Adenovirus-mediated expression of both antisense ODC and AdoMetDC inhibited colorectal cancer cell growth in vitro1
Introduction
Polyamines, which are aliphatic compounds, exist in almost all living species and have important physiological functions in the growth and differentiation of normal cells. In mammary cells, the intracellular polyamine biosynthetic pathway is mainly regulated by the actions of 2 rate-limiting enzymes[1]. The first of these, ornithine decarboxylase (ODC), is required for the first step in polyamine synthesis, in which ornithine is decarboxylated to produce putrescine. The second rate-limiting enzyme is AdoMetDC, which provides the aminopropyl donor dcSAM by decarboxylasting adenosyl-methionine. DcSAM donates its propylamine moiety for the formation of spermidine and spermine by spermidine synthase and spermine synthase, respectively. In addition, exogenous polyamine can also be imported into cells through an as yet unknown transport mechanism[2].
The association between increased polyamine synthesis and cell proliferation and cancer has been known for approximately 40 years. High polyamine levels and elevated polyamine synthetic enzyme activity have been detected in most kinds of cancers[3–5]. In colorectal cancer, the activities of ODC and AdoMetDC and polyamine content are increased 3- to 4-fold over those found in the equivalent normal tissue[6,7]. In addition, our earlier study demonstrated that ODC mRNA and protein levels in colorectal cancer were higher than those in the equivalent normal tissue and, furthermore, they correlated with Dukes stages[8,9]. Therefore, downregulation of ODC and AdoMetDC expression and depletion of polyamine content in colorectal cancer with novel methods such as gene therapy has become a focus of research. Consequently, we constructed a replication-deficient recombinant adenovirus to simultaneously downregulate both ODC and AdoMetDC and detected whether it could inhibit colorectal cancer cell growth in vitro.
Materials and methods
Cells and reagents HT-29 human colon cancer cells and HEK293 packaging cells (transformed human embryonic kidney cells) were obtained from the Chinese Academy of Sciences (Shanghai, China). HT-29 cells were maintained in RPMI-1640 medium supplemented with 10% (v/v) heat-inactivated bovine serum and the antibiotics penicillin G potassium and streptomycin sulfate in a humidified CO2 incubator at 37 °C. HEK293 cells were grown in Dulbecco’s modified Eagle’s medium (Gibco Grand Island, NY, USA) containing 10% fetal bovine serum and penicillin and streptomycin. The shuttle vector pAdTrack and Escherichia coli AdEasy-1 cells (E coli BJ5183 cells transformed with the pAdEasy-1 vector) were provided by Dr Bert Vogelstein (The John Hopkins Oncology Center, Baltimore, MD, USA) The polyamine standards (putrescine, spermidine, spermine), internal standards (1,6-hexanediamine) and dansyl chloride for high performance liquid chromatography (HPLC) were purchased from Sigma (St Louis, MO, USA). Anti-ODC mouse monoclonal antibody and anti-AdoMetDC mouse polyclonal antibody were prepared by our laboratory. Plasmid pTrack-ODCas containing a reverse 120 bp ODC gene was constructed by Dr Yan ZHANG[10].
Amplification of AdoMetDC gene and construction of TA clone Total RNA was extracted from colorectal cancer tissue and then reverse transcribed to first strand cDNA from RNA templates by using a cDNA synthesis kit (TaKaRa, Japan). Synthesized cDNAs were then used as the templates for polymerase chain reaction (PCR) for the amplification of the AdoMetDC gene. The upstream PCR primer was 5'-GG
Construction of plasmid pTrack-ODC-AdoMetDCas Both the recombinant TA vector PMD-AdoMetDC and the shuttle vector pAdTrack-ODCas were digested with XbaI and XhoI. The digested fragments were then separated with electrophoresis and the desired bands of 250 bp and 9.3 kb were cut out from the gel and extracted by using a gel extraction kit. The two purified DNA fragments were ligated with T4 ligase at 16 °C overnight and then transformed into competent DH5α cells. The positive clones (pTrack-ODC-AdoMetDCas) and their insert directions were confirmed by digestion and sequencing. The sequencing primer was 5'-TTC GCT AGT CTC ACG GTG AT-3'.
