Curcumin inhibits WT1 gene expression in human leukemic K562 cells1
Introduction
Leukemias comprise a group of clonal diseases characterized by an accumulation of abnormal blood cells, which are thought to derive from a single cell in the marrow that has undergone a genetic alteration. Leukemia is the most common childhood cancer. Malignant transformation occurs as a result of the accumulation of genetic mutations in cellular genes. Some cases of mutations in oncogenes have provided useful molecular markers for monitoring the course of disease during treatment. The abl translocation in chronic myelogenous leukemia is a good example. The detection of overexpression in specific oncogenes or tumor suppressor genes provides information that is useful in the diagnosis of leukemia and prognosis of the disease. The overexpression of Wilms’ tumor (WT1) protein in leukemia is a promising biomarker. WT1 is expressed in stem cells of the bone marrow, but not in normal mature blood cells[1,2]. Many previous studies have demonstrated that the WT1 gene is highly expressed in leukemic blast cells of myeloid and lymphoid origin, and thus WT1 mRNA provides a novel tumor marker for the detection of minimal residual disease of leukemias and for monitoring disease progression of myelodysplastic syndromes[3–9]. It has been demonstrated that the WT1 gene is expressed in leukemic cell lines K562 and HL60, and that differentiation of these cells in culture is accompanied by downregulation of WT1 protein levels[10,11]. K562 cells, which are derived from chronic myelocytic leukemia blastic crisis and express high levels of WT1, can be treated with nocodazole (40 ng/mL) to synchronously arrest the cell cycle[12].
The WT1 gene is defined as a tumor suppressor gene in childhood renal tumors. However, the wild-type WT1 gene is highly expressed in leukemic blast cells. The WT1 gene is expressed at high levels in various types of leukemias [acute myeloblastic leukemia (AML), acute lymphoblastic leukemia (ALL) and chronic myelocytic leukemia (CML)]. Miwa et al[13] and Miyaki et al[14] examined WT1 gene expression in leukemias using Northern blot analysis and detected WT1 gene expression in some cases of AML, ALL, and CML in the accelerated phase or blast crisis. Inoue et al provided a new insight into the significance of WT1 gene expression in leukemias by quantifying the expression levels of the WT1 gene by quantitative reverse transcriptase-polymerase chain reaction (RT-PCR)[15]. In all leukemia samples examined, including AML, ALL, and CML, significant levels of WT1 gene expression were found, and the average levels were approximately 1000 and 100 000 times greater than those in normal bone marrow or peripheral blood cells, respectively[16]. Moreover, Bergmann et al reported a correlation between WT1 mRNA levels and prognosis[17]. Taken together, all these findings demonstrate that WT1 mRNA is a novel tumor marker in leukemic blast cells of almost all leukemias and that its expression level is a new prognostic factor for acute leukemia.
Curcuminoids are natural phenolic coloring compounds found in rhizomes of Curcuma longa Linn, a member of Zingiberaceae (ginger) family, and commonly known as turmeric. Curcuminoid content in turmeric varies from 1% to 5% of fresh turmeric rhizome, and has been identified as the major yellow pigment in turmeric. It has been widely used as a spice, to color cheese and butter, as a cosmetic, and in some medicinal preparations[18,19]. Curcuminoids include curcumin (curcumin I), demethoxycurcumin (curcumin II) and bisdemethoxycurcumin (curcumin III). All commercial curcuminoids sold as “curcumin” (eg ICN, GNC, and Sigma-Aldrich), are mixtures of the 3 curcuminoids. Curcumin has a wide range of biological and pharmacological activities, including antioxidant properties[20–22], anti-inflammatory properties[19], anti-mutagenic activity in vitro[23], anti-carcinogenic effects[24–26], hypocholesterolemic effects in rats[27], hypoglycemic effects in humans[28], and multidrug resistance (MDR) modulation effects[29]. The safety of curcumin has been studied in various animal models[30], and it is clear that turmeric is not toxic even at high doses in laboratory animals. A single feeding of a 30% turmeric diet to rats did not produce any toxic effects. In a 24-h acute toxicity study, mice were fed doses of 0.5, 1.0, and 3.0 g/kg of turmeric extract. There was no increase in mortality compared with controls in either study. A 90-d treatment with turmeric extract resulted in no significant weight gain[31].
