Tumor necrosis factor and cancer, buddies or foes?1
Introduction
Tumor necrosis factor (TNF, also referred to as TNFα) was identified in the late1970s as a cytokine produced by immune cells having a capacity to suppress tumor cell proliferation and induce tumor regression[1,2]. TNF is a protein consisting of 157 amino acids and is synthesized as a membrane-bound protein (pro-TNF) that is released by TNF-converting enzyme (TACE)-mediated cleavage. Since the TNF gene was cloned in 1984, extensive research has revealed a variety of roles for TNF under physiological conditions such as in body development and immunity, and in pathological responses such as inflammation, tumor growth, transplant rejection, rheumatoid arthritis, and septic shock[3]. On the cellular level TNF exerts its effects through its receptors to activate distinct signaling pathways that regulate cell survival, proliferation, or death. Accordingly, complicated roles for TNF in cancer have emerged. On one hand, its anticancer property is mainly through inducing cancer cell death, a process that could be used for cancer therapy. On the other hand, TNF stimulates proliferation, survival, migration, and angiogenesis in most cancer cells that are resistant to TNF-induced cytotoxicity, resulting in tumor promotion. Thus, TNF is a double-edged sword that could be either pro- or anti-tumorigenic. In this review, we focus on the roles and mechanism of TNF in cancer biology with specific emphasis on carcinogenesis and cancer therapy.
TNF-induced signaling pathways
There are 2 receptors for TNF; namely, TNF receptor 1 (TNFR-1, p55 receptor) and TNFR-2 (p75 receptor). TNFR-1 is ubiquitously expressed, whereas TNFR-2 is mainly expressed in immune cells[3]. Although both the receptors bind TNF, the main receptor mediating the cellular effects of TNF in most cell types is TNFR-1. TNFR-1 is a death domain (DD)-containing receptor with an extracellular domain (ECD), a transmembrane domain (TMD), and an intracellular domain (ICD). TNFR-1 is an important member of the death receptor family that shares the capability of inducing apoptotic cell death. TNFR-2 does not possess a DD, although it can mediate a cell death signal, which may be indirect through TNFR-1[4].
The pathways mediated by TNFR-1 have been extensively studied. Upon binding by TNF, which is a natural homotrimer, TNFR-1 forms a homotrimer to recruit the TNFR-associated death domain (TRADD) through the homologous binding of the DD of both proteins. TRADD serves as a platform to recruit downstream adaptor proteins to generate signals for distinct signaling pathways. These adaptor proteins include receptor interacting protein (RIP), TNFR-associated factor 2 (TRAF-2), and Fas-associated death domain (FADD) that further recruit key molecules that are responsible for intracellular signaling to activate NF-κB, mitogen-activated protein kinases (MAPK), and cell death, respectively (Figure 1).
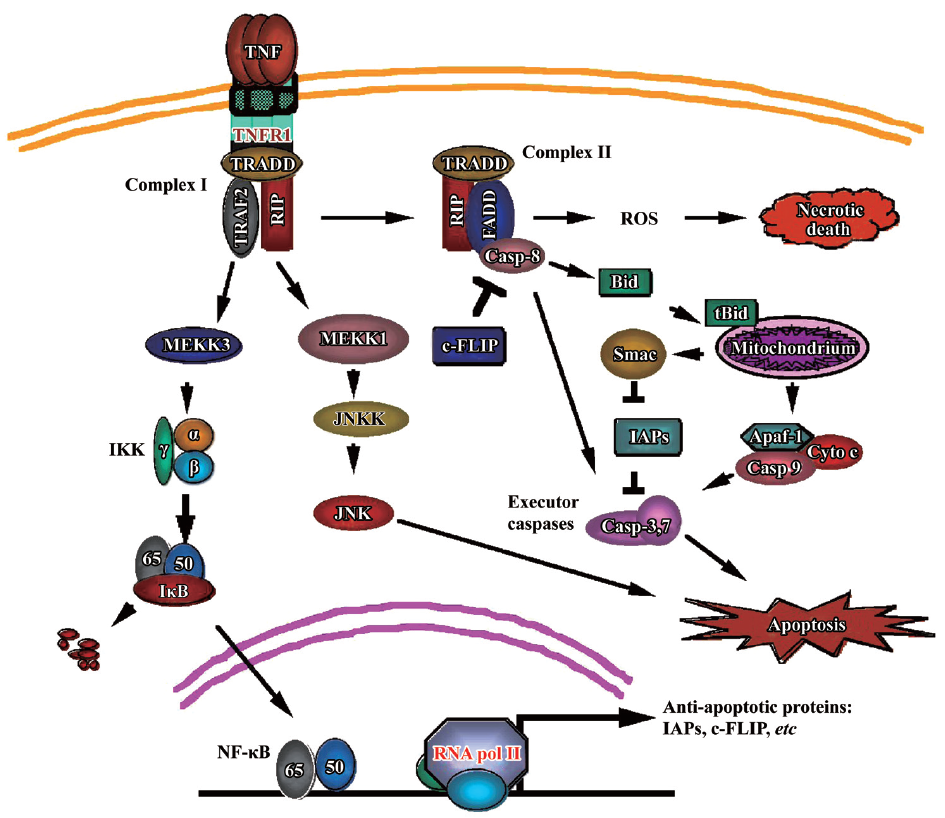
TNF induces NF-κB activation TNF-induced NF-κB activation is initiated by activation of inhibitor of κB (IκB) kinase (IKK). During TNFR-1 signaling IKK is recruited to the TNFR-1 signaling complex (Complex I), which consists of TRADD, TRAF2, and RIP. IKK is activated by a RIP-dependent mechanism that involves MEKK3, TAK1, and TAB2[5,6]. The activated IKK phosphorylates IκB, which retains NF-κB in the cytoplasm, to trigger its rapid polyubiquitination followed by degradation in the 26S proteasome. This process causes the NF-κB nuclear localization signal to be exposed, allowing its nuclear translocation to promote transcription of its target genes. Among NF-κB’s target genes, A20, cIAP-1, cIAP-2, Bcl-xL, XIAP, and IEX-1L are found to have anti-apoptotic properties[7]. Induction of the antioxidant manganese superoxide dismutase (MnSOD) by NF-κB is also implicated in anti-apoptotic and -necrotic cell death[8]. The transcriptional activity of NF-κB is further regulated by phosphorylation and acetylation, which modulate the DNA binding by NF-κB and interaction with transcriptional co-activators and/or co-repressors[4].
TNF induces MAPK activation TNF is able to activate MAPK (extracellular signal-regulated protein kinases [ERK], JNK and p38). In most cell types, JNK is the main MAPK induced by TNF[4,9]. TNFR-1-mediated JNK activation is transduced by TRAF2 and RIP through sequential phosphorylation of the MAP kinase module, MAP3K-MAP2-MAPK[9,10]. MAP3Ks for JNK include members of the MAP/ERK kinase kinase (MEKK) family such as apoptosis signal-regulating kinase 1 (ASK1), mixed lineage kinase (MLK), and transforming growth factor activated kinase 1 (TAK1). Genetic disruption of MEKK1 in mice abrogates TNF-activated JNK. There are 2 MAP2Ks (JNKK1/MKK4/SEK1 and JNKK2/MKK7) that activate JNK. The 2 JNK kinases (JNKKs) phosphorylate JNK at Thr183 and Tyr185, leading to its activation[11]. The role of JNK activation in cell death regulation is controversial, but recent studies suggested that sustained JNK activation, which is suppressed by NF-κB, is pro-apoptotic[12,13]. As is similar to JNK, the activation of ERK and p38 by TNF involves TRAF2 and RIP[9].
TNF induces cytotoxicity As a death receptor TNFR-1 signals cells to die. It is well established that TNF induces apoptosis in a variety of cell types. This pathway is initiated by TNFR1 internally signaling Complex I to form Complex II that consists of TRADD, RIP, FADD, and caspase-8. Caspase-8 is auto-activated to trigger activation of the executor capsases-3, and -7, and the endonucleases, resulting in destruction of cell component proteins, fragmentation of DNA, and, eventually, apoptotic cell death. This death receptor-mediated apoptosis pathway is also called the extrinsic apoptosis pathway. TNF-induced apoptosis also uses the mitochondria-mediated (intrinsic) apoptosis pathway. This is achieved by caspase-8 activating BCL-2 interacting domain (Bid), a BH3-only Bcl2 family member. Cleavage of Bid by caspase-8 generates tBid, which migrates to the mitochondria and causes loss of mitochondrial membrane potential, and release of cytochrome c and second mitochondria-derived activator of caspase (Smac)/ direct IAP binding protein with low pI (DIABLO) from mitochondria to the cytosol. Cytochrome c binds to apoptotic protease activating factor 1 (Apaf-1) and pro-caspase-9 to form apoptosome, resulting in caspase-9-mediated activation of the executor caspases[14]. Smac binds to and inhibits the inhibitor of apoptosis proteins (IAP, including c-IAP1, c-IAP2, X-linked inhibitor of apoptosis protein [XIAP], and survivin), releasing the brake to accelerate apoptosis. In some cancer cells this apoptosis pathway is suppressed partly through the suppression of caspase-8 by cellular FLICE inhibitory protein (c-FLIP), a caspase-8 homolog that competes with caspase-8 for binding to FADD, and suppression of the mitochondrial pathway by the anti-apoptotic Bcl2 family members[15].