Construction of pAdEasy-ODC-AdoMetDCas via homologous recombination in bacteria The pTrack-ODC-AdoMetDCas plasmids were first digested with PmeI and then purified. Five micrograms of purified linearized plasmid was transformed into highly competent cells AdEasy-1 prepared using CaCl2 method for homologous recombination with pAdEasy-1. After growth in L-agar plates containing 50 µg/mL kanamycin for 16–24 h, 10 to 20 of the smallest colonies were picked up and grown in 2 mL L-Broth containing 50 µg/mL kanamycin overnight. The plasmid minipreps were prepared using a conventional alkaline lysis method and were digested by PacI or BamHI for identifying candidate clones that usually yield a large fragment (approximately 30 kb), plus a small fragment of 3.0 kb or 4.5 kb. The correct recombinant miniprep DNAs (pAdEasy-ODC-AdoMetDCas) were next re-transformed into the DH5α and purified using Qiagen’s plasmid midi kit.
Viral production in 293 cells Recombinant adenoviral plasmids (pAdEasy-ODC-AdoMetDCas) were digested with PacI, ethanol precipitated and resuspended in sterile H2O. Fifteen micrograms of digested pAdEasy-ODC-AdoMetDCas was transfected into 293 packaging cells by using standard Lipofectamine 2000 (Gibco) transfection according to the manufacturer’s instructions. Two handred and ninety three cells were harvested at 7–10 d post-transfection, then repeatedly frozen at -80 °C and thawed in a 37 °C water bath for total 4 cycles. The samples were spun at 12 000 g for 10 min and the viral supernatant was stored at -80 °C. For further amplification, more 293 cells needed to be infected with these viral stocks. The recombinant virus particles were purified by ultracentrifugation in cesium chloride step gradients. The 2 genes ligated into the virus DNA were also detected using PCR. The titer of purified adenovirus [as measured by green fluorescent protein (GFP) expression] was 2×1010 pfu/mL[11].
Analysis of gene transduction efficiency in vitro The efficiency of the adenovirus-mediated gene transfer was assessed by using GFP. The HT-29 cells were infected with Ad-GFP at multiplicities of infection (MOI) of 5, 10, 20, 50, and 100 for 24 h. The cells were washed with cold phosphate-buffered saline (PBS), and the GFP-positive cells were quantified using fluorescence microscopy.
Measurement of ODC and AdoMetDC expression by Western blot analysis After the HT-29 cells were treated with 50 MOI of PBS, Ad-GFP, and Ad-ODC-AdoMetDCas for 72 h, total cell lysates were prepared in extraction buffer containing 50 mmol/L Tris (pH 8.0), NP-40 (1%), aprotinin (1 µg/mL), sodium dodecylsulfate (0.1%), sodium azide (0.02%), NaCl (150 mmol/L), and phenylmethylsufonyl fluoride (100 µg/mL). Protein from these samples was qualified by using the bicinchoninic acid assay and transferred onto nitrocellulose membranes (Millipore, Bedford, MA, USA). After they were reacted with appropriate antibodies in PBS containing 5% nonfat dry milk and 0.02% Tween-20, blots were incubated with horseradish peroxidase-conjugated secondary antibodies and exposed to X-ray films (Kodak, Shantou, China) using a Western blotting luminol reagent (Santa Cruz Biotechnology, USA).
Measurement of polyamine content Polyamine content was measured according to the method described previous-ly[12]. A total of 1×106 HT-29 cells were treated with PBS, Ad-GFP and Ad-ODC-AdoMetDCas for 3 d and then harvested by scraping. The cell pellets were permeabilized with 5% trichloroacetic acid for 1 h, and the polyamines in the supernatant were separated by centrifugation. The precipitates left were measured for protein content. The polyamines were mixed with a 2-fold volume of dansyl chloride and dansylated in the presence of sodium carbonate for 20 min at 70 °C. Dansylated polyamines were separated and detected using reverse phase HPLC with a C18 column coupled to a scanning fluorescence detector (excitation wavelength 336 nm, emission wavelength 520 nm). 1,6-Hexanediamine used as an internal standard was dansylated and separated in the same way as the polyamines.