Due to its wide range of biological and pharmacological effects and lack of toxicity in animal models, curcumin was selected for study in leukemia. In this paper we aimed to examine the modulating effect of curcumin on WT1 gene expression in the K562 human leukemic cell line.
Materials and methods
Reagents Commercial grade curcumin (77% curcumin, 17% demethoxycurcumin and 3% bisdemethoxycurcumin), 3-(4,5-dimethyl-2 thiazoyl)-2,5-diphenyl-tetrazolium bromide (MTT) dye, and dimethylsulfoxide (Me2SO) were purchased from Sigma-Aldrich (St Louis, MO, USA). RPMI 1640, SuperScript III One-Step RT-PCR System with Platinum Taq DNA polymerase reagent, Trizol reagent, penicillin-strepto-mycin, L-glutamine, and primers were purchased from Invitro-gen Life Technology (Carlsbad, CA, USA). Primary mouse polyclonal anti-WT1 clone C-19 was purchased from Santa Cruz Biotechnology (Santa Cruz, CA, USA). Horseradish peroxidase (HRP)-conjugated goat anti-mouse IgG was purchased from Promega (Madison, WI, USA). A SuperSignal detection kit was purchased from Pierce (Rockford, IL, USA).
Cells and cell cultures The erythroid leukemic cell line (K562) was a generous gift from Dr Chaisuree SUPAWILAI (Research Institute for Health Sciences, Chiang Mai, Thai-land). This cell line was cultured in RPMI 1640 medium containing 10% fetal calf serum, 1 mmol/L L-glutamine, 50 U/mL penicillin, and 50 µg/mL streptomycin. Cells were maintained in a humidified incubator with an atmosphere of 95% air and 5% CO2 at 37 °C. When the cells reached 80% confluency, they were harvested and plated for consequent passages or for curcumin treatment.
The effects of curcumin on cell growth were observed by examining the morphology of cultures with an inverted phase contrast microscope. The MTT test was used throughout all experiments to check cell viability.
MTT assay Cell survival was determined by using the MTT assay as described elsewhere[32]. The MTT assay was performed by plating cells in 96-well plates (3.0×105 cells/well) in 100 µL medium, and incubating them at 37 °C for 1 d before curcumin treatment. After 1 d, curcumin stocks prepared in Me2SO were added to the culture medium (100 µL) at various concentrations and incubated in a humidified tissue-culture chamber (37 °C, 5% CO2) for another day. The Me2SO concentration was kept at 0.4%. The cell survival in each well was determined by the MTT assay and compared with that of untreated cells. Briefly, after removal of 100 µL medium, MTT stock dye solution was added (15 µL/100 µL medium) to each well, and the plate was incubated at 37 in 5% CO2 atmosphere. After 4 h, Me2SO (100 mL) was added to each well and mixed thoroughly to dissolve the dye crystals. Absorbance at a wavelength of 570 nm was measured with an enzyme-linked immunosorbent assay (ELISA) plate reader with a reference wavelength of 630 nm. Fractional absorbance was calculated by using the following formula:
% Cell survival = Mean absorbance in test wells × 100
Mean absorbance in control wells
Western blot analysis Cell nuclear extracts were prepared as described previously[33]. The cell nuclear proteins (100 µg/lane) were separated by 12% sodium dodecylsulfate-polyacrylamide gel electrophoresis and immunoblotted overnight onto nitrocellulose filters. The filters were incubated sequentially with primary mouse polyclonal anti-WT1 clone C-19 at a 1:1000 dilution, followed by a treatment with HRP-conjugated goat anti-mouse IgG at a 1:15 000 dilution. Proteins were visualized by using the SuperSignal protein detection kit and quantitated by using a scan densitometer.