Despite the well-known Complex II-mediated apoptosis pathway, an additional TNF-induced apoptosis pathway has recently been discovered. Although the exact mechanism has not yet been determined, it is clearly distinct from the well-known pathway that is mediated by FADD, RIP dispensable, and can be suppressed by c-FLIP. The new pathway is mediated by RIP and FADD, independent of TRADD, and is suppressed by c-IAP[16,17] . Both the pathways use caspase-8 as the initiator caspase to activate the apoptotic execution enzymes. Interestingly, in some cancer cells, this new apoptosis pathway is partially or completely suppressed[16].
In addition to apoptosis, TNF can also induce necrotic cell death. Reactive oxygen species (ROS) play a critical role in mediating necrotic cell death because ROS scavenger BHA can effectively block this pathway[18]. This TNF-induced necrosis requires RIP kinase activity[19,20]. Furthermore, this pathway involves RIP-dependent activation of the NADPH oxidase Nox1[21]. However, because this pathway is also damaged in some cancer cells, these cells are capable of evading all of the TNF-induced cell death pathways, resulting in their malignant proliferation. Understanding the mechanism behind this capability would improve TNF’s anticancer value.
The aforementioned crosstalk among the TNF-induced pathways plays a key role in the biological effects of TNF on cancer. For example, NF-κB suppresses the apoptotic JNK activation and mediates expression of anti-apoptotic and antioxidant genes, blocking cell death and facilitating cancer cell proliferation[4,8,12]. Activation of caspase-8 causes cleavage of the key NF-κB mediator RIP to shut off the cell survival signal while it concurrently promotes the apoptosis pathway[22]. The mitochondria-released Smac suppresses IAP, releasing the apoptosis brake. Therefore, the balance of TNF-induced survival- and death-signaling is pivotal in determining the fate of TNF-responding cells. Modulating this balance could help to prevent cancer development and facilitate using TNF for cancer therapy.
TNF and carcinogenesis
A growing body of epidemiological and clinical data supports the concept that chronic inflammation promotes tumor development and progression. As a major pro-inflammatory cytokine, TNF is able to act as an endogenous tumor promoter to bridge inflammation and carcinogenesis. Indeed, recent reports have shown that TNF is involved in all aspects of carcinogenesis as summarized below: cellular transformation, survival, proliferation, invasion, angiogenesis, and metastasis.
Elevated TNF expression levels in tumor patients Numerous reports have shown that the serum TNF concentration is increased in different cancer patients[23,24]. TNF expression was also expressed at higher levels in various pre-neoplastic and tumor tissues[23–25]. Further, the increased TNF expression level in pre-cancerous and tumor cells was associated with the progression of malignant diseases such as chronic lymphocytic leukemia, Barrett’s adenocarcinoma, prostate cancer, breast cancer, and cervical carcinoma[23,24,26–28]. The serum TNF concentration was markedly decreased during chemotherapy in breast and prostate cancer patients, the extent of which was well-correlated with the extent of therapy responses, suggesting that serum TNF level could be an indicator for chemotherapy response and prognosis[23,26,29]. Serum TNF might also be a risk factor for colorectal neoplasia because it is associated with several known risk factors such as age, smoking, and adiposity, and with a higher prevalence of colorectal adenomas[30] (Table 1). These findings indicate that TNF may be involved in tumor development and could be used as an indicator of cancer risk, therapy response, and prognosis for cancer patients.

Full table
TNF promoter polymorphisms and tumor risk Single nucleotide polymorphisms (SNP) in the promoter region of the TNF gene have been intensively studied for susceptibility to numerous cancers. In recent years, several SNP have been identified as being involved in regulating TNF expression. Among these SNP, one at position –308 in the TNF promoter (–308G/A) was found to be associated with susceptibilities to various types of cancer. Individuals carrying an AA/GA genotype at TNF-308 had higher TNF expression levels and an increased cancer risk, including non-Hodgkin’s lymphoma[31,32], hepatocellular carcinoma[33], gastric cancer[34], invasive cervical cancer[35], ulcerative colitis-associated colorectal cancer[36], and non-small cell lung cancer[37]. The –308AA/GA genotype is also associated with the severity of disease in non-small cell lung cancer[37]. In a case-control study carried out in a large north European population, the –308A allele was associated with the presence of vascular invasion of breast cancer[38]. TNF (–308A) was also related to non-Hodgkin’s lymphoma outcome, as freedom from progression was lower and overall survival were shorter in patients with TNF (–308A)[39]. These results suggest that the –308A allele promotes cancer development and progression. On the contrary, the –238A allele in the TNF-promoter is associated with lower risk of cancers. Individuals with –238AA/GA genotypes have decreased susceptibility to lung cancer, gastric cancer, uterine cervical cancer, colorectal cancer, or renal cell carcinoma[37,40]. The different effect of these 2 SNP on cancer provides genetic evidence for the involvement of TNF in carcinogenesis. Carrying the TNF-857T allele, another SNP associated with decreased TNF expression, also confers decreased risk to maltoma[41] and non-Hodgkin’s lymphoma[42]. However, the TNF-857T allele was also found to be associated with increased risk to B-cell lymphoma[43]. Thus, the SNPs may influence the susceptibility to cancer, which may be associated with altered TNF production or a neighboring gene in tight-linkage disequilibrium. These reports indirectly suggest that TNF has a tumor-promoting role and that TNF promoter SNPs could be a predictor for cancer risk (Table 1).
The role of TNF in tumor promotion and growth The tumor promoting role of TNF has also been demonstrated in various mouse tumor models. There is mounting evidence indicating that pathophysiological concentrations of endogenous TNF promote tumor genesis and growth. In a colitis-associated colon carcinogenesis mouse model, mice lacking TNFR-1 or blocking TNF function with the neutralizing antibody etanercept markedly reduced azoxymethane (AOM) and dextran sulfate sodium (DSS)-induced colonic inflammation and attenuated subsequent tumor formation[44]. A similar observation was made in the 7,12-dimethylbenz (α) anthracene (DMBA)-initiated and 12-O-tetradecanoylphorbol 13-acetate (TPA)-promoted mouse skin tumorigenesis model, in which blocking or suppressing TNF conferred resistance to skin carcinogenesis[45,46]. The critical role of TNF in tumor promotion was further confirmed in TNF knockout (TNF–/–) mice expressing both cyclooxygenase-2 (COX-2) and microsomal prostaglandin E synthase-1 (TNF–/– K19-C2mE mice), which showed significant suppression of hyperplastic tumors with reduced cell proliferation compared with TNF wild-type (+/+) K19-C2mE mice[47]. It was found that protein kinase C (PKC) ε activation is an initial signal in TPA-induced shedding of TNF from epidermal keratinocytes[48]. TNF may not be involved in tumor initiation because the frequency of DNA adducts formation and c-Ha-ras mutation is the same in wild-type and TNF–/– mouse epidermis after DMBA treatment. Instead, TNF may mediate tumor promotion via activating NF-κB[49,50] or a PKCα- and AP-1-dependent pathway[51]. TNF treatment dose-dependently increased NF-κB activity and tumor promotion in mouse epidermal JB6 cells[49]. NF-κB activation is critical for TNF-induced tumor promotion, because in the transformation-resistant JB6 mouse epidermal cells (P-cells) TNF was unable to induce NF-κB activation[50]. TNF-induced NF-κB inhibits carcinogen-induced cytotoxicity, thereby increasing the pool of mutated cells that are susceptible to malignant transformation[52]. Activating the PKCα- and activator protein 1 (AP-1)-dependent pathways mediated the induction of a specific subset of AP-1 responsive genes such as granulocyte-macrophage colony-stimulating factor (GM-CSF), matrix metalloproteinases (MMP)-9, and MMP-3 that are important for tumor development[51]. Nuclear factor of activated T-cells 3 (NFAT3) activation may also contribute to TNF-induced anchorage-independent epidermal Cl41 cell growth, at least partially through inducing COX-2 expression[53]. In addition, TNF may promote tumor development via inducing secretory leukocyte protease inhibitor (SLPI)[54], platelet-type 12-lipoxygenase (p12-LOX)[55], or activation-induced cytidine deaminase (AID)[56]. It is also reported that TNF promoted cancer development through inducing gene mutations and DNA instability by upregulating ROS production in the cells[57,58] (Table 2).

Full table
TNF and tumor angiogenesis, invasion, and metastasis It is becoming clear that TNF is involved in tumor angiogenesis, invasion, and metastasis. TNF upregulated expression of angiogenic factors, including interleukin (IL)-8 and vascular endothelial growth factor in U251 glioma cells[59]. TNF secretion by ovarian cancer cells stimulated a constitutive network consisting of cytokines, chemokines, and angiogenic factors that promoted colonization of the peritoneum and neovascularization for developing tumor deposits[60]. In a lung metastasis mouse model, TNFR-1-mediated signals were able to maintain tumor neovascularization at least partly through inducing hepatocyte growth factor (HGF) expression, which was assumed to support lung metastasis[61]. Both exogenous and macrophage-produced TNF accelerated the epithelial-mesenchymal transition (EMT), which characterized the progression of carcinoma and was linked to the acquisition of an invasive phenotype[62–64]. TNF enhanced the invasiveness of tumor cells through inducing MMP-2, -3, -9, and -12[65–67] or α2β1 integrin[68]. TNF activated focal adhesion kinase (FAK), which was a pivotal mediator for MMP-9 production in cholangiocarcinoma cells[69]. Other mechanisms may also underlie TNF-induced tumor cell invasion, including NF-κB- and JNK-mediated upregulation of macrophage migration-inhibitory factor (MIF) and extracellular MMP inducer in tumor cells[70]. In addition, TNF enhanced a variety of tumor cells to adhere to the mesothelium in vitro and increased tumor migration and metastasis in vivo, partly through NF-κB-dependent induction of the chemokine receptor CXCR4, upregulation of monocyte chemoattractant protein-1 (MCP-1), and IL-8 and intercellular adhesion molecule-1 in cancer cells[71–77]. Upregulation of lectin-like oxidized-low-density lipoprotein (oxLDL) receptor-1 (LOX-1) by TNF in endothelial cell was also shown to promote the adhesion and trans-endothelial migration of MDA-MB-231 breast cancer cells[78], which may be involved in colonizing tumor cells at remote sites during metastasis (Table 2).