Measurement of cell growth Viable cell counts were used to observe the effect of adenovirus on cell proliferation. The HT-29 cells were plated in 6-well tissue culture plates at a density of 5×104 cells/well. After 24 h, tumor cells were treated with 50 MOI Ad-GFP, Ad-ODC-AdoMetDCas or PBS as a mock control. Cells in each treatment group were plated in triplicate and cultured for 6 d. Then, every 24 h cells were harvested by trypsinization and stained with 0.4% trypan blue (Gibco, USA) to reveal the dead cells. Viable cells were then counted with hemocytometer.
Statistical analysis Data in this paper are presented as the mean±SD from 3 separate experiments. Student’s t-test was used to compare the data, and P<0.05 was taken as the level of significance. All results were analyzed by using the SPSS (version 10.0) statistical software package.
Results
Identification of AdoMetDC gene and TA clone The AdoMetDC gene was amplified by reverse transcription PCR and then ligated into the TA clone vector PMD-18T. The PCR products were separated by agarose-gel electrophoresis, and an approximately 205 bp band was obtained, which is consistent with the size of the AdoMetDC gene (gi: 5209326; Figure 1). A fragment the same size as the AdoMetDC gene was also produced after the digestion of the constructed TA vector (Figure 1), which suggests that the PCR product was successfully inserted into PMD-18T.
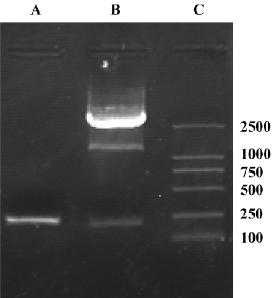
Identification of recombinant shuttle vector pAdTrack-ODC-AdoMetDCas To identify whether AdoMetDC had been inserted into the constructed shuttle vector, it was digested with XbaI and XhoI, and 2 fragments (205 bp and 9.3 kb) were found in 0.8% agarose gel electrophoresis (Figure 2). To identify whether ODC had been inserted, the vector was digested with BglII and SalI, and 2 fragments (120 bp and 9.3 bp) were obtained (Figure 2). Furthermore, to identify whether AdoMetDC plus ODC had been inserted, the vector was digested with BglII and SalI, and 2 fragments (340 bp and 9.3 kb; Figure 2) were produced. The restriction fragments generated in each case were consistent with the sizes of the inserted genes (ODC and AdoMetDC) and the pAdTrack vector. These results suggest that the candidate shuttle vector contained both ODC and AdoMetDC genes. The sequencing results further confirmed the insertion of the genes.
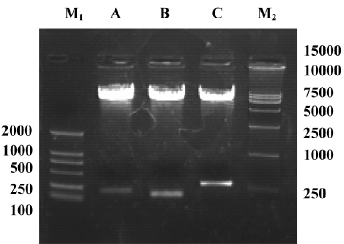
Identification of adenoviral backbone DNA pAdEasy-ODC-AdoMetDCas The recombinant shuttle vector was transformed into E coli AdEasy-1 cells for homologous recombination with the pAdEasy-1 vector. Candidate clones were digested with BamHI or PacI to verify proper recombination. The expected restriction fragments were generated in each case. With BamHI, a 7 kb fragment was produced in addition to the 11.7 and 21.7 kb fragments generated from pAdEasy-1 sequences (Figure 3). With PacI digestion, two fragments (4.5 kb and 35 kb) were produced (Figure 3).
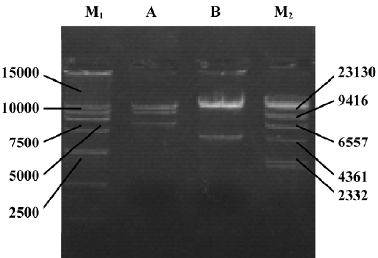
Production of adenovirus in 293 cells To produce the viruses, 15 µg of pAdEasy-ODC-AdoMetDCas was digested with PacI to liberate the linear adenoviral genomes, and the resulting fragments were then transfected into 293 cells. GFP expression was used to monitor the viral production process. As shown in Figure 4A, GFP expression was visible 24 h after transfection, which indicated that the virus particles had been packaged. For amplification, the generated viral particles were used to infect more 293 cells, and stronger GFP expression was found (Figure 4B).