RNA extraction and quantitative RT-PCR RNAs of K562 cells were isolated by using the Trizol reagent according to the manufacturer’s instructions. RNaseOUT was added to the RNA extraction products for RNA protection (40 units per 20 µL of reaction mixture). The amount of RNA was determined by optical density (OD) measurement at a wavelength of 260 nm (one OD unit = 40 µg/mL). RT-PCR was performed using the SuperScript III One-step RT-PCR System with Platinum Taq DNA polymerase reagent. For WT1, the sense primer was 5'-GGCATCTGAGACCAGTGAGAA-3', and the antisense primer was 5'-GAGAGTCAGACTTG-AAAGCAGT-3', corresponding to residues 780–800 and residues 1232–1253, respectively, of the published cDNA sequence[34]. cDNA was synthesized from 1 µg of total RNA at 60 ºC for 30 min and denatured at 94 ºC for 2 min. PCR amplification was performed for 30 cycles of sequential denaturation (94 ºC, 1 min), annealing (60 ºC, 1 min), and extension (72 ºC, 1 min), which gave a 474 bp product. β-Actin gene expression, used as an internal control for RNA loading, was carried out by using the sense primer 5'-CAGAGCAA-GAGAGGCATCCT-3' and the antisense primer 5'-TTGAA-GGTCTCAAAC ATGAT-3' corresponding to residues 216–235 and residues 405–424, respectively. The β-actin cDNA was synthesized from 1 µg of total RNA at 55 ºC for 30 msin and denatured at 94 ºC for 2 min. PCR amplification was performed for 30 cycles of sequential denaturation (94 ºC, 1 min), annealing (55 ºC, 1 min), and extension (72 ºC, 1 min), which yielded a 201 bp product. For a negative control, water was amplified using a total of 30 cycles to detect any possible contamination. A total of 15 µL of each PCR product was electrophoresed on a 1% agarose gel, visualized with ethidium bromide staining (2 mg/mL), and quantitated using scan densitometry (Bio-Rad, Richmond, CA, USA).