A potential anti-oncogenic effect of TNF Although most studies showed that TNF promoted tumorigenesis, TNF exhibited an anti-oncogenic effect in some experimental systems. Despite the reported role of TNF in chronic inflammation, TNF–/– mice were significantly more susceptible to 3′-methylcholanthrene (MCA) -induced skin sarcoma than wild-type mice, suggesting that TNF protects the host against MCA-induced sarcoma formation[79]. A similar effect of TNF was observed in a xenografted glioma mouse model[80,81]. The antitumor role of TNF may involve immune responses that prevent tumor formation, for example, promoting tumor stroma destruction by cytotoxic T lymphocyte (CTL) or tumor infiltrating macrophages[80,82,83] and activating tumor-infiltrating dendritic cells (DC), thereby triggering a potent adaptive immune response leading to tumor rejection[84]. Using gene knock-out mice that were null of TNFR-1, -2, or both, it was found that TNFR-2 expressed in host innate immune cells is sufficient to mediate the antitumor effect of TNF. A large number of macrophages were recruited by the TNFR-2-mediated production of nitric oxide (NO), which efficiently inhibited angiogenesis in the tumor[85].
How the pro- or anti-tumorigenesis roles of TNF are regulated is currently unclear, but could be attributed to differences in organs, cell contexts, and carcinogens. For example, TNF-induced NF-κB activity has contradictory effects in organs with different regenerating rates (ie, anti-tumorigenic in rapid regenerating liver while pro-tumorigenic in slow regenerating colon)[86]. Whether anticancer immunity is involved may also contribute to the discrepancy[80,82,83]. The contradictory roles of TNF in tumorigenesis raise questions regarding the use of anti-inflammatory or anti-TNF agents for cancer prevention. Indeed, data from the Wegener’s Granulomatosis Etanercept Trial demonstrate that combining TNF inhibitor etanercept with cyclophosphamide increased, rather than decreased, the cancer risk beyond that observed with cyclophosphamide alone[87]. Therefore, due to the complexity of the roles of TNF in carcinogenesis, more studies are needed to clarify the mechanism behind the development of different cancers before TNF modulating approaches can be used for cancer prevention. Also, caution and surveillance of cancer risk are necessary when inhibiting TNF inhibition is applied for inflammatory disease therapy.
TNF and cancer therapy
Although TNF has cytotoxic, cytostatic, and immunomodulatory effects on malignant tumors, using TNF as a chemotherapeutic drug has been hampered by its deleterious side effects, including systemic shock and widespread inflammatory responses. In addition, many cancer cells are resistant to TNF-induced cytotoxicity. Therefore, the challenge is to maximize the tumor-selective cytotoxicity and ameliorate the side effects of TNF.
TNF mutants with higher antitumor activity and lower systemic toxicity One attempt to overcome the systematic toxicity is to mutate TNF by molecular engineering. It was reported that a lysine-deficient mutant TNF (mTNF-K90R) had higher affinities to both TNFR-1 and -2, a higher in vitro bioactivity, a considerably longer plasma half-life, and an in vivo antitumor therapeutic window 60-fold greater than that of the wild-type TNF[88]. TNF mutants deficient in their lectin-like activity retained their anti-tumor activities, including necrotic and tumoristatic activities and anti-angiogenic activity, but exhibited reduced systemic toxicity and pro-metastatic capacity[89]. Another TNF mutant in which amino acids Arg, Lys, and Arg substituted for Pro, Ser, and Asp at TNF positions 8, 9, and 10, and Phe substituted for C terminal Leu157, along with deletion of the first 7 N-terminal amino acids, also showed a more effective cytotoxicity than wild-type TNF[90]. Therefore, molecular modification of TNF may pave the way for the use of TNF in tumor therapy, although the mechanism by which the reduction of systematic side effects by the TNF mutations is achieved requires further study.
Sensitizing cancer cells to TNF-induced cytotoxicity With the clarification of the mechanisms underlying the tumor cell’s resistance to TNF, a number of new strategies for sensitizing tumor cells to TNF-induced cell death have been explored. As TNF-activated NF-κB is known to be the major mechanism by which tumor cells escape TNF-induced cytotoxicity, the effect of NF-κB blockage on TNF sensitivity has been studied extensively in a variety of experimental systems. Numerous agents, including naturally occurring and synthetic compounds, were shown to sensitize tumor cells to TNF-induced cell death through inhibiting NF-κB activation. Combining these compounds with TNF led to synergistic cytotoxicity in tumor cells[91–97]. Because Akt contributes to TNF-induced NF-κB activation in lung cancer cells, we investigated and found that concurrently suppressing NF-κB and Akt synergistically triggers TNF-induced cytotoxicity in lung cancer cells[98]. Some compounds enhanced TNF-induced cell death in an NF-κB-independent manner via downregulating the protein level of c-FLIP or increasing expression of both TNF-R1 and cathepsin B[99,100].
In addition, TNF can be used as an adjuvant reagent to promote the anti-cancer effect of chemotherapy agents such as doxorubicin[101], sensitize low epidermal growth factor receptor (EGFR)-expressing carcinomas to anti-EGFR therapy[102], or overcome acquired resistance to EGFR tyrosine kinase inhibitor in non-small-cell lung cancer cells[103]. The combination of TNF and chemotherapeutic agents has been shown to be an effect therapeutic strategy for many tumors by increasing tumor sensitivity to treatment.
Local administration of TNF in an isolated limb or organ setting Due to its systemic toxicity, the clinical use of TNF has been limited to its administration through sophisticated locoregional drug-delivery systems in patients with certain types of organ-confined solid tumors. TNF administration through isolated limb perfusion (ILP) for regionally advanced melanomas and soft tissue sarcomas of the limbs was demonstrated to be safe and efficient. When combined with the alkylating agent melphalan, ILP produces a high objective response rate with acceptable local toxicity and negligible systemic toxicity[104,105]. Several reports showed that the inclusion of TNF in ILP appears to be a very efficacious procedure to obtain local control and achieve limb salvage in patients with metastasized, bulky, limb-threatening tumors[105,106]. The action of TNF-based ILP may be twofold: first, there is an increased endothelium permeability, leading to enhanced chemotherapy drug uptake within the tumor tissue; second, a selective destruction of the tumor vasculature that suppresses the tumor’s blood supply occurs. Downregulation of the vascular endothelial growth factor (VEGF) receptor fetal liver kinase-1 (Flk-1) on tumor endothelium may underlie the mechanism of the tumor vasculature targeting effect of TNF[107,108]. Other TNF-based ILP regimens, including combination with hyperthermia or doxorubicin, also were reported with promising results[109,110]. In addition to ILP, TNF-based isolated hepatic perfusion (IHP) for hepatic metastases and intratumoral administration of TNF into the post-operative tumor cavity for patients with malignant glioma are also shown to be safe and effective therapies[111,112]. However, the effectiveness of including TNF in ILP was challenged by results from a randomized prospective multi-institutional trial that showed that TNF did not significantly enhance short-term (up to 3 month) response rates over melphalan alone in locally advanced extremity melanoma[113]. Nevertheless, although more careful studies are required, locoregional drug-delivery of TNF alone or in combination with other therapeutic agents could be a potential tumor therapy approach.
In addition, tumor-targeted delivery of TNF can substantially maximize the local concentration targeting tumor cells and significantly minimize the doses of TNF, thereby effectively reducing the systemic toxicity.
TNF gene therapy driven by inducible promoters One new strategy is the selective delivery of TNF to tumors using an adenoviral vector, for which TNFerade has been extensively studied. In this replication-incompetent adenoviral vector, expression of human TNF is under the control of the early growth response 1 (Egr-1) promoter, which could be activated by ionizing radiation (IR) and diverse anticancer agents including cisplatin, cyclophosphamide, doxorubicin, 5-fluorouracil, gemcitabine, and paclitaxel, allowing temporal and spatial control of TNF release[114–116]. A potent anticancer activity was observed without significant systemic toxic effects using this vector[114]. The combination of TNFerade and temozolomide or IR significantly improved antiglioma efficacy in 2 glioma xenograft models[117]. The TNFerade vector was activated by resveratrol and can be given to patients who are intolerant of radiation or cytotoxic therapy[118]. TNFerade also reduced metastasis in the syngeneic B16F10 murine melanoma model[119]. Further modification of the adenoviral vector allowed higher specificity in tumor targeting through a combination of modifying the viral capsid and restricting tumor-specific TNF expression. The capsid-modified adenoviral vector ensures high selectivity for targeting the α(v)β(3/5) integrin-positive ovarian cancer cells. Meanwhile, the promoter of the tumor antigen MUC-1 in this vector triggered TNF expression in MUC1-positive tumor cells. When this vector was used in ovarian cancer xenografted models, an effective anti-tumor activity was achieved with no obvious toxicity. Thus, combining capsid modification and transcriptional regulation of TNF expression is a promising strategy for developing TNF-based cancer therapy[120]. An infected cell protein 34.5 (ICP34.5)-deleted oncolytic herpes simplex virus (HSV) vector was found to provide localized TNF delivery and anti-tumor effects[121]. Additionally, the membrane-bound TNF was shown to be ideal for cancer gene therapy because it displays better therapeutic activity and lower side effects[122]. Generation of a TNF mutant that is resistant to cleavage by TACE may be a useful approach to increase locally delivered anticancer therapy value of TNF.