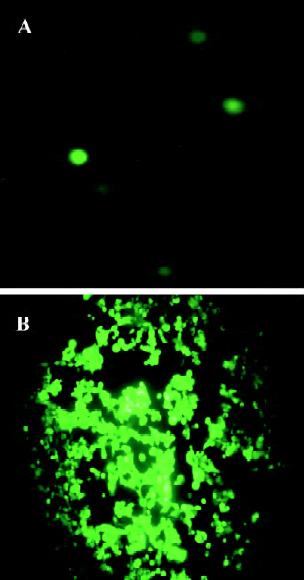
Gene transduction efficiency in vitro The Ad-GFP vector was used to estimate the gene transduction efficiency in HT-29 cells. We found that 69±5.1% of HT-29 cells were GFP-positive with an MOI of 50, and there was no obvious toxicity. Therefore, this MOI was adopted for further studies.
Inhibitory effect of Ad-ODC-AdoMetDCas on ODC and AdoMetDC gene expression in HT-29 cells Western blot analysis was performed to detect the effect of replication-deficient Ad-ODC-AdoMetDCas infection on intracellular ODC and AdoMetDC protein levels. Both ODC and AdoMetDC protein levels were significantly decreased in Ad-ODC-AdoMetDCas-treated HT-29 cells when compared with Ad-GFP or PBS-treated cells (Figure 5).
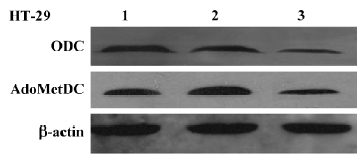
Inhibitory effect of Ad-ODC-AdoMetDCas on polyamine content in HT-29 cells After demonstrating that Ad-ODC-AdoMetDCas depressed ODC and AdoMetDC protein expression in HT-29 cells, we next evaluated whether the polyamine concentration could be decreased accordingly by adenoviral gene transfer into tumor cells. Polyamines in adenovirus-infected or -uninfected colorectal cancer cells were separated by ion-pairing reverse phase HPLC. Incubation with Ad-ODC-AdoMetDCas resulted in depletion of all three polyamines to a very low level in HT-29 cells when compared with cells incubated with Ad-GFP or PBS (Table 1).
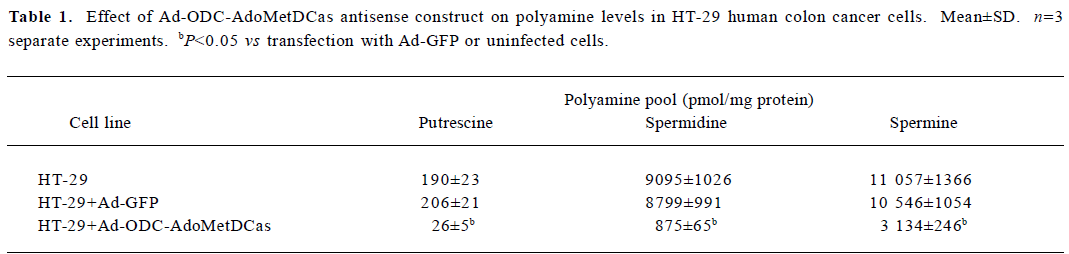
Full table
Inhibitory effect of Ad-ODC-AdoMetDCas on HT-29 cell growth Analysis of cell viability revealed significant inhibition of cell proliferation in colorectal cancer cells treated with Ad-ODC-AdoMetDCas (P<0.05) when compared with control cells treated with Ad-GFP or PBS (Figure 6). This cell growth retardation remains for 7 d (data not shown).
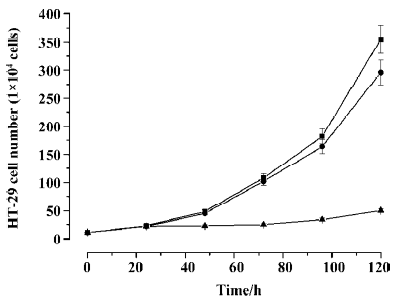
Significant morphological changes were also observed 3 d after incubation with recombinant adenovirus. HT-29 cells infected with Ad-ODC-AdoMetDCas had many vacuoles and particles in the cytoplasm and lost cell-to-cell contacts. In addition, partial cells rounded up and detached from the plate, which indicated cell death (Figure 7A). In contrast, the Ad-GFP-infected cells (Figure 7B) didn’t have any obvious morphological changes relative to the uninfected cells (Figure 7C).