Statistical analysis All data are expressed as mean±SD from triplicate samples of 3 independent experiments. Statistical differences between the means were analyzed by one-way ANOVA. P<0.05 was considered statistically significant.
Results
Cytotoxic effects of curcumin on the K562 leukemic cells Curcumin produced a cytotoxic effect on K562 cells with an inhibitory concentration at 50% (IC50) of approximately 20 µg/mL (54.3 µmol/L) as shown in Figure 1.
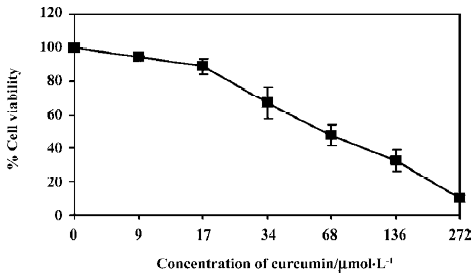
WT1 protein and mRNA expression The K562 cells were treated with 5, 10, or 15 µmol/L curcumin for 2 d before cell harvesting and the nuclear proteins were analyzed by Western blotting. The levels of WT1 protein in the K562 cells were decreased by 10%, 29%, and 63% in response to treatment with 5, 10, and 15 µmol/L curcumin, respectively, compared with the vehicle control (Figure 2).
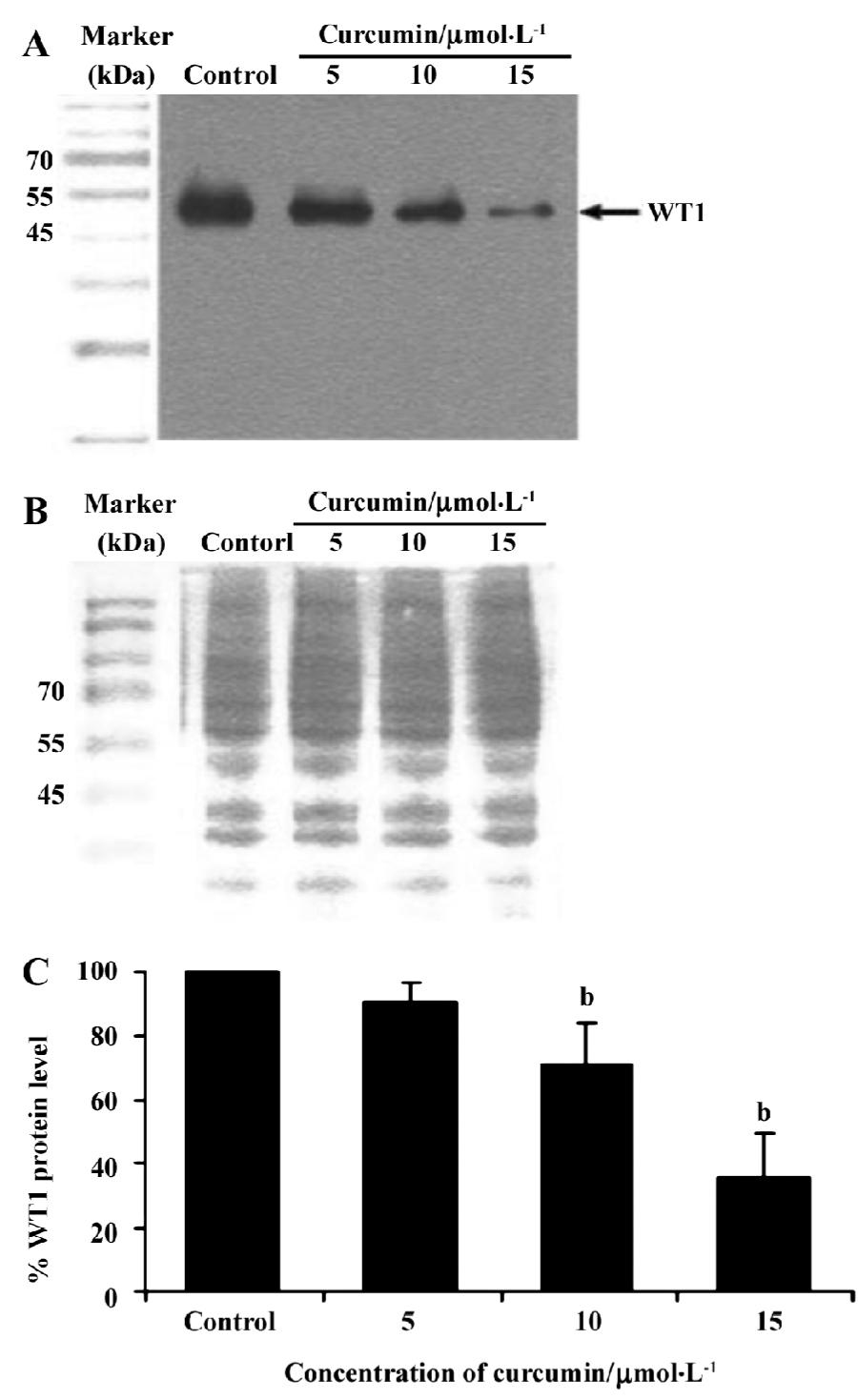
To verify if curcumin could modulate spontaneous WT1 expression (mRNA) occurring in vitro, the same as WT1 protein expression, K562 cells were treated with curcumin (5, 10, or 15 µmol/L) for 2 d and expression of WT1 mRNA was examined by RT-PCR. Values for the expression of WT1 mRNA (after normalization to β-actin expression) in K562 cells were shown to decrease by 17, 37, and 46%, respectively (Figure 3).
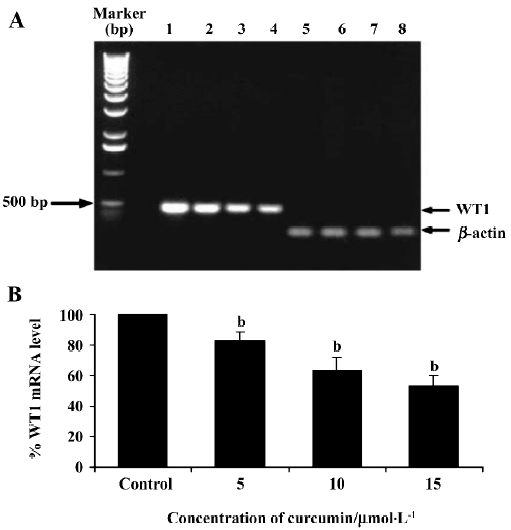
This result clearly shows that curcumin significantly decreased WT1 expression both at the mRNA and protein levels. Therefore, subsequent experiments were designed to observe the modulating effects of curcumin on WT 1 expression after various incubation times. WT1 protein level was found to be decreased by 29%, 51%, and 99%, respectively, in response to 1, 2 and 3 d of treatment (Figure 4). Expression of WT1 mRNA (after normalization to β-actin expression) in K562 cells after treatment with curcumin for 1, 2, and 3 d was decreased by 14%, 38%, and 63%, respectively (Figure 5).