Antibody-directed tumor delivery of TNF Another avenue for improving selective localization of TNF at the tumor site is to generate a recombinant protein that fuses TNF to a monoclonal antibody that specifically targets a tumor antigen. These fusion proteins, immunocytokines, were composed of TNF and an antibody or antibody fragment targeting various tumor antigens such as HER-2/neu, gp240 antigen, EGFR, or fibronectin[123–126]. When systemically given, these immunocytokines were shown to specifically deliver TNF to tumors, which resulted in pronounced anti-tumor activity and lowered side effects. In addition, a different strategy using a bispecific antibody (BsAb) that simultaneously recognizes a cancer antigen and TNF was explored and showed some anticancer effects when combined with radiotherapy[127].
Vascular targeting by TNF coupled to tumor-homing peptides TNF is able to cause selective destruction of tumor-associated blood vessels. This therapeutic property was improved by fusing TNF with peptides, such as CNGRCG or ACDCRGDCFCG, that bind tumor blood vessels. Intramuscular administration of plasmid vectors encoding CNGRCG-TNF (NGR-TNF) or ACDCRGDCFCG-TNF (RGD-TNF) inhibited the growth of tumors implanted at distant sites from the plasmid injection site, indicating that vascular targeting was achieved[128]. The combination of NGR-TNF or RGD-TNF with various chemotherapeutic drugs, including doxorubicin, melphalan, cisplatin, paclitaxel, and gemcitabine, enhanced the anticancer response but did not increase toxicity[129–131]. Neovascular targeted TNF may improve drug delivery to tumors by altering vascular permeability[132]. Local induction of interferon (IFN)-γ may play a crucial role in tumor vascular targeting by NGR-TNF[133]. Therefore, vascular targeting by TNF could be a novel strategy for increasing the therapeutic index of chemotherapeutic drugs.
Shielding or encapsulation of TNF for reducing toxicity Surface-shielded or encapsulated TNF expressing plasmid or TNF protein could be another approach to improve its anticancer value. It was found that systemic administration of the surface-shielded TNF expressing vector using transferrin-polyethylenimine (Tf-PEI) resulted in preferential expression of TNF in tumor cells, induced pronounced hemorrhagic tumor necrosis, and inhibited tumor growth in 3 murine tumor models. Neither a detectable change in TNF serum levels nor systemic TNF-related toxicity was observed[134]. Similarly, encapsulating TNF in pegylated liposomes (TNF-PEGL) dramatically improved the serum circulation time and accumulation of TNF in tumor tissue and increased the anticancer efficacy of radiation or chemotherapy in advanced solid tumors[135,136]. TNF can also be encapsulated in nanoparticles to maximize tumor damage and minimize systemic toxicity[137,138].
Enhancing the antitumor adaptive immune responses of TNF As an important mediator of innate immunity, TNF is capable of activating T cells and DCs to enhance host antitumor adaptive immune response. Administration of adenovirus-encoded TNF together with TNF-gene-engineered DCs elicited tumor-specific cytotoxic T cells and evoked prominent tumor suppression[139,140]. TNF behaved as an immunoadjuvant through inducing major histocompatibility complex (MHC) class I molecules or maintaining the maturation status of DC[141,142]. Similarly, TNF gene-engineered CD8+ cytotoxic T cells had enhanced cytotoxicity and prolonged survival in vivo after adoptive transfer, and exerted a stronger antitumor immunity against the immunosuppressive IL-10-secreting B16 lung tumors in mice[143]. Injecting a TNF-engineered tumor vaccine into tumors strongly augmented the overall antitumor effectiveness through development of systemic antitumor immunity[144,145]. Anchoring the soluble TNF via a biotin-avidin-biotin bridge to the membrane of apoptotic melanoma cells was also found to enhance tumor immunogenicity[146].
Anti-TNF treatment in cancer prevention and therapy Because TNF is implicated in tumor growth under certain circumstances, anti-TNF treatment has been tested in some experimental systems for cancer prevention and therapy. TNF could be blocked with anti-TNF neutralizing monoclonal antibodies, soluble TNF receptors, or TNF autovaccination. Inhibiting TNF using the monoclonal antibody infliximab or recombinant human soluble TNFR-2 exerted strong antitumoral effects in mice with pancreatic tumors[147]. In phase I and II clinical trials, both infliximab and etanercept achieved prolonged disease stabilization in patients with metastatic breast cancer, recurrent ovarian cancer, or immunotherapy-resistant or refractory renal cell carcinoma[148–150]. TNF neutralization also significantly prevented the development of malignant pleural effusion in mice[151]. In addition to infliximab and etanercept, immunizing C57BL/6 mice with TNF autovaccine produced a 100-fold antibody response to TNF and significantly reduced both the number and size of metastases of the B16F10 melanoma cells[152]. However, due to the complexity of the role TNF plays in carcinogenesis, the potential tumor-promoting effect of TNF-modulating strategies should be carefully evaluated. Although TNF blockers did not increase overall tumor risk in patients with rheumatoid arthritis, a recent study showed that TNFR-1-mediated signals in antigen presenting cells and TNFR-2-mediated signals in T cells are critically required for effective tumor immune surveillance[153,154]. Thus, caution should be paid to anti-TNF therapy, as it might dampen tumor surveillance to favor cancer development.
Conclusions and perspectives
With better understanding of the molecular mechanisms of TNF-induced cellular signaling, it is becoming clear that TNF plays a major role in the development of different types of cancer. Thus, TNF could be a molecular target for cancer prevention. The cancer cell killing and anticancer immunity modulation properties of TNF render it a potential cancer therapeutic, which has already achieved promising results when used locally. However, the severe systematic toxicity of TNF substantially diminishes the enthusiasm for its application in a clinical setting, although direct tumor-targeting and modification of TNF partly overcome this hurdle. Recent reports found that induction of TNF at a low concentration by Smac mimetics effectively killed cells derived from different cancer types, suggesting that the anticancer activity of TNF could be achieved at non-toxic concentrations[16,155,156]. Thus, efforts should be made to characterize the TNF-resistance mechanism in order to establish effective means to potentiate TNF killing cancer cells. With such strategies, the TNF doses could be significantly reduced to the non-toxic levels that can be well tolerated by cancer patients. Finally, due to the dual and organ-specific roles of TNF in carcinogenesis, TNF-modulating approaches should be carefully evaluated and monitored in regard to cancer therapy and prevention.
Acknowledgements
We thank Vicki Fisher for editing the manuscript.
References
- Matthews N, Watkins JF. Tumour-necrosis factor from the rabbit. I. Mode of action, specificity and physicochemical properties. Br J Cancer 1978;38:302-9.
- Green S, Dobrjansky A, Chiasson MA. Murine tumor necrosis-inducing factor: purification and effects on myelomonocytic leukemia cells. J Natl Cancer Inst 1982;68:997-1003.
- Aggarwal BB. Signalling pathways of the TNF superfamily: a double-edged sword. Nat Rev Immunol 2003;3:745-56.
- Wajant H, Pfizenmaier K, Scheurich P. Tumor necrosis factor signaling. Cell Death Differ 2003;10:45-65.
- Devin A, Cook A, Lin Y, Rodriguez Y, Kelliher M, Liu Z. The distinct roles of TRAF2 and RIP in IKK activation by TNF-R1: TRAF2 recruits IKK to TNF-R1 while RIP mediates IKK activation. Immunity 2000;12:419-29.
- Yang J, Lin Y, Guo Z, Cheng J, Huang J, Deng L, et al. The essential role of MEKK3 in TNF-induced NF-kappaB activation. Nat Immunol 2001;2:620-4.
- Karin M, Yamamoto Y, Wang QM. The IKK NF-kappa B system: a treasure trove for drug development. Nat Rev Drug Discov 2004;3:17-26.
- Kamata H, Honda S, Maeda S, Chang L, Hirata H, Karin M. Reactive oxygen species promote TNF alpha-induced death and sustained JNK activation by inhibiting MAP kinase phosphatases. Cell 2005;120:649-61.
- Devin A, Lin Y, Liu ZG. The role of the death-domain kinase RIP in tumour-necrosis-factor-induced activation of mitogen-activated protein kinases. EMBO Rep 2003;4:623-7.
- Liu ZG, Hsu H, Goeddel DV, Karin M. Dissection of TNF receptor 1 effector functions: JNK activation is not linked to apoptosis while NF-kappaB activation prevents cell death. Cell 1996;87:565-76.
- Liu J, Lin A. Role of JNK activation in apoptosis: a double-edged sword. Cell Res 2005;15:36-42.
- Lin A, Dibling B. The true face of JNK activation in apoptosis. Aging Cell 2002;1:112-6.