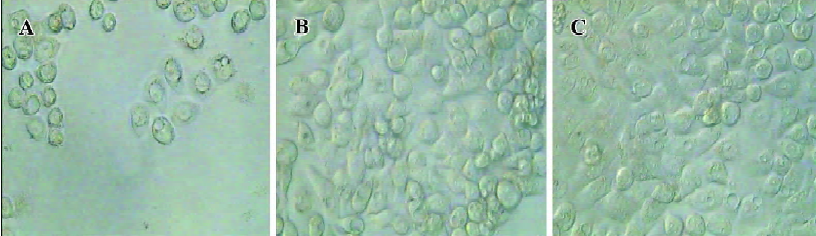
To further examine whether the antiproliferative effect of Ad-ODC-AdoMetDCas can be antagonized by exogenous polyamines, putrescine and spermidine were added to restore HT-29 cell proliferation. The growth arrest caused by Ad-ODC-AdoMetDCas was not reversed by exogenous putrescine, though which was partially reversed by spermidine (Table 2).
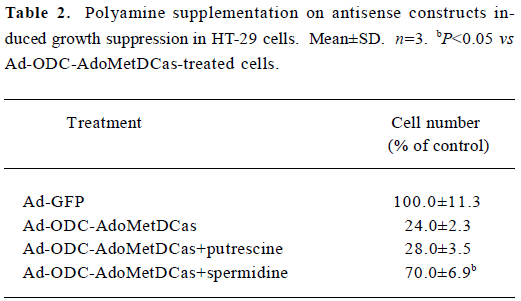
Full table
Discussion
There are many biochemical alterations in colorectal cancer cells, but one of the most consistent changes is the elevation of intracellular polyamine content[13]. Increases in ODC and polyamine content appear in both early and late stages during the adenoma-carcinoma sequence[14]. Aberrations of tumor tissue metabolism render polyamine biosynthesis an attractive target for anticancer drug therapy[15,16]. Blockade of ODC and AdoMetDC enzyme activity by polyamine synthesis inhibitors such as ODC suicide inhibitor alpha-difluoromethylornithine (DFMO) and AdoMetDC inhibitors MGBG and SAM486A was found to be effective in arresting tumor cell growth in a preclinical study[17]. However, due to their low efficiency, general toxicity and side-effects, convincing antitumor effects were not found in subsequent clinical studies[18–20]. Hence, the development of novel strategies for the blockade of polyamine synthesis such as gene therapy are necessary.
There are a number of viral and non-viral delivery routes and methods for gene transfer used in gene therapy[21–24]. Among them, adenovirus-mediated gene transfer deserves particular attention as a means to deliver genes for cancer gene therapy[25]. Adenoviruses are attractive vectors for cancer therapy because of their unparalleled capacity for gene transfer and stability in vivo. Adenoviruses have the capacity to transfer genes to a broad spectrum of cell lines, and is not dependent on active cell division. Additionally, high titers (>1010 pfu/mL) can be generated, unlike retroviral vectors, which are produced at relatively low titers (105 to 106 pfu/mL), thus making high MOI easy to achieve. Furthermore, adenovirus DNA is not integrated into the host genome, and the risk of mutagenesis is low. Adenovirus cannot infect oocytes, therefore no female germline transduction has been noted. To date, adenoviruses have been used in approximately 27% of the more than 600 gene therapy clinical protocols reported[26]. Consequently, given this background, we constructed adenovirus vectors to transfer antisense ODC and AdoMetDC genes to colorectal cancer.
In the present study we successfully constructed a replication-deficient recombinant adenovirus that can simultaneously express antisense ODC and AdoMetDC genes. Western blot analysis demonstrated that Ad-ODC-AdoMetDCas infection significantly reduced both ODC and AdoMetDC protein levels in HT-29 cells. Furthermore, with substantial decreases in ODC and AdoMetDC expression, all 3 intracellular polyamines were depleted to a low level after Ad-ODC-AdoMetDCas infection.