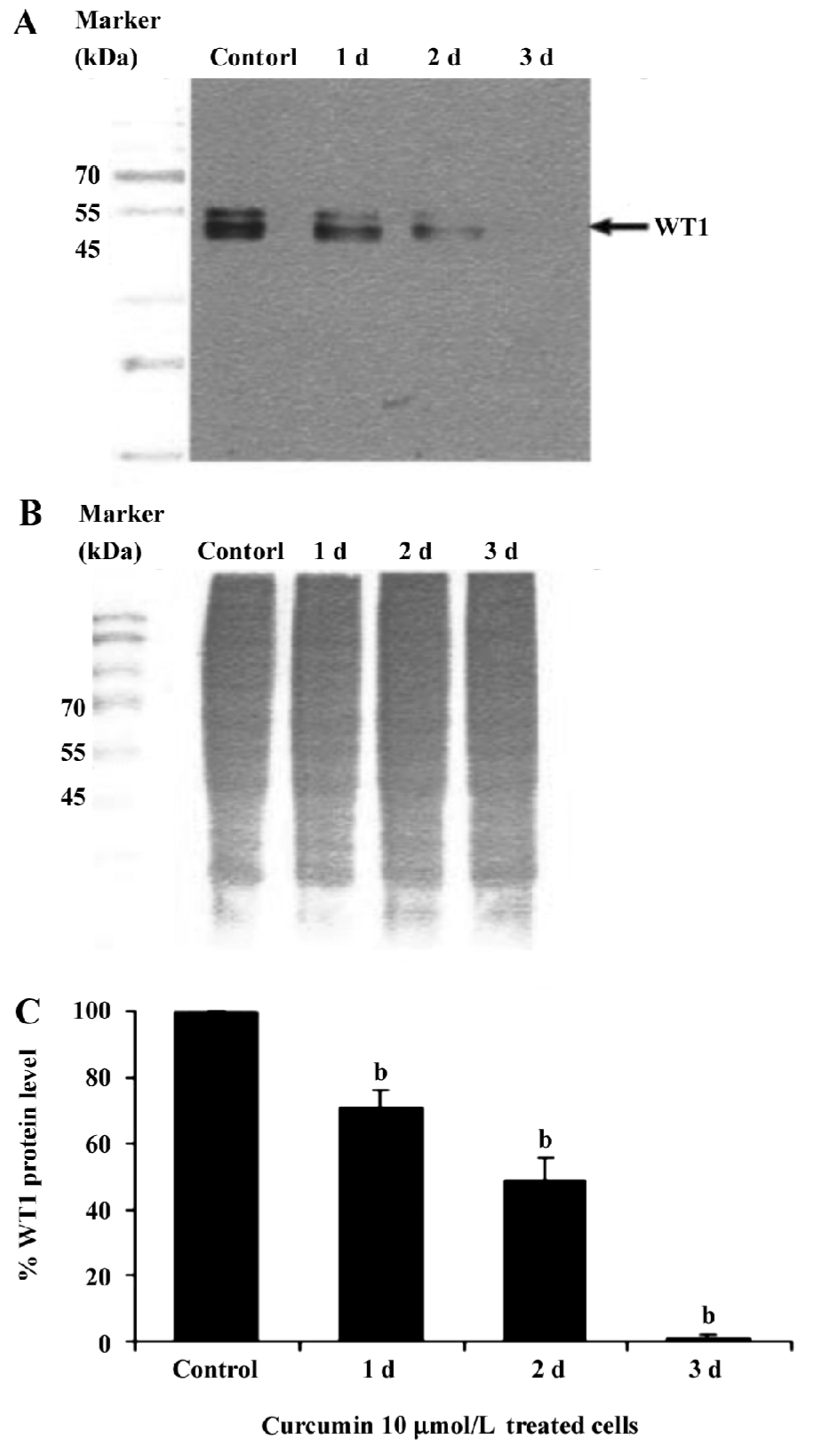
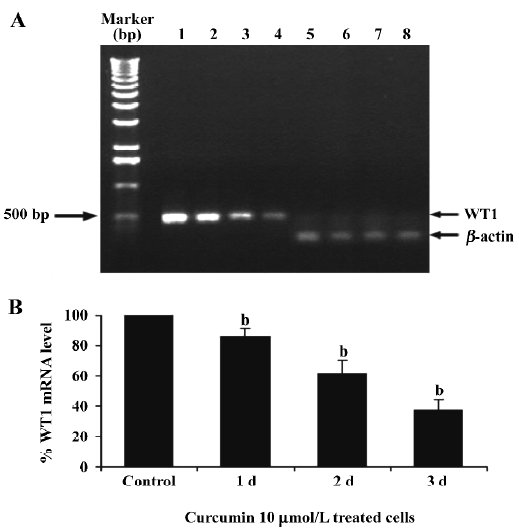
Discussion
In anticancer drugs research, dietary plants, for example, turmeric, chili, ginger, pepper and garlic are of central interest in Thailand. Curcumin, a major active component of the food flavoring turmeric (Curcuma longa Linn) consists of 3 major active ingredients: curcumin, demethoxycurcumin and bisdemethoxycurcumin. Curcumin has numerous biological properties, including antioxidant and anti-inflammatory effects, as well as antimutagen and anticancer properties. Moreover, curcumin also inhibits oncogene expression and protein kinase C activation. The anticancer properties of curcumin have been described by many researchers, including our group. This inhibitory effect by curcumin regulates a wide variety of genes that require AP1 and NFkB activation, which promote cell proliferation and cell differentiation. WT1 protein has been reported to play an important role in early hematopoiesis, and also controls cell differentiation. These transcription factors are regulated by protein kinase C (PKC), which also regulates WT1 protein by phosphorylation at the C-terminal domain[35], and in turn regulates cell proliferation in leukemic cells. The expression of STAT5 mRNA and protein in K562 cells was inhibited by curcumin and curcumin also inhibited K562 cell proliferation[36].
Recently, the WT1 gene, a marker of leukemia, was shown to be overexpressed in leukemic cells, including the K562 and HL60 cell lines[37]. Thus, in the present study, we wanted to demonstrate the possible role of curcumin in the expression of WT 1 protein and WT1 mRNA in K562 human leukemic cells. When 5, 10, or 15 µmol/L curcumin was added to K562 cells for 2 d, there was a decrease in WT1 expression with increasing curcumin concentration, indicating that curcumin reduces