- Ventura JJ, Cogswell P, Flavell RA, Baldwin AS Jr, Davis RJ. JNK potentiates TNF-stimulated necrosis by increasing the production of cytotoxic reactive oxygen species. Genes Dev 2004;18:2905-15.
- Wang X. The expanding role of mitochondria in apoptosis. Genes Dev 2001;15:2922-33.
- Wajant H. Death receptors. Essays Biochem 2003;39:53-71.
- Petersen SL, Wang L, Yalcin-Chin A, Li L, Peyton M, Minna J, et al. Autocrine TNF alpha signaling renders human cancer cells susceptible to Smac-mimetic-induced apoptosis. Cancer Cell 2007;12:445-56.
- Wang L, Du F, Wang X. TNF-alpha induces two distinct caspase-8 activation pathways. Cell 2008;133:693-703.
- Lin Y, Choksi S, Shen HM, Yang QF, Hur GM, Kim YS, et al. Tumor necrosis factor-induced nonapoptotic cell death requires receptor-interacting protein-mediated cellular reactive oxygen species accumulation. J Biol Chem 2004;279:10822-8.
- Holler N, Zaru R, Micheau O, Thome M, Attinger A, Valitutti S, et al. Fas triggers an alternative, caspase-8-independent cell death pathway using the kinase RIP as effector molecule. Nat Immunol 2000;1:489-95.
- Degterev A, Hitomi J, Germscheid M, Ch’en IL, Korkina O, Teng X, et al. Identification of RIP1 kinase as a specific cellular target of necrostatins. Nat Chem Biol 2008;4:313-21.
- Kim YS, Morgan MJ, Choksi S, Liu ZG. TNF-induced activation of the Nox1 NADPH oxidase and its role in the induction of necrotic cell death. Mol Cell 2007;26:675-87.
- Lin Y, Devin A, Rodriguez Y, Liu ZG. Cleavage of the death domain kinase RIP by caspase-8 prompts TNF-induced apoptosis. Genes Dev 1999;13:2514-26.
- Ferrajoli A, Keating MJ, Manshouri T, Giles FJ, Dey A, Estrov Z, et al. The clinical significance of tumor necrosis factor-alpha plasma level in patients having chronic lymphocytic leukemia. Blood 2002;100:1215-9.
- Ahmed MI, Salahy EE, Fayed ST, El-Hefnawy NG, Khalifa A. Human papillomavirus infection among Egyptian females with cervical carcinoma: relationship to spontaneous apoptosis and TNF-alpha. Clin Biochem 2001;34:491-8.
- Szlosarek PW, Grimshaw MJ, Kulbe H, Wilson JL, Wilbanks GD, Burke F, et al. Expression and regulation of tumor necrosis factor alpha in normal and malignant ovarian epithelium. Mol Cancer Ther 2006;5:382-90.
- Michalaki V, Syrigos K, Charles P, Waxman J. Serum levels of IL-6 and TNF-alpha correlate with clinicopathological features and patient survival in patients with prostate cancer. Br J Cancer 2004;90:2312-6.
- Tselepis C, Perry I, Dawson C, Hardy R, Darnton SJ, McConkey C, et al. Tumour necrosis factor-alpha in Barrett’s oesophagus: a potential novel mechanism of action. Oncogene 2002;21:6071-81.
- Garcia-Tunon I, Ricote M, Ruiz A, Fraile B, Paniagua R, Royuela M. Role of tumor necrosis factor-alpha and its receptors in human benign breast lesions and tumors (in situ and infiltrative). Cancer Sci 2006;97:1044-9.
- Berberoglu U, Yildirim E, Celen O. Serum levels of tumor necrosis factor alpha correlate with response to neoadjuvant chemotherapy in locally advanced breast cancer. Int J Biol Markers 2004;19:130-4.
- Kim S, Keku TO, Martin C, Galanko J, Woosley JT, Schroeder JC, et al. Circulating levels of inflammatory cytokines and risk of colorectal adenomas. Cancer Res 2008;68:323-8.
- Bel Hadj Jrad B, Chatti A, Laatiri A, Ahmed SB, Romdhane A, Ajimi S, et al. Tumor necrosis factor promoter gene polymorphism associated with increased susceptibility to non-Hodgkin’s lymphomas. Eur J Haematol 2007;78:117-22.
- Rothman N, Skibola CF, Wang SS, Morgan G, Lan Q, Smith MT, et al. Genetic variation in TNF and IL10 and risk of non-Hodgkin lymphoma: a report from the InterLymph Consortium. Lancet Oncol 2006;7:27-38.
- Ho SY, Wang YJ, Chen HL, Chen CH, Chang CJ, Wang PJ, et al. Increased risk of developing hepatocellular carcinoma associated with carriage of the TNF2 allele of the -308 tumor necrosis factor-alpha promoter gene. Cancer Causes Control 2004;15:657-63.
- Machado JC, Figueiredo C, Canedo P, Pharoah P, Carvalho R, Nabais S, et al. A proinflammatory genetic profile increases the risk for chronic atrophic gastritis and gastric carcinoma. Gastroenterology 2003;125:364-71.
- Duarte I, Santos A, Sousa H, Catarino R, Pinto D, Matos A, et al. G-308A TNF-alpha polymorphism is associated with an increased risk of invasive cervical cancer. Biochem Biophys Res Commun 2005;334:588-92.
- Garrity-Park MM, Loftus EV Jr, Bryant SC, Sandborn WJ, Smyrk TC. Tumor necrosis factor-alpha polymorphisms in ulcerative colitis-associated colorectal cancer. Am J Gastroenterol 2008;103:407-15.
- Shih CM, Lee YL, Chiou HL, Chen W, Chang GC, Chou MC, et al. Association of TNF-alpha polymorphism with susceptibility to and severity of non-small cell lung cancer. Lung Cancer 2006;52:15-20.
- Azmy IA, Balasubramanian SP, Wilson AG, Stephenson TJ, Cox A, Brown NJ, et al. Role of tumour necrosis factor gene polymorphisms (-308 and -238) in breast cancer susceptibility and severity. Breast Cancer Res 2004;6:R395-400.
- Juszczynski P, Kalinka E, Bienvenu J, Woszczek G, Borowiec M, Robak T, et al. Human leukocyte antigens class II and tumor necrosis factor genetic polymorphisms are independent predictors of non-Hodgkin lymphoma outcome. Blood 2002;100:3037-40.
- Jang WH, Yang YI, Yea SS, Lee YJ, Chun JH, Kim HI, et al. The -238 tumor necrosis factor-alpha promoter polymorphism is associated with decreased susceptibility to cancers. Cancer Lett 2001;166:41-6.
- Wu MS, Chen LT, Shun CT, Huang SP, Chiu HM, Wang HP, et al. Promoter polymorphisms of tumor necrosis factor-alpha are associated with risk of gastric mucosa-associated lymphoid tissue lymphoma. Int J Cancer 2004;110:695-700.
- Purdue MP, Lan Q, Kricker A, Grulich AE, Vajdic CM, Turner J, et al. Polymorphisms in immune function genes and risk of non-Hodgkin lymphoma: findings from the New South Wales non-Hodgkin Lymphoma Study. Carcinogenesis 2007;28:704-12.
- Hellmig S, Fischbach W, Goebeler-Kolve ME, Folsch UR, Hampe J, Schreiber S. A functional promotor polymorphism of TNF-alpha is associated with primary gastric B-Cell lymphoma. Am J Gastroenterol 2005;100:2644-9.
- Popivanova BK, Kitamura K, Wu Y, Kondo T, Kagaya T, Kaneko S, et al. Blocking TNF-alpha in mice reduces colorectal carcinogenesis associated with chronic colitis. J Clin Invest 2008;118:560-70.
- Zhaorigetu S, Yanaka N, Sasaki M, Watanabe H, Kato N. Silk protein, sericin, suppresses DMBA-TPA-induced mouse skin tumorigenesis by reducing oxidative stress, inflammatory responses and endogenous tumor promoter TNF-alpha. Oncol Rep 2003;10:537-43.
- Scott KA, Moore RJ, Arnott CH, East N, Thompson RG, Scallon BJ, et al. An anti-tumor necrosis factor-alpha antibody inhibits the development of experimental skin tumors. Mol Cancer Ther 2003;2:445-51.
- Oshima M, Oshima H, Matsunaga A, Taketo MM. Hyperplastic gastric tumors with spasmolytic polypeptide-expressing metaplasia caused by tumor necrosis factor-alpha-dependent inflammation in cyclooxygenase-2/microsomal prostaglandin E synthase-1 transgenic mice. Cancer Res 2005;65:9147-51.
- Wheeler DL, Ness KJ, Oberley TD, Verma AK. Protein kinase Cepsilon is linked to 12-O-tetradecanoylphorbol-13-acetate-induced tumor necrosis factor-alpha ectodomain shedding and the development of metastatic squamous cell carcinoma in protein kinase Cepsilon transgenic mice. Cancer Res 2003;63:6547-55.
- Suzukawa K, Weber TJ, Colburn NH. AP-1, NF-kappa-B, and ERK activation thresholds for promotion of neoplastic transformation in the mouse epidermal JB6 model. Environ Health Perspect 2002;110:865-70.
- Hu J, Nakano H, Sakurai H, Colburn NH. Insufficient p65 phosphorylation at S536 specifically contributes to the lack of NF-kappaB activation and transformation in resistant JB6 cells. Carcinogenesis 2004;25:1991-2003.
