some tumors. Moreover, IL-6 and Hsp90 seem to play an important role in the survival response of cancer exposed to the toxic effects of chemotherapy or radiotherapy[22]. A recent study has suggested a positive correlation between Hsp90 and IL-6 in the epithelium and stroma of intra-epithelial prostatic neoplasia and prostatic carcinoma[5,13]. The present study is the first report indicating that Hsp90 inhibitor blocked IL-6 secretion and attenuated cell proliferation of advanced prostate carcinoma cells in vitro.
17AAG is a benzoquinone antibiotic that downregulates oncoproteins by binding specifically to the cytosolic chaperone protein, Hsp90. 17AAG also inhibits Hsp90-mediated conformational folding and promotes the degradation of oncoproteins[23]. Preclinical studies have indicated that 17AAG could be beneficial in combination therapy with other chemotherapeutic agents in advanced prostate cancer[10].
Other studies have also found that Hsp90 inhibitor, geldanamycin, not only inhibits the production of IL-6 in activated macrophage, but also suppresses IL-6-induced gene expression in hepatoma cells[24]. However, there is no direct evidence to support the interregulation between IL-6 and Hsp90 in prostate carcinoma cells. The results using RT–PCR and the transient gene expression assay suggest that 17AAG downregulated the gene expression of IL-6, but not IL-6R. By using the RT–PCR, ELISA, and transient gene expression assays, we found that Hsp90 inhibitor decreased the IL-6 gene expression at the transcriptional level.
The promoter of the human IL-6 gene contains potential binding sites for a number of transcription factors, such as AP-1, cAMP response element binding protein, CCAAT enhancer-binding proteins, and NF-κB, which is known to participate in the induction of IL-6 gene expression in prostate carcinoma cells[25]. Previous studies have indicated that the induction of IL-6 gene expression in the prostate cells by PMA is dependent on the binding of the NF-κB in the promoter of IL-6 gene[26,27]. The results are in agreement with our present report. In the present study, the transient gene expression with the 5´ deletion reporter assay suggested that 17AAG-downregulated reporter activity was dependent on the 5´ flanking region (–149 to +8) of IL-6 gene. It is known that many proteins are reported to be the client protein of Hsp90[9]. However, the client proteins involved in the modulation of the IL-6 promoter has yet to be identified. The CCAAT/enhancer-binding protein, NF-κB, and GATA-1 binding sites were previously identified within the promoter region of IL-6 gene[25,26]. Our results showed that even when the NF-κB binding site was mutated, 17AAG still downregulated the gene expression of IL-6. These data suggest that although 17AAG treatment blocked the stimulation of PMA on IL-6 expression, 17AAG-modulated IL-6 expression is dependent on the region of proximal basic promoter (–149 to +8), but is not related to the NF-κB signal pathway.
References
- Royuela M, Ricote M, Parsons MS, Garcia-Tunon I, Paniagua R, De Miguel MP. Immunohistochemical analysis of the IL-6 family of cytokines and their receptor in benign, hyperplasic and malignant human prostate. J Pathol 2004;202:41-9.
- Palmer J, Hertzog PJ, Hammacher A. Differential expression and effects of gp130 cytokines and receptors in prostate cancer cells. Int J Biochem Cell Biol 2004;36:2258-69.
- Edwards J, Bartlett JMS. The androgen receptor and signal-transduction pathways in hormone-refractory prostate cancer. Part 2: androgen-receptor cofactors and bypass pathways. BJU Int 2005;95:1327-35.
- Pfitzenmaier J, Vessella R, Higano CS, Noteboom JL, Wallace D Jr, Corey E. Elevation of cytokine levels in cachectic patients with prostate carcinoma. Cancer 2003;97:1211-6.
- George DJ, Halabi S, Shepard TF, Sanford B, Vogelzang NJ, Small EJ, et al. The prognostic significance of plasma interleukin-6 levels in patients with metastatic hormone-refractory prostate cancer: results from cancer and leukemia group B 9480. Clin Cancer Res 2005;11:1815-20.
- Jia L, Choong CS, Ricciardelli C, Kim J, Tilley WD, Coetzee GA. Androgen receptor signaling: mechanism of interleukin-6 inhibition. Cancer Res 2004;64:2619-26.
- Culig Z, Steiner H, Bartsch G, Hobisch A. Interleukin-6 regulation of prostate cancer cells growth. J Cell Biochem 2005;95:497-505.
- Ritossa F. Discovery of the heat shock response. Cell Stress Chaperones 1996;1:97-8.