the level of immunoreactive WT1 protein observed in K562 cells and also reduces the level of WT1 mRNA under the same conditions. The experimental results shown in Figures 4 and 5 also indicate the time-dependent inhibitory effect of curcumin treatment for 1–3 d on WT1. The mechanistic roles of curcumin in WT1 gene promoter activity and signaling control are under extensive investigation in our laboratory.
In our experiment in which we evaluated the cytotoxicity of curcumin in human K562 leukemic cells (Figure 1), we found an IC50 value of 54 μmol/L. This indicates that curcumin is less toxic in K562 cells than in HL-60 and U937 leukemic cells, which have IC50 of 19 and 24 μmol/L, respectively (data not shown). The result is consistent with our previous observation demonstrating that curcumin affects proliferation to various degrees in different cancer cell lines, including Hep-2 (human larynx cancer), PC-9 and PC-14 (human lung cancer), Hep-1 (mouse hepatoma), F-25 (mutated H-ras transfected NIH mouse fibroblast)[38], and B-NHL cell line Raji cells[39]. Duvoix et al found that curcumin reduces the levels of GSTP1-1 mRNA as well as protein, which is related to its apoptotic effect on the K562 cell line[40].
Taken together, our data indicate that treatment of human K562 leukemic cells with non-cytotoxic concentrations (low doses) of curcumin inhibits WT1 gene expression, whereas curcumin at a high dose induces cell cytotoxicity (IC50=54 µmol/L). Our results suggest that curcumin could potentially be used as a chemotherapeutic agent for human leukemia. This research may lead to clinical trials in the future.
Acknowledgements
We are grateful to Dr Pranee LEECHANACHAI, Suchart KIATWATTANACHARERN, and Tanawan SAMLEERAT for their helpful suggestions. We gratefully acknowledge Dr John MCDERMED for his critical reading of the manuscript.