- Arnott CH, Scott KA, Moore RJ, Hewer A, Phillips DH, Parker P, et al. Tumour necrosis factor-alpha mediates tumour promotion via a PKC alpha- and AP-1-dependent pathway. Oncogene 2002;21:4728-38.
- Yang H, Bocchetta M, Kroczynska B, Elmishad AG, Chen Y, Liu Z, et al. TNF-alpha inhibits asbestos-induced cytotoxicity via a NF-kappaB-dependent pathway, a possible mechanism for asbestos-induced oncogenesis. Proc Natl Acad Sci USA 2006;103:10397-402.
- Yan Y, Li J, Ouyang W, Ma Q, Hu Y, Zhang D, et al. NFAT3 is specifically required for TNF-alpha-induced cyclooxygenase-2 (COX-2) expression and transformation of Cl41 cells. J Cell Sci 2006;119:2985-94.
- Devoogdt N, Revets H, Kindt A, Liu YQ, De Baetselier P, Ghassabeh GH. The tumor-promoting effect of TNF-alpha involves the induction of secretory leukocyte protease inhibitor. J Immunol 2006;177:8046-52.
- Piao YS, Du YC, Oshima H, Jin JC, Nomura M, Yoshimoto T, et al. Platelet-type 12-lipoxygenase accelerates tumor promotion of mouse epidermal cells through enhancement of cloning efficiency. Carcinogenesis 2008;29:440-7.
- Komori J, Marusawa H, Machimoto T, Endo Y, Kinoshita K, Kou T, et al. Activation-induced cytidine deaminase links bile duct inflammation to human cholangiocarcinoma. Hepatology 2008;47:888-96.
- Yan B, Wang H, Rabbani ZN, Zhao Y, Li W, Yuan Y, et al. Tumor necrosis factor-alpha is a potent endogenous mutagen that promotes cellular transformation. Cancer Res 2006;66:11565-70.
- Babbar N, Casero RA Jr. Tumor necrosis factor-alpha increases reactive oxygen species by inducing spermine oxidase in human lung epithelial cells: a potential mechanism for inflammation-induced carcinogenesis. Cancer Res 2006;66:11125-30.
- Nabors LB, Suswam E, Huang Y, Yang X, Johnson MJ, King PH. Tumor necrosis factor alpha induces angiogenic factor up-regulation in malignant glioma cells: a role for RNA stabilization and HuR. Cancer Res 2003;63:4181-7.
- Kulbe H, Thompson R, Wilson JL, Robinson S, Hagemann T, Fatah R, et al. The inflammatory cytokine tumor necrosis factor-alpha generates an autocrine tumor-promoting network in epithelial ovarian cancer cells. Cancer Res 2007;67:585-92.
- Tomita Y, Yang X, Ishida Y, Nemoto-Sasaki Y, Kondo T, Oda M, et al. Spontaneous regression of lung metastasis in the absence of tumor necrosis factor receptor p55. Int J Cancer 2004;112:927-33.
- Bates RC, DeLeo MJ 3rd, Mercurio AM. The epithelial-mesenchymal transition of colon carcinoma involves expression of IL-8 and CXCR-1-mediated chemotaxis. Exp Cell Res 2004;299:315-24.
- Bates RC, Mercurio AM. Tumor necrosis factor-alpha stimulates the epithelial-to-mesenchymal transition of human colonic organoids. Mol Biol Cell 2003;14:1790-800.
- Chuang MJ, Sun KH, Tang SJ, Deng MW, Wu YH, Sung JS, et al. Tumor-derived tumor necrosis factor-alpha promotes progression and epithelial-mesenchymal transition in renal cell carcinoma cells. Cancer Sci 2008;99:905-1.
- Cheng SM, Xing B, Li JC, Cheung BK, Lau AS. Interferon-gamma regulation of TNFalpha-induced matrix metalloproteinase 3 expression and migration of human glioma T98G cells. Int J Cancer 2007;121:1190-6.
- Esteve PO, Chicoine E, Robledo O, Aoudjit F, Descoteaux A, Potworowski EF, et al. Protein kinase C-zeta regulates transcription of the matrix metalloproteinase-9 gene induced by IL-1 and TNF-alpha in glioma cells via NF-kappa B. J Biol Chem 2002;277:35150-5.
- Hagemann T, Robinson SC, Schulz M, Trumper L, Balkwill FR, Binder C. Enhanced invasiveness of breast cancer cell lines upon co-cultivation with macrophages is due to TNF-alpha dependent up-regulation of matrix metalloproteases. Carcinogenesis 2004;25:1543-9.
- Montesano R, Soulie P, Eble JA, Carrozzino F. Tumour necrosis factor alpha confers an invasive, transformed phenotype on mammary epithelial cells. J Cell Sci 2005;118:3487-500.
- Mon NN, Hasegawa H, Thant AA, Huang P, Tanimura Y, Senga T, et al. A role for focal adhesion kinase signaling in tumor necrosis factor-alpha-dependent matrix metalloproteinase-9 production in a cholangiocarcinoma cell line, CCKS1. Cancer Res 2006;66:6778-84.
- Hagemann T, Wilson J, Kulbe H, Li NF, Leinster DA, Charles K, et al. Macrophages induce invasiveness of epithelial cancer cells via NF-kappa B and JNK. J Immunol 2005;175:1197-205.
- Ziprin P, Ridgway PF, Pfistermuller KL, Peck DH, Darzi AW. ICAM-1 mediated tumor-mesothelial cell adhesion is modulated by IL-6 and TNF-alpha: a potential mechanism by which surgical trauma increases peritoneal metastases. Cell Commun Adhes 2003;10:141-54.
- van Grevenstein WM, Hofland LJ, van Rossen ME, van Koetsveld PM, Jeekel J, van Eijck CH. Inflammatory cytokines stimulate the adhesion of colon carcinoma cells to mesothelial monolayers. Dig Dis Sci 2007;52:2775-83.
- Choo MK, Sakurai H, Koizumi K, Saiki I. TAK1-mediated stress signaling pathways are essential for TNF-alpha-promoted pulmonary metastasis of murine colon cancer cells. Int J Cancer 2006;118:2758-64.
- Kitakata H, Nemoto-Sasaki Y, Takahashi Y, Kondo T, Mai M, Mukaida N. Essential roles of tumor necrosis factor receptor p55 in liver metastasis of intrasplenic administration of colon 26 cells. Cancer Res 2002;62:6682-7.
- Kulbe H, Hagemann T, Szlosarek PW, Balkwill FR, Wilson JL. The inflammatory cytokine tumor necrosis factor-alpha regulates chemokine receptor expression on ovarian cancer cells. Cancer Res 2005;65:10355-62.
- Mochizuki Y, Nakanishi H, Kodera Y, Ito S, Yamamura Y, Kato T, et al. TNF-alpha promotes progression of peritoneal metastasis as demonstrated using a green fluorescence protein (GFP)-tagged human gastric cancer cell line. Clin Exp Metastasis 2004;21:39-47.
- Alkhamesi NA, Ziprin P, Pfistermuller K, Peck DH, Darzi AW. ICAM-1 mediated peritoneal carcinomatosis, a target for therapeutic intervention. Clin Exp Metastasis 2005;22:449-59.
- Liang M, Zhang P, Fu J. Up-regulation of LOX-1 expression by TNF-alpha promotes trans-endothelial migration of MDA-MB-231 breast cancer cells. Cancer Lett 2007;258:31-7.
- Swann JB, Vesely MD, Silva A, Sharkey J, Akira S, Schreiber RD, et al. Demonstration of inflammation-induced cancer and cancer immunoediting during primary tumorigenesis. Proc Natl Acad Sci USA 2008;105:652-6.
- Nakagawa J, Saio M, Tamakawa N, Suwa T, Frey AB, Nonaka K, et al. TNF expressed by tumor-associated macrophages, but not microglia, can eliminate glioma. Int J Oncol 2007;30:803-11.
- Villeneuve J, Tremblay P, Vallieres L. Tumor necrosis factor reduces brain tumor growth by enhancing macrophage recruitment and microcyst formation. Cancer Res 2005;65:3928-36.
- Dace DS, Chen PW, Niederkorn JY. CD8+ T cells circumvent immune privilege in the eye and mediate intraocular tumor rejection by a TNF-alpha-dependent mechanism. J Immunol 2007;178:6115-22.
- Zhang B, Karrison T, Rowley DA, Schreiber H. IFN-gamma- and TNF-dependent bystander eradication of antigen-loss variants in established mouse cancers. J Clin Invest 2008;118:1398-404.
- Larmonier N, Cathelin D, Larmonier C, Nicolas A, Merino D, Janikashvili N, et al. The inhibition of TNF-alpha anti-tumoral properties by blocking antibodies promotes tumor growth in a rat model. Exp Cell Res 2007;313:2345-55.
- Zhao X, Mohaupt M, Jiang J, Liu S, Li B, Qin Z. Tumor necrosis factor receptor 2-mediated tumor suppression is nitric oxide dependent and involves angiostasis. Cancer Res 2007;67:4443-50.
- Karin M, Lawrence T, Nizet V. Innate immunity gone awry: linking microbial infections to chronic inflammation and cancer. Cell 2006;124:823-35.
- Stone JH, Holbrook JT, Marriott MA, Tibbs AK, Sejismundo LP, Min YI, et al. Solid malignancies among patients in the Wegener’s Granulomatosis Etanercept Trial. Arthritis Rheum 2006;54:1608-18.