In addition, our data showed that Ad-ODC-AdoMetDCas infection had a significant effect in suppressing colorectal cancer cell growth relative to vector infection. Polyamines are known to play a key role in maintaining a high cell proliferation rate. A reduction in polyamines may contribute to the suppression of cancer growth. Previous studies have shown that DFMO induced growth arrest across a range of cancer cells, including bladder[27], breast[28], intestinal[29], skin[30] and colon cancer[31]. However, it is known that cell growth inhibition due to single blockade of ODC (eg by DFMO) can be rescued by exogenous putrescine[32]. The reason for this is that environmental putrescine that is actively taken up by epithelial cells[33] compensates for impaired putrescine synthesis, and then via AdoMetDC, is rapidly converted to metabolically active spermidine and spermine. In contrast, cell growth arrest induced by Ad-ODC-AdoMetDCas was not rescued by exogenous putrescine. The reason may be the simultaneous inhibition of AdoMetDC by Ad-ODC-AdoMetDCas, which would impair intracellular spermine biosynthesis and subsequently block DNA synthesis[34]. Therefore, Ad-ODC-AdoMetDCas would be particularly useful in the treatment of colorectal cancers, which are reportedly “bathed” in large amounts of luminal putrescine[33].
In summary, our data provide evidence that adenovirus-mediated expression of both antisense ODC and AdoMetDC depletes the polyamine pool and leads to significant suppression of colorectal cancer cell growth. Synergistic inhibition of ODC and AdoMetDC by using a gene therapy approach might therefore represent a novel treatment option for colorectal cancer.
Acknowledgement
We would like to thank Dr Bert VOGELSTEIN for providing the pAdEasy-1 adenovirus system.
References
- Morgan DM. Polyamines. An overview. Mol Biotechnol 1999;11:229-50.
- Dandrifosse G, Deloyer P, Grandfils C, Loret S, Peulen O, Dandrifosse AC. Statement on polyamines. Rev Med Liege 1999;54:175-83.
- Glikman P, Vegh I, Pollina MA, Mosto AH, Levy CM. Ornithine decarboxylase activity, prolactin blood levels, and estradiol and progesterone receptors in human breast cancer. Cancer 1987;60:2237-43.
- Upp JR Jr, Saydjari R, Townsend CM Jr, Singh P, Barranco SC, Thompson JC. Polyamine levels and gastrin receptors in colon cancers. Ann Surg 1988;207:662-9.
- Gerner EW, Meyskens FL Jr. Polyamines and cancer: old molecules, new understanding. Nat Rev Cancer. 2004;4:781-92.
- Loser C, Folsch UR, Paprotny C, Creutzfeldt W. Polyamines in colorectal cancer. Evaluation of polyamine concentrations in the colon tissue, serum and urine of 50 patients with colorectal cancer. Cancer. 1990;65:958-66.
- Higuchi CM, Wang W. Comodulation of cellular polyamines and proliferation: biomarker application to colorectal mucosa. J Cell Biochem 1995;57:256-61.
- Sun AH, Liu XX, Zhang B. Expression and clinical implication of ODC mRNA in colorectal carcinoma. Chin J Curr Adv Gen Surg 2004;7:150-2.
- Hu HY, Liu XX, Jiang CY, Lu Y, Zhang Y, Zhang B, et al. Ornithine decarboxylase gene is overexpressed in colorectal carcinoma. World J Gastroenterol 2005;11:2244-8.
- Zhang Y, Liu XX, Zhang B, Hu HY, Gong L. Antitumor effect of antisense ODC adenovirus on human prostate cancer cells. Prostate Cancer Prostatic Dis 2005;8:280-6.
- Zhang B, Liu XX, Jiang CY, Zhang Y, Hu HY, Geng Z. Construction of an antisense bicistronic recombinant adenovirus vector of ornithine decarboxylase and S-adenosylmethionine decarbo-xylase. J Shandong Univ 2005;43:387-90. (Health Sci).
- Aboul-Enein HY, al-Duraibi IA. Separation of several free polyamines and their acetylated derivatives by ion-pair reversed-phase high performance liquid chromatography. Biomed Chromatogr 1998;12:291-3.
- Wallace HM, Caslake R. Polyamines and colon cancer. Eur J Gastroenterol Hepatol 2001;13:1033-9.
- Kinzler KW, Vogelstein B. Lessons from hereditary colorectal cancer. Cell 1996;87:159-70.
- Wang JF, Su RB, Wu N, Xu B, Lu XQ, Liu Y. Inhibitory effect of agmatine on proliferation of tumor cells by modulation of polyamine metabolism. Acta Pharmacol Sin 2005;26:616-22.