References
- Fraizer GC, Patmasiriwat P, Zhang XH, Saunders GF. Expression of the tumor suppressor gene WT1 in both human and mouse bone marrow. Blood 1995;86:4704-6.
- Patmasiriwat P, Fraizer GC, Claxton D, Kantarjian H, Saunders GF. Expression of WT1 and GATA-1 in AML with chromosome 16q22 abnormalities. Leukemia 1996;10:1127-33.
- Osborne D, Frost L, Tobal K, Liu Yin JA. Elevated levels of WT1 transcripts in bone marrow harvests are associated with a high relapse risk in patients autografted for acute myeloid leukemia. Bone Marrow Transplant 2005;36:67-70.
- Olszewski M, Muang W, Chou PM, Duerst R, Kletzel M. Wilms’ tumor1 (WT1) gene in hematopoiesis: a surrogate marker of cell proliferation as a possible mechanism of action? Cytotherapy 2005;7:57-61.
- Kelley TW, Huntsman D, McNagny KM, Roskelley CD, Hsi ED. Podocalyxin: a marker of blasts in acute leukemia. Am J Clin Pathol 2005;124:134-42.
- Keilholz U, Menssen HD, Gaiger A, Menke A, Oji Y, Oka Y, et al. Wilms’ tumour 1 (WT1) in human neoplasia. Leukemia 2005;19:1318-23.
- Barragan E, Cervera J, Bolufer P, Ballester S, Martin G, Fernandez P, et al. Prognostic implications of Wilms’ tumor gene (WT1) expression in patients with de novo acute myeloid leukemia. Haematologica 2004;89:926-33.
- Bai B, Wang HW, Xu YQ, Yang HN, Qiao ZH. Fluorescence quantitative PCR detection of WT1 gene expression in peripheral blood of patients with acute leukemias and its clinical implication. Chin J Exp Hematol 2005;13:610-4. Chinese..
- Svensson E, Eriksson H, Gekas C, Olofsson T, Richter J, Gullberg U. DNA-binding dependent and independent functions of WT1 protein during human hematopoiesis. Exp Cell Res 2005;308:211-21.
- Phelan SA, Lindberg C, Call KM. Wilms’ tumor gene, WT1, mRNA is down-regulated during induction of erythroid and megakaryocytic differentiation of K562 cells. Cell growth Differ 1994;5:677-86.
- Sekiya M, Adachi M, Hinoda Y, Imai K, Yachi A. Down-regulation of Wilms’ tumor gene (WT1) during myelomonocytic differentiation in HL60 cells. Blood 1994;83:1876-82.
- Yamagami T, Sugiyama H, Inoue K, Ogawa H, Tatekawa T, Hirata M, et al. Growth inhibition of human leukemic cells by WT1 (Wilms’ tumor gene) antisense oligodeoxynucleotides: implications for the involvement of WT1 in leukemogenesis. Blood 1996;87:2878-84.
- Miwa H, Beran M, Saunders GF. Expression of Wilms’ tumor gene in human leukemias. Leukemia 1992;6:405-9.
- Miyaki T, Ahuji H, Kubota T, Kubonishi I, Koeffler HP, Miyoshi I. Expression of candidate Wilms’ tumor gene, WT1, in human leukemia cells. Leukemia 1993;7:970-7.
- Inoue K, Sugiyama H, Ogawa H, Nakagawa M, Yamagami T, Miwa H, et al. WT1 as a new prognostic factor and a new marker for the detection of minimal residual disease in acute leukemia. Blood 1994;84:3071-9.
- Sugiyama H. Wilms’ tumor gene WT1: oncogenic function and clinical application. Int J Hematol 2001;73:177-87.
- Bergmann L, Miething C, Maurer U, Brieger J, Karakas T, Weidmann E, et al. High level of Wilms’ tumor gene (WT) mRNA in acute myeloid leukemia are associated with a worse long-term outcome. Blood 1997;90:1217-25.
- Govindarajan VS. Turmeric: chemistry, technology and quality. CRC Crit Rev Food Sci Nutr 1990;12:199-301.
- Ammon HPT, Wahl MA. Pharmacology of Curcuma longa. Planta Med 1991;57:1-7.
- Kunchandy E, Rao MNA. Oxygen radical scavenging activity of curcumin. Int J Pharm 1990;58:237-40.