- Shibata H, Yoshioka Y, Ikemizu S, Kobayashi K, Yamamoto Y, Mukai Y, et al. Functionalization of tumor necrosis factor-alpha using phage display technique and PEGylation improves its antitumor therapeutic window. Clin Cancer Res 2004;10:8293-300.
- Lucas R, Montesano R, Pepper MS, Hafner M, Sablon E, Dunant Y, et al. Lectin-deficient TNF mutants display comparable anti-tumour but reduced pro-metastatic potential as compared to the wild-type molecule. Int J Cancer 2001;91:543-9.
- Yan Z, Zhao N, Wang Z, Li B, Bao C, Shi J, et al. A mutated human tumor necrosis factor-alpha improves the therapeutic index in vitro and in vivo. Cytotherapy 2006;8:415-23.
- Wang X, Ju W, Renouard J, Aden J, Belinsky SA, Lin Y. 17-allylamino-17-demethoxygeldanamycin synergistically potentiates tumor necrosis factor-induced lung cancer cell death by blocking the nuclear factor-kappa B pathway. Cancer Res 2006;66:1089-95.
- Zhang S, Lin ZN, Yang CF, Shi X, Ong CN, Shen HM. Suppressed NF-kappaB and sustained JNK activation contribute to the sensitization effect of parthenolide to TNF-alpha-induced apoptosis in human cancer cells. Carcinogenesis 2004;25:2191-9.
- Fas SC, Baumann S, Zhu JY, Giaisi M, Treiber MK, Mahlknecht U, et al. Wogonin sensitizes resistant malignant cells to TNFalpha- and TRAIL-induced apoptosis. Blood 2006;108:3700-6.
- Ju W, Wang X, Shi H, Chen W, Belinsky SA, Lin Y. A critical role of luteolin-induced reactive oxygen species in blockage of tumor necrosis factor-activated nuclear factor-kappa B pathway and sensitization of apoptosis in lung cancer cells. Mol Pharmacol 2007;71:1381-8.
- Rae C, Langa S, Tucker SJ, MacEwan DJ. Elevated NF-kappaB responses and FLIP levels in leukemic but not normal lymphocytes: reduction by salicylate allows TNF-induced apoptosis. Proc Natl Acad Sci USA 2007;104:12790-5.
- Shukla S, Gupta S. Suppression of constitutive and tumor necrosis factor alpha-induced nuclear factor (NF)-kappa B activation and induction of apoptosis by apigenin in human prostate carcinoma PC-3 cells: correlation with down-regulation of NF-kappaB-responsive genes. Clin Cancer Res 2004;10:3169-78.
- Shishodia S, Sethi G, Konopleva M, Andreeff M, Aggarwal BB. A synthetic triterpenoid, CDDO-Me, inhibits IkappaBalpha kinase and enhances apoptosis induced by TNF and chemotherapeutic agents through down-regulation of expression of nuclear factor kappaB-regulated gene products in human leukemic cells. Clin Cancer Res 2006;12:1828-38.
- Wang X, Chen W, Lin Y. Sensitization of TNF-induced cytotoxicity in lung cancer cells by concurrent suppression of the NF-kappaB and Akt pathways. Biochem Biophys Res Commun 2007;355:807-12.
- Duverger V, Murphy AM, Sheehan D, England K, Cotter TG, Hayes I, et al. The anticancer drug mithramycin A sensitises tumour cells to apoptosis induced by tumour necrosis factor (TNF). Br J Cancer 2004;90:2025-31.
- Mathiasen IS, Hansen CM, Foghsgaard L, Jaattela M. Sensitization to TNF-induced apoptosis by 1,25-dihydroxy vitamin D(3) involves up-regulation of the TNF receptor 1 and cathepsin B. Int J Cancer 2001;93:224-31.
- Cao W, Chi WH, Wang J, Tang JJ, Lu YJ. TNF-alpha promotes Doxorubicin-induced cell apoptosis and anti-cancer effect through downregulation of p21 in p53-deficient tumor cells. Biochem Biophys Res Commun 2005;330:1034-40.
- Hambek M, Solbach C, Schnuerch HG, Roller M, Stegmueller M, Sterner-Kock A, et al. Tumor necrosis factor alpha sensitizes low epidermal growth factor receptor (EGFR)-expressing carcinomas for anti-EGFR therapy. Cancer Res 2001;61:1045-9.
- Ando K, Ohmori T, Inoue F, Kadofuku T, Hosaka T, Ishida H, et al. Enhancement of sensitivity to tumor necrosis factor alpha in non-small cell lung cancer cells with acquired resistance to gefitinib. Clin Cancer Res 2005;11:8872-9.
- Hayes AJ, Neuhaus SJ, Clark MA, Thomas JM. Isolated limb perfusion with melphalan and tumor necrosis factor alpha for advanced melanoma and soft-tissue sarcoma. Ann Surg Oncol 2007;14:230-8.
- Grunhagen DJ, de Wilt JH, ten Hagen TL, Eggermont AM. Technology insight: Utility of TNF-alpha-based isolated limb perfusion to avoid amputation of irresectable tumors of the extremities. Nat Clin Pract Oncol 2006;3:94-103.
- Lans TE, Grunhagen DJ, de Wilt JH, van Geel AN, Eggermont AM. Isolated limb perfusions with tumor necrosis factor and melphalan for locally recurrent soft tissue sarcoma in previously irradiated limbs. Ann Surg Oncol 2005;12:406-11.
- Farma JM, Puhlmann M, Soriano PA, Cox D, Paciotti GF, Tamarkin L, et al. Direct evidence for rapid and selective induction of tumor neovascular permeability by tumor necrosis factor and a novel derivative, colloidal gold bound tumor necrosis factor. Int J Cancer 2007;120:2474-80.
- Menon C, Iyer M, Prabakaran I, Canter RJ, Lehr SC, Fraker DL. TNF-alpha downregulates vascular endothelial Flk-1 expression in human melanoma xenograft model. Am J Physiol Heart Circ Physiol 2003;284:H317-29.
- Rossi CR, Mocellin S, Pilati P, Foletto M, Campana L, Quintieri L, et al. Hyperthermic isolated perfusion with low-dose tumor necrosis factor alpha and doxorubicin for the treatment of limb-threatening soft tissue sarcomas. Ann Surg Oncol 2005;12:398-405.
- Di Filippo F, Garinei R, Anza M, Cavaliere F, Giannarelli D, Cagol PP, et al. Doxorubicin in isolation limb perfusion in the treatment of advanced limb soft tissue sarcoma. J Exp Clin Cancer Res 2003;22 Suppl:81-7.
- van Etten B, de Vries MR. Degree of tumour vascularity correlates with drug accumulation and tumour response upon TNF-alpha-based isolated hepatic perfusion. Br J Cancer 2003;88:314-9.
- Oshiro S, Tsugu H, Komatsu F, Ohnishi H, Ueno Y, Sakamoto S, et al. Evaluation of intratumoral administration of tumor necrosis factor-alpha in patients with malignant glioma. Anticancer Res 2006;26:4027-32.
- Cornett WR, McCall LM, Petersen RP, Ross MI, Briele HA, Noyes RD, et al. Randomized multicenter trial of hyperthermic isolated limb perfusion with melphalan alone compared with melphalan plus tumor necrosis factor: American College of Surgeons Oncology Group Trial Z0020. J Clin Oncol 2006;24:4196-201.
- McLoughlin JM, McCarty TM, Cunningham C, Clark V, Senzer N, Nemunaitis J, et al. TNFerade, an adenovector carrying the transgene for human tumor necrosis factor alpha, for patients with advanced solid tumors: surgical experience and long-term follow-up. Ann Surg Oncol 2005;12:825-30.
- Lopez CA, Kimchi ET, Mauceri HJ, Park JO, Mehta N, Murphy KT, et al. Chemoinducible gene therapy: a strategy to enhance doxorubicin antitumor activity. Mol Cancer Ther 2004;3:1167-75.
- Gupta VK, Park JO, Jaskowiak NT, Mauceri HJ, Seetharam S, Weichselbaum RR, et al. Combined gene therapy and ionizing radiation is a novel approach to treat human esophageal adenocarcinoma. Ann Surg Oncol 2002;9:500-4.
- Yamini B, Yu X, Pytel P, Galanopoulos N, Rawlani V, Veerapong J, et al. Adenovirally delivered tumor necrosis factor-alpha improves the antiglioma efficacy of concomitant radiation and temozolomide therapy. Clin Cancer Res 2007;13:6217-23.
- Bickenbach KA, Veerapong J, Shao MY, Mauceri HJ, Posner MC, Kron SJ, et al. Resveratrol is an effective inducer of CArG-driven TNF-alpha gene therapy. Cancer Gene Ther 2008;15:133-9.
- MacGill RS, Davis TA, Macko J, Mauceri HJ, Weichselbaum RR, King CR. Local gene delivery of tumor necrosis factor alpha can impact primary tumor growth and metastases through a host-mediated response. Clin Exp Metastasis 2007;24:521-31.
- Murugesan SR, Akiyama M, Einfeld DA, Wickham TJ, King CR. Experimental treatment of ovarian cancers by adenovirus vectors combining receptor targeting and selective expression of tumor necrosis factor. Int J Oncol 2007;31:813-22.
- Han ZQ, Assenberg M, Liu BL, Wang YB, Simpson G, Thomas S, et al. Development of a second-generation oncolytic Herpes simplex virus expressing TNFalpha for cancer therapy. J Gene Med 2007;9:99-106.