- Janne J. AIhonen L, Leinonen P. Polyamines: from molecular biology to clinical applications. Ann Med 1991;23:241-59.
- Seiler N. Thirty years of polyamine-related approaches to cancer therapy. Retrospect and prospect. Part 2. Structural analogues and derivatives. Curr Drug Targets 2003;4:565-85.
- Meyskens FL, Gerner EW. Development of difluoromethyl-ornithine (DFMO) as a chemoprevention agent. Clin Cancer Res 1999;5:945-51.
- Loesberg C, Van Rooji H, Romijn JC, Smets LA. Mitochondrial effects of the guanidino group-containing cytostatic drugs, m-iodobenzylguanidine and methylglyoxal bis (guanylhydrazone). Biochem Pharmacol 1991;42:793-8.
- Dorhout B, Odink MF, de Hoog EM, Kingma AW, van der Veer E, Muskiet FA. 4'-Aminoindan-1-one 2'-amidinohydrazone (CGP48664A) exerts in vitro growth inhibitory effects that are not only related to S-adenosylmethionine decarboxylase inhibi-tion. Biochim Biophys Acta 1997;1335:144-52.
- Yi C, Huang Y, Guo ZY, Wang SR. Antitumor effect of cytosine deaminase/5-fluorocytosine suicide gene therapy system mediated by Bifidobacterium infantis on melanoma. Acta Pharmacol Sin 2005;26:629-34.
- Zhang SN, Yuan SZ, Zhu ZH, Wen ZF, Huang ZQ, Zeng ZY. Apoptosis induced by 5-flucytosine in human pancreatic cancer cells genetically modified to express cytosine deaminase. Acta Pharmacol Sin 2000;21:655-9.
- Hu YC. Baculovirus as a highly efficient expression vector in insect and mammalian cells. Acta Pharmacol Sin 2005;26:405-16.
- Li L, He DL. Transfection of promyelocytic leukemia in retrovirus vector inhibits growth of human bladder cancer cells. Acta Pharmacol Sin 2005;26:610-5.
- Kanerva A, Hemminki A. Modified adenoviruses for cancer gene therapy. Int J Cancer 2004;110:475-80.
- St George JA. Gene therapy progress and prospects: adenoviral vectors. Gene Ther 2003;10:1135-41.
- Nowels K, Homma Y, Seidenfeld J, Oyasu R. Prevention of inhibitory effects of alpha-difluoromethylornithine on rat urinary bladder carcinogenesis by exogenous putrescine. Cancer Biochem Biophys 1986;8:257-63.
- Thompson HJ, Ronan AM. Effect of D,L-2-difluoromethylorni-thine and endocrine manipulation on the induction of mammary carcinogenesis by 1-methyl-1-nitrosourea. Carcinogenesis 1986;7:2003-6.
- Nigro ND, Bull AW, Boyd ME. Inhibition of intestinal carcinogenesis in rats: effect of difluoromethylornithine with piroxicam or fish oil. J Natl Cancer Inst 1986;77:1309-13.
- Arbeit JM, Riley RR, Huey B, Porter C, Kelloff G, Lubet R, et al. Difluoromethylornithine chemoprevention of epidermal carcinogenesis in K14- HPV16 transgenic mice. Cancer Res 1999;59:3610-20.
- Marton LJ, Pegg AE. Polyamines as targets for therapeutic intervention. Annu Rev Pharmacol Toxicol 1995;35:55-91.
- Gamet L, Cazenave Y, Trocheris V, Denis-Pouxviel C, Murat JC. Involvement of ornithine decarboxylase in the control of proliferation of the HT29 human colon cancer cell line. Effect of vasoactive intestinal peptide on enzyme activity. Int J Cancer 1991;47:633-8.
- McCormack SA, Johnson LR. Putrescine uptake and release by colon cancer cells. Am J Physiol 1989;256:G868-77.
- Desiderio MA, Bergamaschi D, Mascellani E, De Feudis P, Erba E, D’Incalci M. Treatment with inhibitors of polyamine biosynthesis, which selectively lower intracellular spermine, does not affect the activity of alkylating agents but antagonizes the cytotoxicity of DNA topoisomerase II inhibitors. Br J Cancer 1997;75:1028-34.