- Soudamini KK, Kuttan R. Inhibition of chemical carcinogenesis by curcumin. J Ethnopharmacol 1989;27:227-33.
- Kuo ML, Huang TS, Lin JK. Curcumin, an antioxidant and antitumor promoter, induces apoptosis in human leukemia cells. Biochim Biophys Acta 1996;1317:95-100.
- Nagabhushan M, Amonkar AJ, Bhide SV. In vitro antimutagenicity of curcumin against environmental mutagens. Food Chem Toxicol 1987;25:545-7.
- Rao CV, Rivenson A, Simi B, Reddy BS. Chemoprevention of colon cancer by dietary curcumin. Ann NY Acad Sci 1995;768:201-4.
- Limtrakul P, Lipigorngoson S, Namwong O, Apisariyakul A, Dunn FW. Inhibitory effect of dietary curcumin on skin carcinogenesis in mice. Cancer Lett 1997;116:197-203.
- Limtrakul P, Anuchapreeda S, Lipigorngoson S, Dunn FW. Inhibition of carcinogen induced c-Ha-ras and c-fos proto-oncogenes expression by dietary curcumin. BMC Cancer 2001;1:1-7.
- Rao DS, Sekhara NC, Satyanarayana MN, Srinivasan M. Effect of curcumin on serum and liver cholesterol levels in the rat. J Nutr 1970;100:1307-15.
- Srinivasan M. Effect of curcumin on blood sugar as seen in a diabetic subject. Indian J Med Sci 1972;26:269-70.
- Anuchapreeda S, Leechanachai P, Smith M, Ambudkar SV, Limtrakul P. Modulation of P-glycoprotein expression and function by curcumin in Multidrug resistant human KB cells. Biochem Pharmacol 2002;64:573-82.
- Qureshi S, Shah AH, Ageel AM. Toxicity studies on Alpinia galanga and Curcuma longa. Planta Medica 1992;58:124-7.
- Shankar TN, Shantha NV, Ramesh HP, Murthy IA, Murthy VS. Toxicity studies on Turmeric: acute toxicity studies in rats, guinea pigs and monkeys. Indian J Exp Biol 1980;18:73-5.
- Alley MC, Scudiero DA, Monks A. Feasibility of drug screening with panels of human tumour cell lines using a microculture tetrazolium assay. Cancer Res 1988;48:589-01.
- Limtrakul P, Chearwae W, Leukumharn S. Inhibitory effect of curcumin on TPA (12-O-tetradecanoyl phorbol-13-acetate)-induced activation of protein kinase C isoenzyme-epsilon and c-fos protein level in human keratinocytes. Science Asia 2005;31:113-20.
- Oji Y, Ogawa H, Tamaki H, Oka Y, Tsuboi A, Kim EH, et al. Expression of Wilms’ tumor gene WT1 in solid tumors and its involvement in tumor cell growth. Jpn J Cancer Res 1999;90:194-204.
- Ye Y, Raychaudhuri B, Gurney A, Campbell CE, Williams BR. Regulation of WT1 by phosphorylation: inhibition of DNA binding, alteration of transcriptional activity and cellular translation. EMBO J 1996;15:5606-15.
- Chen WH, Chen Y, Gu JX, He J. Effect of curcumin on STAT5 signaling molecule in K562 cells. Chin J Hematol 2004;25:151-3.
- Wu YJ, Fraizer DC, Suanders GF. GATA-1 transactivates the WT1 hematopoietic specific enhancer. J Biol Chem 1995;270:5944-9.
- Limtrakul P, Chearwae W, Anuchapreeda S. The effect of curcumin on the proliferation of cancer cell lines. Chiang Mai Med Bull 1999;38:55-61.
- Liu HL, Chen Y, Cui GH, Zhou JF. Curcumin, a potent anti-tumor reagent, is a novel histone deacetylase inhibitor regulating B-NHL cell line Raji proliferation. Acta Pharmacol Sin 2005;26:603-9.
- Dovoix A, Morcean F, Delhalle S, Schmitz M. Inhibition of apoptosis by curcumin mediation by glutathione S-transferase P1-1 inhibition. Biochem Pharmacol 2003;66:1475-83.