- Li Q, Li L, Shi W, Jiang X, Xu Y, Gong F, et al. Mechanism of action differences in the antitumor effects of transmembrane and secretory tumor necrosis factor-alpha in vitro and in vivo. Cancer Immunol Immunother 2006;55:1470-9.
- Lyu MA, Rosenblum MG. The immunocytokine scFv23/TNF sensitizes HER-2/neu-overexpressing SKBR-3 cells to tumor necrosis factor (TNF) via up-regulation of TNF receptor-1. Mol Cancer Ther 2005;4:1205-13.
- Liu Y, Zhang W, Cheung LH, Niu T, Wu Q, Li C, et al. The antimelanoma immunocytokine scFvMEL/TNF shows reduced toxicity and potent antitumor activity against human tumor xenografts. Neoplasia 2006;8:384-93.
- Christ O, Seiter S, Matzku S, Burger C, Zoller M. Efficacy of local versus systemic application of antibody-cytokine fusion proteins in tumor therapy. Clin Cancer Res 2001;7:985-98.
- Halin C, Gafner V, Villani ME, Borsi L, Berndt A, Kosmehl H, et al. Synergistic therapeutic effects of a tumor targeting antibody fragment, fused to interleukin 12 and to tumor necrosis factor alpha. Cancer Res 2003;63:3202-10.
- Larbouret C, Robert B, Linard C, Teulon I, Gourgou S, Bibeau F, et al. Radiocurability by targeting tumor necrosis factor-alpha using a bispecific antibody in carcinoembryonic antigen transgenic mice. Int J Radiat Oncol Biol Phys 2007;69:1231-7.
- Zarovni N, Monaco L, Corti A. Inhibition of tumor growth by intramuscular injection of cDNA encoding tumor necrosis factor alpha coupled to NGR and RGD tumor-homing peptides. Hum Gene Ther 2004;15:373-82.
- Sacchi A, Gasparri A, Gallo-Stampino C, Toma S, Curnis F, Corti A. Synergistic antitumor activity of cisplatin, paclitaxel, and gemcitabine with tumor vasculature-targeted tumor necrosis factor-alpha. Clin Cancer Res 2006;12:175-82.
- Curnis F, Gasparri A, Sacchi A, Longhi R, Corti A. Coupling tumor necrosis factor-alpha with alphaV integrin ligands improves its antineoplastic activity. Cancer Res 2004;64:565-71.
- Curnis F, Sacchi A, Corti A. Improving chemotherapeutic drug penetration in tumors by vascular targeting and barrier alteration. J Clin Invest 2002;110:475-82.
- van Laarhoven HW, Gambarota G, Heerschap A, Lok J, Verhagen I, Corti A, et al. Effects of the tumor vasculature targeting agent NGR-TNF on the tumor microenvironment in murine lymphomas. Invest New Drugs 2006;24:27-36.
- Sacchi A, Gasparri A, Curnis F, Bellone M, Corti A. Crucial role for interferon gamma in the synergism between tumor vasculature-targeted tumor necrosis factor alpha (NGR-TNF) and doxorubicin. Cancer Res 2004;64:7150-5.
- Kircheis R, Ostermann E, Wolschek MF, Lichtenberger C, Magin-Lachmann C, Wightman L, et al. Tumor-targeted gene delivery of tumor necrosis factor-alpha induces tumor necrosis and tumor regression without systemic toxicity. Cancer Gene Ther 2002;9:673-80.
- ten Hagen TL, Seynhaeve AL, van Tiel ST, Ruiter DJ, Eggermont AM. Pegylated liposomal tumor necrosis factor-alpha results in reduced toxicity and synergistic antitumor activity after systemic administration in combination with liposomal doxorubicin (Doxil) in soft tissue sarcoma-bearing rats. Int J Cancer 2002;97:115-20.
- Kim DW, Andres ML, Miller GM, Cao JD, Green LM, Seynhaeve AL, et al. Immunohistological analysis of immune cell infiltration of a human colon tumor xenograft after treatment with Stealth liposome-encapsulated tumor necrosis factor-alpha and radiation. Int J Oncol 2002;21:973-9.
- Goel R, Swanlund D, Coad J, Paciotti GF, Bischof JC. TNF-alpha-based accentuation in cryoinjury-dose, delivery, and response. Mol Cancer Ther 2007;6:2039-47.
- Visaria RK, Griffin RJ, Williams BW, Ebbini ES, Paciotti GF, Song CW, et al. Enhancement of tumor thermal therapy using gold nanoparticle-assisted tumor necrosis factor-alpha delivery. Mol Cancer Ther 2006;5:1014-20.
- Kianmanesh A, Hackett NR, Lee JM, Kikuchi T, Korst RJ, Crystal RG. Intratumoral administration of low doses of an adenovirus vector encoding tumor necrosis factor alpha together with naive dendritic cells elicits significant suppression of tumor growth without toxicity. Hum Gene Ther 2001;12:2035-49.
- Liu Y, Saxena A, Zheng C, Carlsen S, Xiang J. Combined alpha tumor necrosis factor gene therapy and engineered dendritic cell vaccine in combating well-established tumors. J Gene Med 2004;6:857-68.
- Lu Y, Yamauchi N, Koshita Y, Fujiwara H, Sato Y, Fujii S, et al. Administration of subtumor regression dosage of TNF-alpha to mice with pre-existing parental tumors augments the vaccination effect of TNF gene-modified tumor through the induction of MHC class I molecule. Gene Ther 2001;8:499-507.
- Chen S, Fribley A, Wang CY. Potentiation of tumor necrosis factor-mediated apoptosis of oral squamous cell carcinoma cells by adenovirus-mediated gene transfer of NF-kappaB inhibitor. J Dent Res 2002;81:98-102.
- Ye Z, Shi M, Chan T, Sas S, Xu S, Xiang J. Engineered CD8+ cytotoxic T cells with fiber-modified adenovirus-mediated TNF-alpha gene transfection counteract immunosuppressive interleukin-10-secreting lung metastasis and solid tumors. Cancer Gene Ther 2007;14:661-75.
- Lasek W, Mackiewicz A, Czajka A, Switaj T, Golb J, Wiznerowicz M, et al. Antitumor effects of the combination therapy with TNF-alpha gene-modified tumor cells and interleukin 12 in a melanoma model in mice. Cancer Gene Ther 2000;7:1581-90.
- Nagy T, Glavinas H, Szincsak N, Hunyadi J, Janossy T, Duda E, et al. Tumor cells expressing membrane-bound tumor necrosis factor activate macrophages and have a compromised growth in immunosuppressed and immunodeficient mice. Cancer Lett 2003;196:49-56.
- Zimmermann VS, Bondanza A, Monno A, Rovere-Querini P, Corti A, Manfredi AA. TNF-alpha coupled to membrane of apoptotic cells favors the cross-priming to melanoma antigens. J Immunol 2004;172:2643-50.
- Egberts JH, Cloosters V, Noack A, Schniewind B, Thon L, Klose S, et al. Anti-tumor necrosis factor therapy inhibits pancreatic tumor growth and metastasis. Cancer Res 2008;68:1443-50.
- Madhusudan S, Foster M, Muthuramalingam SR, Braybrooke JP, Wilner S, Kaur K, et al. A phase II study of etanercept (Enbrel), a tumor necrosis factor alpha inhibitor in patients with metastatic breast cancer. Clin Cancer Res 2004;10:6528-34.
- Madhusudan S, Muthuramalingam SR, Braybrooke JP, Wilner S, Kaur K, Han C, et al. Study of etanercept, a tumor necrosis factor-alpha inhibitor, in recurrent ovarian cancer. J Clin Oncol 2005;23:5950-9.
- Harrison ML, Obermueller E, Maisey NR, Hoare S, Edmonds K, Li NF, et al. Tumor necrosis factor alpha as a new target for renal cell carcinoma: two sequential phase II trials of infliximab at standard and high dose. J Clin Oncol 2007;25:4542-9.
- Stathopoulos GT, Kollintza A, Moschos C, Psallidas I, Sherrill TP, Pitsinos EN, et al. Tumor necrosis factor-alpha promotes malignant pleural effusion. Cancer Res 2007;67:9825-34.
- Waterston AM, Salway F, Andreakos E, Butler DM, Feldmann M, Coombes RC. TNF autovaccination induces self anti-TNF antibodies and inhibits metastasis in a murine melanoma model. Br J Cancer 2004;90:1279-84.
- Geborek P, Bladstrom A, Turesson C, Gulfe A, Petersson IF, Saxne T, et al. Tumour necrosis factor blockers do not increase overall tumour risk in patients with rheumatoid arthritis, but may be associated with an increased risk of lymphomas. Ann Rheum Dis 2005;64:699-703.
- Calzascia T, Pellegrini M, Hall H, Sabbagh L, Ono N, Elford AR, et al. TNF-alpha is critical for antitumor but not antiviral T cell immunity in mice. J Clin Invest 2007;117:3833-45.
- Vince JE, Wong WW, Khan N, Feltham R, Chau D, Ahmed AU, et al. IAP antagonists target cIAP1 to induce TNFalpha-dependent apoptosis. Cell 2007;131:682-93.
- Varfolomeev E, Blankenship JW, Wayson SM, Fedorova AV, Kayagaki N, Garg P, et al. IAP antagonists induce autoubiquitination of c-IAPs, NF-kappaB activation, and TNF alpha-dependent apoptosis. Cell 2007;131:669-81.