- Cullinan SB, Whitesell L. Heat shock protein 90: a unique chemotherapeutic target. Semin Oncol 2006;33:457-65.
- Lattouf JB, Srinivasan R, Pinto PA, Linehan WM, Neckers L. Mechanisms of disease: the role of heat-shock protein 90 in genitourinary malignancy. Nat Clin Pract Urol 2006;3:590-600.
- Solit DB, Scher H, Rosen N. Hsp90 as a therapeutic target in prostate cancer. Semin Oncol 2003;30:709-16.
- Ripley BJM, Isenberg DA, Latchman DS. Elevated levels of the 90 kDa heat shock protein (hsp90) in SLE correlate with levels of IL-6 and autoantibodies to hsp90. J Autoimmun 2001;17:341-6.
- Cardillo MR, Ippoliti F. IL-6, IL-10 and HSP90 expression in tissue microarrays from human prostate cancer assessed by computer-assisted image analysis. Anticancer Res 2006;26:3409-16.
- Tsui KH, Hsieh WC, Lin MH, Chang PL, Juang HH. Triiodothyronine modulates cell proliferation of human prostatic carcinoma cells by downregulation of the B-cell translocation gene 2. Prostate 2008;68:610-9.
- Tsui KH, Chang PL, Juang HH. Manganese antagonizes iron blocking mitochondrial acontiase expresson in human prostate carcinoma cells. Asian J Androl 2006;8:307-16.
- Steiner H, Godoy-Tundidor S, Rogatsch H, Berger AP, Fuchs D, Comuzzi B, et al. Accelerated in vivo growth of prostate tumors that up-regulate interleukin-6 is associated with reduced retinoblastoma protein expression and activation of the mitogen-activated protein kinase pathway. Am J Pathol 2003;162:655-63.
- George DJ, Halabi S, Shepard TF, Sanford B, Vogelzang NJ, Small EJ, et al. The prognostic significance of plasma interleukin-6 levels in patients with metastatic hormone-refractory prostate cancer: results from cancer and leukemia group B 9480. Clin Cancer Res 2005;11:1815-20.
- Araki S, Omori Y, Lyn D, Singh RK, Meinbach DM, Sandman Y, et al. Interleukin-8 is a molecular determinant of androgen independence and progression in prostate cancer. Cancer Res 2007;67:6854-62.
- Chung TDK, Yu JJ, Spiotto MT, Bartkowski M, Simons JW. Characterization of the role of IL-6 in the progression of prostate cancer. Prostate 1999;38:199-207.
- Abdul M, Hoosein N. Differences in the expression and effects of interleukin-1 and 2 on androgen-sensitive and -insensitive human prostate cancer cell lines. Cancer Let 2000;149:37-42.
- Steiner H, Cavarretta IT, Moser PL, Berger AP, Bektic J, Dietrich H, et al. Regulation of growth of prostate cancer cells selected in the presence of interleukin-6 by the anti-interleukin-6 antibody CNTO326. Prostate 2006;66:1744-52.
- Harashima K, Akimoto T, Nonaka T, Tsuzuki K, Mitsuhashi N, Nakano T. Heat shock protein (90) chaperon complex inhibitor radiciol, potentiated radiation-induced cell killing in a hormone-sensitive prostate cancer cell line through degradation of androgen receptor. Int J Radiat Biol 2005;81:63-76.
- Goetz MP, Toft DO, Ames MM, Erlichman C. The Hsp90 chaperone complex as a novel target for cancer therapy. Ann Oncol 2003;14:1169-76.
- Wax S, Piecyk M, Maritim B, Anderson P. Geldanamycin inhibits the production of inflammatory cytokines inactivated macrophages by reducing the stability and translation of cytokine transcripts. Arthritis Rheum 2003;48:541-50.
- Xiao W, Hodge DR, Wang L, Yang X, Zhang X, Farrar W. Co-operative function between nuclear factors NF-κB and CCAAT/enhancer-binding protein-β (C/EBP-β) regulate the IL-6 promoter in autocrine human prostate cancer cells. Prostate 2004;61:354-70.
- Zerbini LF, Wang Y, Cho JY, Libermann TA. Constitutive activation of nuclear factor κB p50/65 and Fra-1 and JunD is essential for deregulated interleukin 6 expression in prostate cancer. Cancer Res 2003;63:2206-15.
- Keller ET, Chang C, Ershler WB. Inhibiton of NF-κB activity through maintenance of IκBα levels contributes to dihydrotestosterone-mediated repression of the interleukin-6 promoter. J Biol Chem 1996;271:26267-75.